Carbohydrate synthesis
![]() | This article may be too technical for most readers to understand.(February 2016) |
Carbohydrate synthesis is a sub-field of organic chemistry concerned with generating complex carbohydrate structures from simple units (monosaccharides) through natural or unnatural processes. The generation of carbohydrate structures involves linking glycosyl groups like monosaccharides or oligosaccharides through glycosidic bonds is called glycosylation. Carbohydrate synthesis aims to generate the polysaccharides with controlled structures through atomically economic methods. Therefore, it is important to construct glycosidic linkages that have optimum molecular geometry (stereoselectivity) and the stable bond (regioselectivity) at the reaction site (anomeric centre).[1]

Background
[edit]Generally speaking, carbohydrates can be classified into two groups, simple sugars, and complex carbohydrates. Simple sugars, also called monosaccharides, are carbohydrates that cannot be further broke down by hydrolysis. When glycosidic linkages connect two or more monosaccharide units, complex carbohydrates are formed. Complex carbohydrates, according to the different number of monosaccharide units, can be classed into three groups, disaccharides, oligosaccharides, and polysaccharides. A disaccharide is formed from two monosaccharides. When a carbohydrate contains 3-10 monosaccharides,[2] it is called a oligosaccharide. Higher oligosaccharides with more than 10 monosaccharides are polysaccharides.
So far, there has not been a unified synthetic strategy of consistent oligosaccharide production because of the nuances in the anomeric effects of monomers and the complexity in the carbohydrate structures.[3][4] The facile procedures such as the one-pot and solid phase synthesis which ensures atom economy[5][3] are used. However, further developments in those synthetic approaches are needed since still not fully controlled and automated.[5]
Significance
[edit]Glycoconjugate is the covalently bonded product of oligosaccharides to the biomolecules such as proteins and lipids.[6] They play indispensable role in the biological activities of mammalian cells from energy generation to cell signalling.[6][7][8] These glycoconjugates with short oligosaccharide structures are important for the characterization and purification in the course glycoconjucate vaccine developments.[9] Therefore, research in the engineering of the glycosyl precursors that create oligosaccharides with controlled size is important in carbohydrate synthesis.[citation needed]
Oligosaccharide synthesis
[edit]Oligosaccharides have diverse structures. The number of monosaccharides, ring size, the different anomeric stereochemistry, and the existence of the branched-chain sugars all contribute to the amazing complexity of the oligosaccharide structures. The essence of the reducing oligosaccharide synthesis is connecting the anomeric hydroxyl of the glycosyl donors to the alcoholic hydroxyl groups of the glycosyl acceptors. Protection of the hydroxyl groups of the acceptor with the target alcoholic hydroxyl group unprotected can assure regiochemical control. Additionally, factors such as the different protecting groups, the solvent, and the glycosylation methods can influence the anomeric configurations. This concept is illustrated by an oligosaccharide synthesis in Scheme 1. Oligosaccharide synthesis normally consists of four parts: preparation of the glycosyl donors, preparation of the glycosyl acceptors with a single unprotected hydroxyl group, the coupling of them, and the deprotection process.[citation needed]

Building blocks
[edit]Common donors in oligosaccharide synthesis are glycosyl halides, glycosyl acetates, thioglycosides, trichloroacetimidates, pentenyl glycosides, and glycals. Of all these donors, glycosyl halides are classic donors, which played a historical role in the development of glycosylation reactions. Thioglycoside and trichloroacetimidate donors are used more than others in contemporary glycosylation methods. When it comes to the trichloroacetimidate method, one of the advantages is that there is no need to introduce heavy metal reagents in the activation process. Moreover, using different bases can selectively lead to different anomeric configurations. (Scheme 2) As to the thioglycosides, the greatest strength is that they can offer temporary protection to the anomeric centre because they can survive after most of the activation processes.[10] Additionally, a variety of activation methods can be employed, such as NIS/ AgOTf, NIS/ TfOH, IDCP (Iodine Dicollidine Perchlorate), iodine, and Ph2SO/ Tf2O. Furthermore, in the preparation of 1, 2-trans glycosidic linkage, using thioglycosides and imidates can promote the rearrangement of the orthoester byproducts, since the reaction mixtures are acidic enough.

