Voyager program

The Voyager program is an American scientific program that employs two interstellar probes, Voyager 1 and Voyager 2. They were launched in 1977 to take advantage of a favorable alignment of the two gas giants Jupiter and Saturn and the ice giants, Uranus and Neptune, to fly near them while collecting data for transmission back to Earth. After launch, the decision was made to send Voyager 2 near Uranus and Neptune to collect data for transmission back to Earth.[1]
On 25 August 2012, data from Voyager 1 indicated that it had entered interstellar space.[2]On 5 November 2019, data from Voyager 2 indicated that it also had entered interstellar space.[3] On 4 November 2019, scientists reported that, on 5 November 2018, the Voyager 2 probe had officially reached the interstellar medium (ISM), a region of outer space beyond the influence of the solar wind, as did Voyager 1 in 2012.[4][5][6] In August 2018, NASA confirmed, based on results by the New Horizons spacecraft, the existence of a "hydrogen wall" at the outer edges of the Solar System that was first detected in 1992 by the two Voyager spacecraft.[7][8][9]
As of 2024,[update] the Voyagers are still in operation beyond the outer boundary of the heliosphere in interstellar space. Voyager 1 is moving with a velocity of 61,198 kilometers per hour (38,027 mph), or 17 km/s, relative to the Sun, and is 24,475,900,000 kilometers (1.52086×1010 mi) from the Sun[10] reaching a distance of 162 AU (24.2 billion km; 15.1 billion mi) from Earth as of May 25, 2024.[11] As of 2024[update], Voyager 2 is moving with a velocity of 55,347 kilometers per hour (34,391 mph), or 15 km/s, relative to the Sun, and is 20,439,100,000 kilometers (1.27003×1010 mi) from the Sun[12] reaching a distance of 136.627 AU (20.4 billion km; 12.7 billion mi) from Earth as of May 25, 2024.[11]
History
[edit]Mariner Jupiter-Saturn
[edit]

Voyager did things no one predicted, found scenes no one expected, and promises to outlive its inventors. Like a great painting or an abiding institution, it has acquired an existence of its own, a destiny beyond the grasp of its handlers.
The two Voyager space probes were originally conceived as part of the Planetary Grand Tour planned during the late 1960s and early 70s that aimed to explore Jupiter, Saturn, Saturn's moon, Titan, Uranus, Neptune, and Pluto. The mission originated from the Grand Tour program, conceptualized by Gary Flandro, an aerospace engineer at the Jet Propulsion Laboratory, in 1964, which leveraged a rare planetary alignment occurring once every 175 years.[14][15] This alignment allowed a craft to reach all outer planets using gravitational assists. The mission was to send several pairs of probes and gained momentum in 1966 when it was endorsed by NASA's Jet Propulsion Laboratory. However, in December 1971, the Grand Tour mission was canceled when funding was redirected to the Space Shuttle program.[16]
In 1972, a scaled-down (four planets, two identical spacecraft) mission was proposed, utilizing a spacecraft derived from the Mariner series, initially intended to be Mariner 11 and Mariner 12. The gravity-assist technique, successfully demonstrated by Mariner 10, would be used to achieve significant velocity changes by maneuvering through an intermediate planet's gravitational field to minimize time towards Saturn.[17] The spacecrafts were then moved into a separate program named Mariner Jupiter-Saturn (also Mariner Jupiter-Saturn-Uranus,[18] MJS, or MJSU), part of the Mariner program, later renamed because it was thought that the design of the two space probes had progressed sufficiently beyond that of the Mariner family to merit a separate name.[19]
Voyager probes
[edit]
On March 4, 1977, NASA announced a competition to rename the mission, believing the existing name was not appropriate as the mission had differed significantly from previous Mariner missions. Voyager was chosen as the new name, referencing an earlier suggestion by William Pickering, who had proposed the name Navigator. Due to the name change occurring close to launch, the probes were still occasionally referred to as Mariner 11 and Mariner 12, or Voyager 11 and Voyager 12.[16]
Two mission trajectories were established: JST aimed at Jupiter, Saturn, and enhancing a Titan flyby, while JSX served as a contingency plan. JST focused on a Titan flyby, while JSX provided a flexible mission plan. If JST succeeded, JSX could proceed with the Grand Tour, but in case of failure, JSX could be redirected for a separate Titan flyby, forfeiting the Grand Tour opportunity.[17] The second probe, now Voyager 2, followed the JSX trajectory, granting it the option to continue on to Uranus and Neptune. Upon Voyager 1 completing its main objectives at Saturn, Voyager 2 received a mission extension, enabling it to proceed to Uranus and Neptune. This allowed Voyager 2 to diverge from the originally planned JST trajectory.[16]
The probes would be launched in August or September 1977, with their main objective being to compare the characteristics of Jupiter and Saturn, such as their atmospheres, magnetic fields, particle environments, ring systems, and moons. They would fly by planets and moons in either a JST or JSX trajectory. After completing their flybys, the probes would communicate with Earth, relaying vital data using their magnetometers, spectrometers, and other instruments to detect interstellar, solar, and cosmic radiation. Their radioisotope thermoelectric generators (RTGs) would limit the maximum communication time with the probes to roughly a decade. Following their primary missions, the probes would continue to drift into interstellar space.[17]
Voyager 2 was the first to be launched. Its trajectory was designed to allow flybys of Jupiter, Saturn, Uranus, and Neptune. Voyager 1 was launched after Voyager 2, but along a shorter and faster trajectory that was designed to provide an optimal flyby of Saturn's moon Titan,[20] which was known to be quite large and to possess a dense atmosphere. This encounter sent Voyager 1 out of the plane of the ecliptic, ending its planetary science mission.[21] Had Voyager 1 been unable to perform the Titan flyby, the trajectory of Voyager 2 could have been altered to explore Titan, forgoing any visit to Uranus and Neptune.[22] Voyager 1 was not launched on a trajectory that would have allowed it to continue to Uranus and Neptune, but could have continued from Saturn to Pluto without exploring Titan.[23]
During the 1990s, Voyager 1 overtook the slower deep-space probes Pioneer 10 and Pioneer 11 to become the most distant human-made object from Earth, a record that it will keep for the foreseeable future. The New Horizons probe, which had a higher launch velocity than Voyager 1, is travelling more slowly due to the extra speed Voyager 1 gained from its flybys of Jupiter and Saturn. Voyager 1 and Pioneer 10 are the most widely separated human-made objects anywhere since they are travelling in roughly opposite directions from the Solar System.
