ТРАППИСТ-1
Данные наблюдений Эпоха J2000 Равноденствие J2000 | |
---|---|
Созвездие | Водолей |
Прямое восхождение | 23 час 06 м 29.368 с [1] |
Склонение | −05° 02′ 29.04″ [1] |
Apparent magnitude (V) | 18.798±0.082[2] |
Characteristics | |
Evolutionary stage | Main sequence |
Spectral type | M8V[3] |
Apparent magnitude (R) | 16.466±0.065[2] |
Apparent magnitude (I) | 14.024±0.115[2] |
Apparent magnitude (J) | 11.354±0.022[4] |
Apparent magnitude (H) | 10.718±0.021[4] |
Apparent magnitude (K) | 10.296±0.023[4] |
V−R color index | 2.332 |
R−I color index | 2.442 |
J−H color index | 0.636 |
J−K color index | 1.058 |
Astrometry | |
Proper motion (μ) | RA: 930.788[1] mas/yr Dec.: −479.038[1] mas/yr |
Parallax (π) | 80.2123 ± 0.0716 mas[1] |
Distance | 40.66 ± 0.04 ly (12.47 ± 0.01 pc) |
Details | |
Mass | 0.0898±0.0023[5] M☉ |
Radius | 0.1192±0.0013[5] R☉ |
Luminosity (bolometric) | 0.000553±0.000018[5] L☉ |
Surface gravity (log g) | 5.2396+0.0056 −0.0073[a][5] cgs |
Temperature | 2,566±26[5] K |
Metallicity [Fe/H] | 0.04±0.08[6] dex |
Rotation | 3.295±0.003 days[7] |
Rotational velocity (v sin i) | 6[8] km/s |
Age | 7.6±2.2[9] Gyr |
Other designations | |
Database references | |
SIMBAD | data |
Exoplanet Archive | data |
TRAPPIST-1 — холодный красный карлик. [с] с семью известными экзопланетами . Он расположен в созвездии Водолея на расстоянии около 40,66 световых лет от Земли и имеет температуру поверхности около 2566 К (2290 °C ; 4160 °F ). Его радиус немного больше Юпитера , а масса составляет около 9% солнечной . Его возраст оценивается в 7,6 миллиардов лет, что делает его старше Солнечной системы . Об открытии звезды впервые было опубликовано в 2000 году.
Наблюдения в 2016 году с помощью Малого телескопа транзитных планет и планетезималей (TRAPPIST) в обсерватории Ла Силья в Чили и других телескопов привели к открытию двух планет земной группы на орбите вокруг TRAPPIST-1. В 2017 году дальнейший анализ первоначальных наблюдений выявил еще пять планет земной группы. Семи планетам требуется от 1,5 до 19 дней, чтобы вращаться вокруг звезды по круговым орбитам. Вероятно, они приливно привязаны к TRAPPIST-1, так что одна сторона каждой планеты всегда обращена к звезде, что приводит к постоянному дню на одной стороне и постоянной ночи на другой. Их массы сравнимы с массами Земли, и все они лежат в одной плоскости; с Земли они словно движутся мимо диска звезды.
Up to four of the planets – designated d, e, f and g – orbit at distances where temperatures are suitable for the existence of liquid water, and are thus potentially hospitable to life. There is no evidence of an atmosphere on any of the planets, and observations of TRAPPIST-1 b have ruled out the existence of an atmosphere. It is unclear whether radiation emissions from TRAPPIST-1 would allow for such atmospheres. The planets have low densities; they may consist of large amounts of volatile materials. Due to the possibility of several of the planets being habitable, the system has drawn interest from researchers and has appeared in popular culture.