Stereoselectivity
[edit]The structures of acceptors play a critical role in the rate and stereoselectivity of glycosylations. Generally, the unprotected hydroxyl groups are less reactive when they are between bulky protecting groups. That is the reason why the hydroxyl group at OH-4 in pyranosides is unreactive. Hyperconjugation is involved when OH-4 is anti-periplanar to the ring oxygen, which can also reduce its reactivity. (Scheme 3) Furthermore, acyl protecting groups can reduce the reactivity of the acceptors compared with alkyl protecting groups because of their electron-withdrawing ability. Hydroxyl group at OH-4 of N-acetylglucosamine derivatives is particularly unreactive.[11]
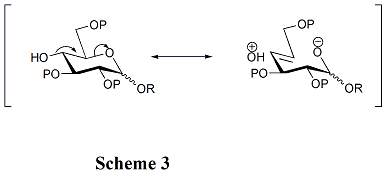
The glycosidic bond is formed from a glycosyl donor and a glycosyl acceptor. There are four types of glycosidic linkages: 1, 2-trans-α, 1, 2-trans-beta, 1, 2-cis-α, and 1, 2-cis-beta linkages. 1, 2-trans glycosidic linkages can be easily achieved by using 2-O-acylated glycosyl donors (neighboring group participation). To prevent the accumulation of the orthoester intermediates, the glycosylation condition should be slightly acidic.
Challenging linkages
[edit]When connecting the monosaccharides, the oligosaccharides need to be reducing in order to sequentially connect the glycosyl units. The monosaccharides, in nature prefer ɑ-linkages due to anomeric effect,[1] but the disaccharides with ɑ-linkages are non-reducing thus deactivating the consequent connection of the monosaccharides.[12] In order to make the process of glycosylation continuous and automated, the glycosidic linkages must maintain beta so to keep the structure open to coupling with more glycosyl groups.
It is somewhat more difficult to prepare 1, 2-cis-β-glycosidic linkages stereoselectively. Typically, when non-participating groups on O-2 position, 1, 2-cis-β-linkage can be achieved either by using the historically important halide ion methods, or by using 2-O-alkylated glycosyl donors, commonly thioglycosides or trichloroacetimidates, in nonpolar solvents.[citation needed]
In the early 1990s, it was still the case that the beta mannoside linkage was too challenging to be attempted by amateurs. However, the method introduced by Crich (Scheme 4), with 4,6-benzylidene protection a prerequisite and anomeric alpha triflate a key intermediate leaves this problem essentially solved. The concurrently developed but rather more protracted intramolecular aglycon delivery (IAD) approach[13] is a little-used but nevertheless stereospecific alternative.

See also
[edit]References
[edit]- ^ a b Tiwari, Vinod Kumar (October 26, 2023). Synthetic Strategies in Carbohydrate Chemistry (1st ed.). Elsevier. pp. 3–7. ISBN 9780323986496.
- ^ Laurentin, A.; Edwards, C. A. (2013-01-01), "Fiber: Resistant Starch and Oligosaccharides", in Caballero, Benjamin (ed.), Encyclopedia of Human Nutrition (Third Edition), Waltham: Academic Press, pp. 246–253, ISBN 978-0-12-384885-7, retrieved 2024-04-13
- ^ a b Seeberger, Peter H.; Werz, Daniel B. (April 2007). "Synthesis and medical applications of oligosaccharides". Nature. 446 (7139): 1046–1051. Bibcode:2007Natur.446.1046S. doi:10.1038/nature05819. ISSN 1476-4687. PMID 17460666.
- ^ Boltje, Thomas J.; Buskas, Therese; Boons, Geert-Jan (2009-11-01). "Opportunities and challenges in synthetic oligosaccharide and glycoconjugate research". Nature Chemistry. 1 (8): 611–622. Bibcode:2009NatCh...1..611B. doi:10.1038/nchem.399. ISSN 1755-4330. PMC 2794050. PMID 20161474.
- ^ a b Xu, Han; Shen, Baoxing; Qiao, Meng; Linhardt, Robert J.; Zhang, Xing (2021-04-15). "Recent advances on the one-pot synthesis to assemble size-controlled glycans and glycoconjugates and polysaccharides". Carbohydrate Polymers. 258: 117672. doi:10.1016/j.carbpol.2021.117672. ISSN 1879-1344. PMID 33593549.
- ^ a b Shivatare, Sachin S.; Shivatare, Vidya S.; Wong, Chi-Huey (2022-10-26). "Glycoconjugates: Synthesis, Functional Studies, and Therapeutic Developments". Chemical Reviews. 122 (20): 15603–15671. doi:10.1021/acs.chemrev.1c01032. ISSN 0009-2665. PMC 9674437. PMID 36174107.
- ^ Chandel, Navdeep S. (January 2021). "Carbohydrate Metabolism". Cold Spring Harbor Perspectives in Biology. 13 (1): a040568. doi:10.1101/cshperspect.a040568. ISSN 1943-0264. PMC 7778149. PMID 33397651.
- ^ Daniel E. Levy & Péter Fügedi.; The organic chemistry of sugars; Taylor & Francis: 2006, pp 181-197
- ^ Stefanetti, Giuseppe; MacLennan, Calman Alexander; Micoli, Francesca (2022-09-29). "Impact and Control of Sugar Size in Glycoconjugate Vaccines". Molecules. 27 (19): 6432. doi:10.3390/molecules27196432. ISSN 1420-3049. PMC 9572008. PMID 36234967.
- ^ Robert V. Stick.; Carbohydrates: The Sweet Molecules of Life.; Academic Press.; 2001, pp 113-177
- ^ Crich, D.; Dudkin V. J. Am. Chem. Soc. 2001, 123, 6819-6825
- ^ "Influence of glycosidic linkage on the nature of carbohydrate binding in β-prism I fold lectins: An X-ray and molecular dynamics investigation on banana lectin–carbohydrate complexes". Glycobiology. 21 (1): 23–33. January 2011. doi:10.1093/glycob/cwq128.
- ^ Garegg, P. J. Chemtracts-Org. Chem., 1992, 5, 389
External links
[edit]Media related to Carbohydrate synthesis at Wikimedia Commons