In December 2004, Voyager 1 crossed the termination shock, where the solar wind is slowed to subsonic speed, and entered the heliosheath, where the solar wind is compressed and made turbulent due to interactions with the interstellar medium. On 10 December 2007, Voyager 2 also reached the termination shock, about 1.6 billion kilometres (1 billion miles) closer to the Sun than from where Voyager 1 first crossed it, indicating that the Solar System is asymmetrical.[24]
In 2010 Voyager 1 reported that the outward velocity of the solar wind had dropped to zero, and scientists predicted it was nearing interstellar space.[25] In 2011, data from the Voyagers determined that the heliosheath is not smooth, but filled with giant magnetic bubbles, theorized to form when the magnetic field of the Sun becomes warped at the edge of the Solar System.[26]
In June 2012, Scientists at NASA reported that Voyager 1 was very close to entering interstellar space, indicated by a sharp rise in high-energy particles from outside the Solar System.[27][28] In September 2013, NASA announced that Voyager 1 had crossed the heliopause on 25 August 2012, making it the first spacecraft to enter interstellar space.[29][30][31]
In December 2018, NASA announced that Voyager 2 had crossed the heliopause on 5 November 2018, making it the second spacecraft to enter interstellar space.[3]
As of 2017[update] Voyager 1 and Voyager 2 continue to monitor conditions in the outer expanses of the Solar System.[32] The Voyager spacecraft are expected to be able to operate science instruments through 2020, when limited power will require instruments to be deactivated one by one. Sometime around 2025, there will no longer be sufficient power to operate any science instruments.
In July 2019, a revised power management plan was implemented to better manage the two probes' dwindling power supply.[33]
Spacecraft design
[edit]
The Voyager spacecraft each weigh 773 kilograms (1,704 pounds). Of this total weight, each spacecraft carries 105 kilograms (231 pounds) of scientific instruments.[34] The identical Voyager spacecraft use three-axis-stabilized guidance systems that use gyroscopic and accelerometer inputs to their attitude control computers to point their high-gain antennas towards the Earth and their scientific instruments towards their targets, sometimes with the help of a movable instrument platform for the smaller instruments and the electronic photography system.
The diagram shows the high-gain antenna (HGA) with a 3.7 m (12 ft) diameter dish attached to the hollow decagonal electronics container. There is also a spherical tank that contains the hydrazine monopropellant fuel.
The Voyager Golden Record is attached to one of the bus sides. The angled square panel to the right is the optical calibration target and excess heat radiator. The three radioisotope thermoelectric generators (RTGs) are mounted end-to-end on the lower boom.
The scan platform comprises: the Infrared Interferometer Spectrometer (IRIS) (largest camera at top right); the Ultraviolet Spectrometer (UVS) just above the IRIS; the two Imaging Science Subsystem (ISS) vidicon cameras to the left of the UVS; and the Photopolarimeter System (PPS) under the ISS.
Only five investigation teams are still supported, though data is collected for two additional instruments.[35] The Flight Data Subsystem (FDS) and a single eight-track digital tape recorder (DTR) provide the data handling functions.
The FDS configures each instrument and controls instrument operations. It also collects engineering and science data and formats the data for transmission. The DTR is used to record high-rate Plasma Wave Subsystem (PWS) data, which is played back every six months.
The Imaging Science Subsystem made up of a wide-angle and a narrow-angle camera is a modified version of the slow scan vidicon camera designs that were used in the earlier Mariner flights. The Imaging Science Subsystem consists of two television-type cameras, each with eight filters in a commandable filter wheel mounted in front of the vidicons. One has a low resolution 200 mm (7.9 in) focal length wide-angle lens with an aperture of f/3 (the wide-angle camera), while the other uses a higher resolution 1,500 mm (59 in) narrow-angle f/8.5 lens (the narrow-angle camera).