Discovery
[edit]The star now known as TRAPPIST-1 was discovered in 1999 by astronomer John Gizis and colleagues[16] during a survey of close-by ultra-cool dwarf stars.[17][18] It appeared in sample C[16][17] of the surveyed stars, which was obtained in June 1999. Publication of the discovery took place in 2000.[19] The name is a reference to the TRansiting Planets and PlanetesImals Small Telescope (TRAPPIST)[11][d] project that discovered the first two exoplanets around the star.[23]
Its planetary system was discovered by a team led by Michaël Gillon, a Belgian astronomer[24] at the University of Liege,[25] in 2016[26] during observations made at the La Silla Observatory, Chile,[27][28] using the TRAPPIST telescope. The discovery was based on anomalies in the light curves[e] measured by the telescope in 2015. These were initially interpreted as indicating the existence of three planets. In 2016, separate discoveries revealed that the third planet was in fact multiple planets. The telescopes and observatories involved were[11] the Spitzer Space Telescope; the ground-based TRAPPIST and TRAPPIST-North in Oukaïmeden Observatory, Morocco; the South African Astronomical Observatory; and the Liverpool Telescopes and William Herschel Telescopes in Spain.[30]
The observations of TRAPPIST-1 are considered among the most important research findings of the Spitzer Space Telescope.[31] Complementing the findings were observations by the Himalayan Chandra Telescope, the United Kingdom Infrared Telescope, and the Very Large Telescope.[32] Since then, research has confirmed the existence of at least seven planets in the system,[33] the orbits of which have been calculated using measurements from the Spitzer and Kepler telescopes.[34] Some news reports incorrectly attributed the discovery of the TRAPPIST-1 planets to NASA; in fact the TRAPPIST project that led to their discovery received funding from both NASA and the European Research Council of the European Union (EU).[35]
Description
[edit]
TRAPPIST-1 is in the constellation Aquarius,[25] five degrees south of the celestial equator.[f][1][37] It is a relatively close star[38] located 40.66±0.04 light-years from Earth,[g][1] with a large proper motion[h][38]and no companion stars.[41]
It is a red dwarf of spectral class M8.0±0.5,[i][32][44] meaning it is relatively small and cold.[45] With a radius 12% of that of the Sun, TRAPPIST-1 is only slightly larger than the planet Jupiter (though much more massive).[32] Its mass is approximately 9% of that of the Sun,[45] being just sufficient to allow nuclear fusion to take place.[46][47] TRAPPIST-1's density is unusually low for a red dwarf.[48] It has a low effective temperature[j] of 2,566 K (2,293 °C) making it, as of 2022[update], the coldest-known star to host planets.[50] TRAPPIST-1 is cold enough for condensates to form in its photosphere;[k] these have been detected through the polarisation they induce in its radiation during transits of its planets.[52]
There is no evidence that it has a stellar cycle.[l][54] Its luminosity, emitted mostly as infrared radiation, is about 0.055% that of the Sun.[45][55] Low precision[56] measurements from the XMM-Newton satellite[57] and other facilities[58] show that the star emits faint radiation at short wavelengths such as x-rays and UV radiation.[m][57] There are no detectable radio wave emissions.[60]
Rotation period and age
[edit]Measurements of TRAPPIST-1's rotation have yielded a period of 3.3 days; earlier measurements of 1.4 days appear to have been caused by changes in the distribution of its starspots.[61] Its rotational axis may be slightly offset from that of its planets.[62]
Using a combination of techniques, the age of TRAPPIST-1 has been estimated at about 7.6±2.2 billion years,[63] making it older than the Solar System, which is about 4.5 billion years old.[64] It is expected to shine for ten trillion years – about 700 times[65] longer than the present age of the Universe[66] – whereas the Sun will run out of hydrogen and leave the main sequence[n] in a few billion years.[65]
Activity
[edit]Photospheric features have been detected on TRAPPIST-1.[68] The Kepler and Spitzer Space Telescopes have observed possible bright spots, which may be faculae,[o][70][71] although some of these may be too large to qualify as such.[72] Bright spots are correlated to the occurrence of some stellar flares.[p][74]
The star has a strong magnetic field[75] with a mean intensity of about 600 gauss.[q][77] The magnetic field drives high chromospheric[r][75] activity, and may be capable of trapping coronal mass ejections.[s][69][78]
According to Garraffo et al. (2017), TRAPPIST-1 loses about 3×10−14 solar masses per year[79] to the stellar wind, a rate which is about 1.5 times that of the Sun. [80] Dong et al. (2018) simulated the observed properties of TRAPPIST-1 with a mass loss of 4.1×10−15 solar masses per year.[79] Simulations to estimate mass loss are complicated because, as of 2019, most of the parameters that govern TRAPPIST-1's stellar wind are not known from direct observation.[81]
Planetary system
[edit]
TRAPPIST-1 is orbited by seven planets, designated TRAPPIST-1b, 1c, 1d, 1e, 1f, 1g, and 1h[82] in alphabetic order going out from the star.[t][85] These planets have orbital periods ranging from 1.5–19 days,[86][87][6] at distances of 0.011–0.059 astronomical units[u] (1,700,000–8,900,000 km).[89]
All the planets are much closer to their star than Mercury is to the Sun,[26] making the TRAPPIST-1 system very compact.[90] Kral et al. (2018) did not detect any comets around TRAPPIST-1,[91] and Marino et al. (2020) found no evidence of a Kuiper belt,[92] although it is uncertain whether a Solar System-like belt around TRAPPIST-1 would be observable from Earth.[93] Observations with the Atacama Large Millimeter Array found no evidence of a circumstellar dust disk.[94]
The inclinations of planetary orbits relative to the system's ecliptic are less than 0.1 degrees[v],[96] making TRAPPIST-1 the flattest planetary system in the NASA Exoplanet Archive.[97] The orbits are highly circular, with minimal eccentricities[w][90] and are well-aligned with the spin axis of TRAPPIST-1.[99] The planets orbit in the same plane and, from the perspective of the Solar System, transit TRAPPIST-1 during their orbit[100] and frequently pass in front of each other.[101]
Size and composition