Three spacecraft were built, Voyager 1 (VGR 77-1), Voyager 2 (VGR 77-3), and test spare model (VGR 77-2).[36][37]
Scientific instruments
[edit]Instrument name | Abbreviation | Description | ||||||||||||||||||||||||||||||||||||||||||||||||||||||||||||||||||||||||||||||||||||
---|---|---|---|---|---|---|---|---|---|---|---|---|---|---|---|---|---|---|---|---|---|---|---|---|---|---|---|---|---|---|---|---|---|---|---|---|---|---|---|---|---|---|---|---|---|---|---|---|---|---|---|---|---|---|---|---|---|---|---|---|---|---|---|---|---|---|---|---|---|---|---|---|---|---|---|---|---|---|---|---|---|---|---|---|---|---|
Imaging Science System
|
ISS
|
Used a two-camera system (narrow-angle/wide-angle) to provide imagery of Jupiter, Saturn and other objects along the trajectory.
| ||||||||||||||||||||||||||||||||||||||||||||||||||||||||||||||||||||||||||||||||||||
Radio Science System
|
RSS
|
Used the telecommunications system of the Voyager spacecraft to determine the physical properties of planets and satellites (ionospheres, atmospheres, masses, gravity fields, densities) and the amount and size distribution of material in the Saturn rings and the ring dimensions.
| ||||||||||||||||||||||||||||||||||||||||||||||||||||||||||||||||||||||||||||||||||||
IRIS
|
Investigated both global and local energy balance and atmospheric composition. Vertical temperature profiles were also obtained from the planets and satellites, as well as the composition, thermal properties, and size of particles in Saturn's rings.
| |||||||||||||||||||||||||||||||||||||||||||||||||||||||||||||||||||||||||||||||||||||
Ultraviolet Spectrometer
|
UVS
|
Designed to measure atmospheric properties, and to measure radiation.
| ||||||||||||||||||||||||||||||||||||||||||||||||||||||||||||||||||||||||||||||||||||
Triaxial Fluxgate Magnetometer ![]() |
MAG
|
Designed to investigate the magnetic fields of Jupiter and Saturn, the solar-wind interaction with the magnetospheres of these planets, and the interplanetary magnetic field out to the solar wind boundary with the interstellar magnetic field and beyond, if crossed.
| ||||||||||||||||||||||||||||||||||||||||||||||||||||||||||||||||||||||||||||||||||||
![]() |
PLS
|
Investigated the macroscopic properties of the plasma ions and measures electrons in the energy range from 5 eV to 1 keV.
| ||||||||||||||||||||||||||||||||||||||||||||||||||||||||||||||||||||||||||||||||||||
Low Energy Charged Particle Instrument ![]() |
LECP
|
Measures the differential in energy fluxes and angular distributions of ions, electrons and the differential in energy ion composition.
| ||||||||||||||||||||||||||||||||||||||||||||||||||||||||||||||||||||||||||||||||||||
CRS
|
Determines the origin and acceleration process, life history, and dynamic contribution of interstellar cosmic rays, the nucleosynthesis of elements in cosmic-ray sources, the behavior of cosmic rays in the interplanetary medium, and the trapped planetary energetic-particle environment.
| |||||||||||||||||||||||||||||||||||||||||||||||||||||||||||||||||||||||||||||||||||||
Planetary Radio Astronomy Investigation
|
PRA
|
Used a sweep-frequency radio receiver to study the radio-emission signals from Jupiter and Saturn.
| ||||||||||||||||||||||||||||||||||||||||||||||||||||||||||||||||||||||||||||||||||||
Photopolarimeter System
|
PPS
|
Used a 6-inch f/1.4 Dahl-Kirkham-type Cassegrain telescope with an analyzer wheel containing five analyzers of 0,60,120,45 and 135 degrees and filter wheel with eight spectral bands covering 2350 to 7500A to gather information on surface texture and composition of Jupiter, Saturn, Uranus and Neptune and information on atmospheric scattering properties and density for these planets.
| ||||||||||||||||||||||||||||||||||||||||||||||||||||||||||||||||||||||||||||||||||||
PWS
|
Provides continuous, sheath-independent measurements of the electron-density profiles at Jupiter and Saturn as well as basic information on local wave-particle interaction, useful in studying the magnetospheres.
|
-
A view of some of Voyager's instruments from below. Left: the cameras, ultraviolet and infrared spectrometers (far left), plasma detector (black box lower right), particle and radiation detectors (far right). On the boom, center and right, are plasma, particle, and cosmic ray detectors.
-
Voyager's fully extended 13-meter-long magnetometer boom
Computers and data processing
[edit]There are three different computer types on the Voyager spacecraft, two of each kind, sometimes used for redundancy. They are proprietary, custom-built computers built from CMOS and TTL medium-scale CMOS integrated circuits and discrete components, mostly from the 7400 series of Texas Instruments.[40] Total number of words among the six computers is about 32K. Voyager 1 and Voyager 2 have identical computer systems.[41][42]
The Computer Command System (CCS), the central controller of the spacecraft, has two 18-bit word, interrupt-type processors with 4096 words each of non-volatile plated-wire memory. During most of the Voyager mission the two CCS computers on each spacecraft were used non-redundantly to increase the command and processing capability of the spacecraft. The CCS is nearly identical to the system flown on the Viking spacecraft.[43]
The Flight Data System (FDS) is two 16-bit word machines with modular memories and 8198 words each.
The Attitude and Articulation Control System (AACS) is two 18-bit word machines with 4096 words each.
Unlike the other on-board instruments, the operation of the cameras for visible light is not autonomous, but rather it is controlled by an imaging parameter table contained in one of the on-board digital computers, the Flight Data Subsystem (FDS). More recent space probes, since about 1990, usually have completely autonomous cameras.
The computer command subsystem (CCS) controls the cameras. The CCS contains fixed computer programs such as command decoding, fault detection, and correction routines, antenna-pointing routines, and spacecraft sequencing routines. This computer is an improved version of the one that was used in the Viking orbiter.[43] The hardware in both custom-built CCS subsystems in the Voyagers is identical. There is only a minor software modification for one of them that has a scientific subsystem that the other lacks.
According to Guinness Book of Records, CCS holds record of "longest period of continual operation for a computer". It has been running continuously since 20 August 1977.[44]
The Attitude and Articulation Control Subsystem (AACS) controls the spacecraft orientation (its attitude). It keeps the high-gain antenna pointing towards the Earth, controls attitude changes, and points the scan platform. The custom-built AACS systems on both craft are identical.
It has been erroneously reported[45] on the Internet that the Voyager space probes were controlled by a version of the RCA 1802 (RCA CDP1802 "COSMAC" microprocessor), but such claims are not supported by the primary design documents. The CDP1802 microprocessor was used later in the Galileo space probe, which was designed and built years later. The digital control electronics of the Voyagers were not based on a microprocessor integrated-circuit chip.
Communications
[edit]The uplink communications are executed via S-band microwave communications. The downlink communications are carried out by an X-band microwave transmitter on board the spacecraft, with an S-band transmitter as a back-up. All long-range communications to and from the two Voyagers have been carried out using their 3.7-meter (12 ft) high-gain antennas. The high-gain antenna has a beamwidth of 0.5° for X-band, and 2.3° for S-band.[46]: 17 (The low-gain antenna has a 7 dB gain and 60° beamwidth.)[46]: 17
Because of the inverse-square law in radio communications, the digital data rates used in the downlinks from the Voyagers have been continually decreasing the farther that they get from the Earth. For example, the data rate used from Jupiter was about 115,000 bits per second. That was halved at the distance of Saturn, and it has gone down continually since then.[46] Some measures were taken on the ground along the way to reduce the effects of the inverse-square law. In between 1982 and 1985, the diameters of the three main parabolic dish antennas of the Deep Space Network were increased from 64 to 70 m (210 to 230 ft)[46]: 34 dramatically increasing their areas for gathering weak microwave signals.
Whilst the craft were between Saturn and Uranus the onboard software was upgraded to do a degree of image compression and to use a more efficient Reed-Solomon error-correcting encoding.[46]: 33
Then between 1986 and 1989, new techniques were brought into play to combine the signals from multiple antennas on the ground into one, more powerful signal, in a kind of an antenna array.[46]: 34 This was done at Goldstone, California, Canberra (Australia), and Madrid (Spain) using the additional dish antennas available there. Also, in Australia, the Parkes Radio Telescope was brought into the array in time for the fly-by of Neptune in 1989. In the United States, the Very Large Array in New Mexico was brought into temporary use along with the antennas of the Deep Space Network at Goldstone.[46]: 34 Using this new technology of antenna arrays helped to compensate for the immense radio distance from Neptune to the Earth.
Power
[edit]
Electrical power is supplied by three MHW-RTG radioisotope thermoelectric generators (RTGs). They are powered by plutonium-238 (distinct from the Pu-239 isotope used in nuclear weapons) and provided approximately 470 W at 30 volts DC when the spacecraft was launched. Plutonium-238 decays with a half-life of 87.74 years,[47] so RTGs using Pu-238 will lose a factor of 1−0.5(1/87.74) = 0.79% of their power output per year.
In 2011, 34 years after launch, the thermal power generated by such an RTG would be reduced to (1/2)(34/87.74) ≈ 76% of its initial power. The RTG thermocouples, which convert thermal power into electricity, also degrade over time reducing available electric power below this calculated level.
By 7 October 2011 the power generated by Voyager 1 and Voyager 2 had dropped to 267.9 W and 269.2 W respectively, about 57% of the power at launch. The level of power output was better than pre-launch predictions based on a conservative thermocouple degradation model. As the electrical power decreases, spacecraft loads must be turned off, eliminating some capabilities. There may be insufficient power for communications by 2032.[48]
Voyager Interstellar Mission
[edit]
Voyager 2 crossed the heliosheath in November 2018.[3][49]
The Voyager primary mission was completed in 1989, with the close flyby of Neptune by Voyager 2. The Voyager Interstellar Mission (VIM) is a mission extension, which began when the two spacecraft had already been in flight for over 12 years.[50] The Heliophysics Division of the NASA Science Mission Directorate conducted a Heliophysics Senior Review in 2008. The panel found that the VIM "is a mission that is absolutely imperative to continue" and that VIM "funding near the optimal level and increased DSN (Deep Space Network) support is warranted."[51]
The main objective of the VIM was to extend the exploration of the Solar System beyond the outer planets to the heliopause (the farthest extent at which the Sun's radiation predominates over interstellar winds) and if possible even beyond. Voyager 1 crossed the heliopause boundary in 2012, followed by Voyager 2 in 2018. Passing through the heliopause boundary has allowed both spacecraft to make measurements of the interstellar fields, particles and waves unaffected by the solar wind. Two significant findings so far have been the discovery of a region of magnetic bubbles[52] and no indication of an expected shift in the Solar magnetic field.[53]
The entire Voyager 2 scan platform, including all of the platform instruments, was switched off in 1998. All platform instruments on Voyager 1, except for the ultraviolet spectrometer (UVS)[54] have also been switched off.
The Voyager 1 scan platform was scheduled to go off-line in late 2000 but has been left on to investigate UV emission from the upwind direction. UVS data are still captured but scans are no longer possible.[55]
Gyro operations ended in 2016 for Voyager 2 and in 2017 for Voyager 1. Gyro operations are used to rotate the probe 360 degrees six times per year to measure the magnetic field of the spacecraft, which is then subtracted from the magnetometer science data.
The two spacecraft continue to operate, with some loss in subsystem redundancy but retain the capability to return scientific data from a full complement of Voyager Interstellar Mission (VIM) science instruments.
Both spacecraft also have adequate electrical power and attitude control propellant to continue operating until around 2025, after which there may not be electrical power to support science instrument operation; science data return and spacecraft operations will cease.[56]
Mission details
[edit]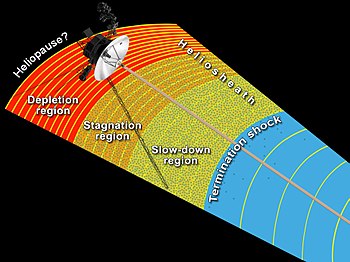
By the start of VIM, Voyager 1 was at a distance of 40 AU from the Earth, while Voyager 2 was at 31 AU. VIM is in three phases: termination shock, heliosheath exploration, and interstellar exploration phase. The spacecraft began VIM in an environment controlled by the Sun's magnetic field, with the plasma particles being dominated by those contained in the expanding supersonic solar wind. This is the characteristic environment of the termination shock phase. At some distance from the Sun, the supersonic solar wind will be held back from further expansion by the interstellar wind. The first feature encountered by a spacecraft as a result of this interaction – between interstellar wind and solar wind – was the termination shock, where the solar wind slows to subsonic speed, and large changes in plasma flow direction and magnetic field orientation occur. Voyager 1 completed the phase of termination shock in December 2004 at a distance of 94 AU, while Voyager 2 completed it in August 2007 at a distance of 84 AU. After entering into the heliosheath, the spacecraft were in an area that is dominated by the Sun's magnetic field and solar wind particles. After passing through the heliosheath, the two Voyagers began the phase of interstellar exploration. The outer boundary of the heliosheath is called the heliopause. This is the region where the Sun's influence begins to decrease and interstellar space can be detected.[58]
Voyager 1 is escaping the Solar System at the speed of 3.6 AU per year 35° north of the ecliptic in the general direction of the solar apex in Hercules, while Voyager 2's speed is about 3.3 AU per year, heading 48° south of the ecliptic. The Voyager spacecraft will eventually go on to the stars. In about 40,000 years, Voyager 1 will be within 1.6 light years (ly) of AC+79 3888, also known as Gliese 445, which is approaching the Sun. In 40,000 years Voyager 2 will be within 1.7 ly of Ross 248 (another star which is approaching the Sun), and in 296,000 years it will pass within 4.6 ly of Sirius, which is the brightest star in the night-sky.[2] The spacecraft are not expected to collide with a star for 1 sextillion (1020) years.[59]
In October 2020, astronomers reported a significant unexpected increase in density in the space beyond the Solar System, as detected by the Voyager space probes. According to the researchers, this implies that "the density gradient is a large-scale feature of the VLISM (very local interstellar medium) in the general direction of the heliospheric nose".[60][61]
Voyager Golden Record
[edit]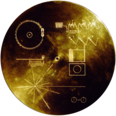
Both spacecraft carry a 12-inch (30 cm) golden phonograph record that contains pictures and sounds of Earth, symbolic directions on the cover for playing the record, and data detailing the location of Earth.[32][28] The record is intended as a combination time capsule and an interstellar message to any civilization, alien or far-future human, that may recover either of the Voyagers. The contents of this record were selected by a committee that included Timothy Ferris and was chaired by Carl Sagan.[28]
Pale Blue Dot
[edit]
Pale Blue Dot is a photograph of Earth taken on February 14, 1990, by the Voyager 1 space probe from a distance of approximately 6 billion kilometers (3.7 billion miles, 40.5 AU), as part of that day's Family Portrait series of images of the Solar System.[62] The Voyager program's discoveries during the primary phase of its mission, including new close-up color photos of the major planets, were regularly documented by print and electronic media outlets. Among the best-known of these is an image of the Earth as a Pale Blue Dot, taken in 1990 by Voyager 1, and popularized by Carl Sagan,[63]
Consider again that dot. That's here. That's home. That's us....The Earth is a very small stage in a vast cosmic arena.... To my mind, there is perhaps no better demonstration of the folly of human conceits than this distant image of our tiny world. To me, it underscores our responsibility to deal more kindly and compassionately with one another and to preserve and cherish that pale blue dot, the only home we've ever known.
See also
[edit]- Family Portrait
- The Farthest, a 2017 documentary on the program.
- Interstellar Express, a pair of Chinese probes inspired in part by the Voyagers.
- Interstellar probe
- Pioneer program
- Planetary Grand Tour
- Timeline of Solar System exploration
References
[edit]- ^ a b "The Fantastic Voyage of Voyager". The Attic. 9 January 2020. Archived from the original on 13 April 2020. Retrieved 3 March 2020.
- ^ a b Jpl.Nasa.Gov. "Voyager Enters Interstellar Space – NASA Jet Propulsion Laboratory". Jpl.nasa.gov. Archived from the original on 13 April 2020. Retrieved 14 September 2013.
- ^ a b c Brown, Dwayne; Fox, Karen; Cofield, Calia; Potter, Sean (10 December 2018). "Release 18-115 – NASA's Voyager 2 Probe Enters Interstellar Space". NASA. Archived from the original on 27 June 2023. Retrieved 10 December 2018.
- ^ University of Iowa (4 November 2019). "Voyager 2 reaches interstellar space – Iowa-led instrument detects plasma density jump, confirming spacecraft has entered the realm of the stars". EurekAlert!. Archived from the original on 13 April 2020. Retrieved 4 November 2019.
- ^ Chang, Kenneth (4 November 2019). "Voyager 2's Discoveries From Interstellar Space – In its journey beyond the boundary of the solar wind's bubble, the probe observed some notable differences from its twin, Voyager 1". The New York Times. Archived from the original on 13 April 2020. Retrieved 5 November 2019.
- ^ "Solar System Exploration". JPL-NASA. Archived from the original on 18 April 2019. Retrieved 19 February 2021.
- ^ Gladstone, G. Randall; et al. (7 August 2018). "The Lyman-α Sky Background as Observed by New Horizons". Geophysical Research Letters. 45 (16): 8022–8028. arXiv:1808.00400. Bibcode:2018GeoRL..45.8022G. doi:10.1029/2018GL078808. S2CID 119395450.
- ^ Letzter, Rafi (9 August 2018). "NASA Spotted a Vast, Glowing 'Hydrogen Wall' at the Edge of Our Solar System". Live Science. Archived from the original on 13 April 2020. Retrieved 10 August 2018.
- ^ "Voyager – Fact Sheet". voyager.jpl.nasa.gov. Archived from the original on 13 April 2020. Retrieved 17 May 2018.
- ^ "Voyager Mission Status". JPL. Archived from the original on 1 January 2018. Retrieved 10 February 2022.
- ^ a b "Voyager – Mission Status". Jet Propulsion Laboratory. National Aeronautics and Space Administration. Archived from the original on 1 January 2018. Retrieved 24 April 2021.
- ^ "In Depth – Voyager 2". JPL. Archived from the original on 20 April 2017. Retrieved 10 February 2022.
- ^ Dave Doody (15 September 2004). "Basics of Space Flight Section I. The Environment of Space". .jpl.nasa.gov. Archived from the original on 17 August 2015. Retrieved 29 December 2017.
- ^ Flandro, Gary (1966). "Fast Reconnaissance Missions to the Outer Solar System Using Energy Derived from the Gravitational Field of Jupiter" (PDF). Astronautica Acta. 12: 329–337. Archived (PDF) from the original on 30 March 2019. Retrieved 1 June 2024.
- ^ "Planetary Voyage". USA.gov. 30 October 2013. Archived from the original on 27 November 2013. Retrieved 15 October 2013.
- ^ a b c Butrica, Andrew J. (1998). "Voyager: The Grand Tour of Big Science". In Mack, Pamela E. (ed.). From Engineering Science to Big Science: The NACA and NASA Collier Trophy Research Project Winners. Washington, D.C.: NASA. ISBN 978-1-4102-2531-3. Archived from the original on 23 August 2014. Retrieved 25 August 2014.
- ^ a b c Smurmeier, H. M. (1 April 1974). "The Mariner Jupiter/Saturn 1977 Mission" (1974)". Embry–Riddle Aeronautical University. Archived from the original on 20 April 2023. Retrieved 16 May 2024.
- ^ "The Voyagers: An unprecedented on-going mission of exploration". NASASpaceFlight.com. Jeff Goldader, Chris Gebhardt. 7 August 2011. Archived from the original on 17 May 2024. Retrieved 17 May 2024.
- ^ Chapter 11 "Voyager: The Grand Tour of Big Science" Archived 29 February 2020 at the Wayback Machine (sec. 268.), by Andrew,J. Butrica, found in From Engineering Science To Big Science ISBN 978-0-16-049640-0 edited by Pamela E. Mack, NASA, 1998
- ^ David W. Swift (1 January 1997). Voyager Tales: Personal Views of the Grand Tour. AIAA. p. 69. ISBN 978-1-56347-252-7.
- ^ "Voyager FAQ". Jet Propulsion Laboratory. Archived from the original on 21 July 2011. Retrieved 1 January 2015.
- ^ Jim Bell (24 February 2015). The Interstellar Age: Inside the Forty-Year Voyager Mission. Penguin Publishing Group. p. 94. ISBN 978-0-698-18615-6. Archived from the original on 24 July 2024. Retrieved 9 February 2016.
- ^ Alan Stern (23 June 2014). "The PI's Perspective: What If Voyager Had Explored Pluto?". New Horizons: NASA's Mission to Pluto and the Kuiper Belt. Retrieved 29 August 2020.
- ^ "NASA - Voyager 2 Proves Solar System Is Squashed". www.nasa.gov. Archived from the original on 13 April 2020. Retrieved 6 February 2020.
- ^ Brown, Dwayne; Cook, Jia-Rui; Buckley, M. (14 December 2010). "Nearing Interstellar Space, NASA Probe Sees Solar Wind Decline". Applied Physics Lab, Johns Hopkins University. Archived from the original on 15 December 2010.
- ^ Smith, Catharine (10 June 2011). "WATCH: NASA Discovers 'Bubbles' At Solar System's Edge". Huffington Post. Archived from the original on 13 April 2020. Retrieved 11 June 2011.
- ^ Amos, Jonathan (15 June 2012). "Particles point way for Nasa's Voyager". BBC News. Archived from the original on 15 June 2012. Retrieved 15 June 2012.
- ^ a b c Ferris, Timothy (May 2012). "Timothy Ferris on Voyagers' Never-Ending Journey". Smithsonian Magazine. Archived from the original on 4 November 2013. Retrieved 15 June 2012.
- ^ Cook, Jia-Rui C.; Agle, D. C.; Brown, Dwayne (12 September 2013). "NASA Spacecraft Embarks on Historic Journey into Interstellar Space". NASA. Archived from the original on 11 June 2020. Retrieved 12 September 2013.
- ^ "Voyager 1 has entered a new region of space, sudden changes in cosmic rays indicate". Archived from the original on 22 March 2013. Retrieved 20 March 2013.
- ^ "Report: NASA Voyager Status Update on Voyager 1 Location". NASA. Archived from the original on 13 April 2020. Retrieved 20 March 2013.
- ^ a b Krauss, Lawrence M. (5 September 2017). "Pondering Voyagers' Interstellar Journeys, and Our Own". The New York Times. Archived from the original on 13 April 2020. Retrieved 5 September 2017.
- ^ Cofield, Calla (8 July 2019). "A New Plan for Keeping NASA's Oldest Explorers Going". NASA. Archived from the original on 13 April 2020. Retrieved 12 July 2019.
- ^ Haynes, Robert (January 1987). "How We Get Pictures from Space, Revised Edition". NASA Facts. NTRS. Archived from the original on 30 July 2023. Retrieved 7 July 2017.
- ^ Voyager - Spacecraft Archived 24 March 2007 at the Wayback Machine Nasa website
- ^ Pyne 2010, p. 39.
- ^ Folger, Tim (July 2022). "Record-Breaking Voyager Spacecraft Begin to Power Down". Scientific American. Archived from the original on 23 June 2022. Retrieved 12 April 2024.
- ^ "Voyager 1 Narrow Angle Camera Description". NASA. Archived from the original on 11 August 2011. Retrieved 17 January 2011.
- ^ "Voyager 1 Wide Angle Camera Description". NASA. Archived from the original on 7 November 2021. Retrieved 17 January 2011.
- ^ "Voyagers 1 and 2 Take Embedded Computers into Interstellar Space". 25 July 2022. Archived from the original on 5 August 2023. Retrieved 5 August 2023.
- ^ "Voyager Frequently Asked Questions". Archived from the original on 21 July 2011.
- ^ "Voyager 1 Instrument Host Information". seti.org. Archived from the original on 24 July 2024. Retrieved 10 August 2019.
- ^ a b Tomayko, James E. (3 August 1987). "Distributed Computing On Board Voyager and Galileo (chapter 6)". In Kent, Allen; Williams, James G. (eds.). Computers in Spaceflight: The NASA Experience. Encyclopedia of Computer Science and Technology. Vol. 18. Supplement 3. NASA. ISBN 978-0-8247-2268-5. Archived from the original on 18 October 2023. Retrieved 26 July 2022 – via NASA History.
- ^ "Longest period of continual operation for a computer". Guinness World Records. 20 August 1977. Archived from the original on 28 April 2023. Retrieved 28 April 2023.
- ^ Johnson, Herb (November 2014). "COSMAC 1802 History in Space". Archived from the original on 15 July 2015. Retrieved 27 July 2015.
- ^ a b c d e f g Ludwig, Roger; Taylor, Jim (March 2002). "Voyager Telecommunications" (PDF). NASA. Archived (PDF) from the original on 18 March 2021. Retrieved 26 March 2016.
- ^ "The Actinide Research Quarterly: Summer 1997". lanl.gov. Archived from the original on 8 March 2022. Retrieved 6 February 2020.
- ^ Segal, Michael (1 September 2017). "Beyond Voyager". Nautilus. Archived from the original on 2 September 2017. Retrieved 2 September 2017.
- ^ Cofield, Calia; Cook, Jia-Rui; Fox, Karen (5 October 2018). "NASA Voyager 2 Could Be Nearing Interstellar Space". NASA. Archived from the original on 5 October 2018. Retrieved 6 October 2018.
- ^ "Interstellar Mission". NASA. Archived from the original on 15 October 2009. Retrieved 30 May 2008.
- ^ "Senior Review 2008 of the Mission Operations and Data Analysis Program for the Heliophysics Operating Missions" (PDF). NASA. p. 7. Archived from the original (PDF) on 26 June 2008. Retrieved 30 May 2008.
- ^ "GMS: Voyager Satellites Find Magnetic Bubbles at Edge of Solar System". 9 June 2011. Archived from the original on 5 December 2022. Retrieved 11 August 2022.
- ^ Grant, Andrew (2019). "The confounding magnetic readings of Voyager 1". Physics Today. doi:10.1063/pt.6.3.20190215a. S2CID 242207067. Archived from the original on 15 August 2022. Retrieved 11 August 2022.
- ^ "Ultraviolet Spectrometer". Voyager: The Interstellar Mission. NASA JPL. Archived from the original on 5 March 2006. Retrieved 11 June 2006.
- ^ E. C. Stone; J. D. Richardson; E. B. Massey. "The Voyager Interstellar Mission Proposal to Senior Review 2010 of the Mission Operations and Data Analysis Program for the Heliophysics Operating Missions" (PDF). NASA. p. 24. Archived from the original (PDF) on 23 December 2016. Retrieved 20 November 2016.
- ^ "Voyager – Spacecraft Lifetime NASA website". Archived from the original on 1 March 2017. Retrieved 13 September 2011.
- ^ "NASA – Transitional Regions at the Heliosphere's Outer Limits". Archived from the original on 8 July 2013.
- ^ JPL.NASA.GOV. "Voyager – The Interstellar Mission". voyager.jpl.nasa.gov. Archived from the original on 15 October 2009. Retrieved 27 May 2016.
- ^ Coryn A.L. Bailer-Jones, Davide Farnocchia (3 April 2019). "Future stellar flybys of the Voyager and Pioneer spacecraft". Research Notes of the American Astronomical Society. 3 (4): 59. arXiv:1912.03503. Bibcode:2019RNAAS...3...59B. doi:10.3847/2515-5172/ab158e. S2CID 134524048.
- ^ Starr, Michelle (19 October 2020). "Voyager Spacecraft Detect an Increase in The Density of Space Outside The Solar System". ScienceAlert. Archived from the original on 19 October 2020. Retrieved 19 October 2020.
- ^ Kurth, W.S.; Gurnett, D.A. (25 August 2020). "Observations of a Radial Density Gradient in the Very Local Interstellar Medium by Voyager 2". The Astrophysical Journal Letters. 900 (1): L1. Bibcode:2020ApJ...900L...1K. doi:10.3847/2041-8213/abae58. S2CID 225312823.
- ^ Staff (12 February 2020). "Pale Blue Dot Revisited". NASA. Archived from the original on 12 February 2020. Retrieved 12 February 2020.
- ^ Sagan, Carl (1997). Pale Blue Dot. United States: Random House USA Inc. p. 6-7. ISBN 978-0-345-37659-6.
Further reading
[edit]- Swift, David W. (1997). Voyager Tales. Reston, Va: American Institute of Aeronautics and Astronautics. ISBN 978-1-56347-252-7.
- Gallentine, Jay (2009). Ambassadors from Earth: Pioneering Explorations with Unmanned Spacecraft. Lincoln: U of Nebraska Press. ISBN 978-0-8032-2220-5.
- Pyne, Stephen J. (2010). Voyager: Exploration, Space, and the Third Great Age of Discovery. Penguin Books. ISBN 978-0-14-311959-3.
- Bell, Jim (2015). The Interstellar Age: Inside the Forty-Year Voyager Mission. Penguin Publishing Group. ISBN 978-0-698-18615-6.
External links
[edit]
NASA sites
- NASA Voyager website
- Voyager Mission status (updated in real time)
- Voyager Spacecraft Lifetime
- NASA Facts – Voyager Mission to the Outer Planets
- Voyager 1 and 2 atlas of six Saturnian satellites, 1984
- JPL Voyager Telecom Manual
NASA instrument information pages:
- "Voyager instrument overview". Archived from the original on 21 July 2011.
- "CRS – COSMIC RAY SUBSYSTEM". Archived from the original on 3 August 2014. Retrieved 11 November 2017.
- "ISS NA – IMAGING SCIENCE SUBSYSTEM – NARROW ANGLE". NASA. Retrieved 2 April 2023.
- "ISS WA – IMAGING SCIENCE SUBSYSTEM – WIDE ANGLE". Archived from the original on 18 July 2009. Retrieved 29 October 2009.
- "IRIS – INFRARED INTERFEROMETER SPECTROMETER AND RADIOMETER". Archived from the original on 18 July 2009. Retrieved 29 October 2009.
- "LECP – LOW ENERGY CHARGED PARTICLE". Archived from the original on 18 July 2009. Retrieved 29 October 2009.
- "MAG – TRIAXIAL FLUXGATE MAGNETOMETER". Archived from the original on 18 July 2009. Retrieved 29 October 2009.
- "PLS – PLASMA SCIENCE EXPERIMENT". Archived from the original on 18 July 2009. Retrieved 29 October 2009.
- "PPS – PHOTOPOLARIMETER SUBSYSTEM". Archived from the original on 25 August 2009. Retrieved 29 October 2009.
- "PRA – PLANETARY RADIO ASTRONOMY RECEIVER". Archived from the original on 18 July 2009. Retrieved 29 October 2009.
- "PWS – PLASMA WAVE RECEIVER". Archived from the original on 18 July 2009. Retrieved 29 October 2009.
- "RSS – RADIO SCIENCE SUBSYSTEM". Archived from the original on 3 August 2014. Retrieved 11 November 2017.
- "UVS – ULTRAVIOLET SPECTROMETER". Archived from the original on 3 August 2014. Retrieved 11 November 2017.
Non-NASA sites
- Spacecraft Escaping the Solar System – current positions and diagrams
- NPR: Science Friday 8/24/07 Interviews for 30th anniversary of Voyager spacecraft
- Illustrated technical paper by RL Heacock, the project engineer
- Gray, Meghan. "Voyager and Interstellar Space". Deep Space Videos. Brady Haran.
- PBS featured documentary The Farthest-Voyager in Space
- Voyager image album by Kevin M. Gill