[edit]The radii of the planets are estimated to range between 77.5+1.4
−1.4 and 112.9+1.5
−1.3% of Earth's radius.[102] The planet/star mass ratio of the TRAPPIST-1 system resembles that of the moon/planet ratio of the Solar System's gas giants.[103]
The TRAPPIST-1 planets are expected to have compositions that resemble each other[104] as well as that of Earth.[105] The estimated densities of the planets are lower than Earth's[34] which may imply that they have large amounts of volatile chemicals.[x] Alternatively, their cores may be smaller than that of Earth and therefore they may be rocky planets with less iron than that of Earth,[107][108] include large amounts of elements other than iron,[109] or their iron may exist in an oxidised form rather than as a core.[108] Their densities are too low for a pure magnesium silicate composition,[y] requiring the presence of lower-density compounds such as water.[111][112] Planets b, d, f, g and h are expected to contain large quantities of volatile chemicals.[113] The planets may have deep atmospheres and oceans, and contain vast amounts of ice.[114] Subsurface oceans, buried under icy shells, would form in the colder planets.[115] Several compositions are possible considering the large uncertainties in their densities.[116] The photospheric features of the star may introduce inaccuracies in measurements of the properties of TRAPPIST-1's planets,[68] including their densities being underestimated by 8+20
-7 percent,[117] and incorrect estimates of their water content.[118]
Resonance and tides
[edit]The planets are in orbital resonances.[119] The durations of their orbits have ratios of 8:5, 5:3, 3:2, 3:2, 4:3 and 3:2 between neighbouring planet pairs,[120] and each set of three is in a Laplace resonance.[z][90] Simulations have shown such resonances can remain stable over billions of years but that their stability is strongly dependent on initial conditions. Many configurations become unstable after less than a million years. The resonances enhance the exchange of angular momentum between the planets, resulting in measurable variations – earlier or later – in their transit times in front of TRAPPIST-1. These variations yield information on the planetary system,[122] such as the masses of the planets, when other techniques are not available.[123] The resonances and the proximity to the host star have led to comparisons between the TRAPPIST-1 system and the Galilean moons of Jupiter.[100] Kepler-223 is another exoplanet system with a TRAPPIST-1-like long resonance.[124]
The mutual interactions of the planets could prevent them from reaching full synchronisation, which would have important implications for the planets' climates. These interactions could force periodic or episodic full rotations of the planets' surfaces with respect to the star on timescales of several Earth years.[125] Vinson, Tamayo and Hansen (2019) found the planets TRAPPIST-1d, e and f likely have chaotic rotations due to mutual interactions, preventing them from becoming synchronised to their star. Lack of synchronisation potentially makes the planets more habitable.[126] Other processes that can prevent synchronous rotation are torques induced by stable triaxial deformation of the planets,[aa] which would allow them to enter 3:2 resonances.[128]
The closeness of the planets to TRAPPIST-1 results in tidal interactions[129] stronger than those on Earth.[130]All the planets have reached an equilibrium with slow planetary rotations and tidal locking,[129] which can lead to the synchronisation of a planet's rotation to its revolution around its star.[ab][132]
The planets are likely to undergo substantial tidal heating[133] due to deformations arising from their orbital eccentricities and gravitational interactions with one another.[134] Such heating would facilitate volcanism and degassing[ac] especially on the innermost planets, with degassing facilitating the establishment of atmospheres.[136] According to Luger et al. (2017), tidal heating of the four innermost planets is expected to be greater than Earth's inner heat flux.[137] For the outer planets Quick et al. (2020) noted that their tidal heating could be comparable to that in the Solar System bodies Europa, Enceladus, and Triton,[138] and may be sufficient to drive detectable cryovolcanic activity.[139]
Tidal heating could influence temperatures of the night sides and cold areas where volatiles may be trapped, and gases are expected to accumulate; it would also influence the properties of any subsurface oceans[140] where cryovolcanism,[ad][142] volcanism and hydrothermal venting[ae] could occur.[144] It may further be sufficient to melt the mantles of the four innermost planets, in whole or in part,[145] potentially forming subsurface magma oceans.[146] This heat source is likely dominant over radioactive decay, both of which have substantial uncertainties and are considerably less than the stellar radiation received.[147] Intense tides could fracture the planets' crusts even if they are not sufficiently strong to trigger the onset of plate tectonics.[148] Tides can also occur in the planetary atmospheres.[149]
Skies and impact of stellar light
[edit]
Because most of TRAPPIST-1's radiation is in the infrared region, there may be very little visible light on the planets' surfaces; Amaury Triaud, one of the system's co-discoverers, said the skies would never be brighter than Earth's sky at sunset[151] and only a little brighter than a night with a full moon. Ignoring atmospheric effects, illumination would be orange-red.[152] All of the planets would be visible from each other and would, in many cases, appear larger than Earth's Moon in the sky of Earth;[26] observers on TRAPPIST-1e, f and g, however, could never experience a total stellar eclipse.[ag][85] Assuming the existence of atmospheres, the star's long-wavelength radiation would be absorbed to a greater degree by water and carbon dioxide than sunlight on Earth; it would also be scattered less by the atmosphere[153] and less reflected by ice,[154] although the development of highly reflective hydrohalite ice may negate this effect.[155] The same amount of radiation results in a warmer planet compared to Sun-like irradiation;[153] more radiation would be absorbed by the planets' upper atmosphere than by the lower layers, making the atmosphere more stable and less prone to convection.[156]
Habitable zone
[edit]
For a dim star like TRAPPIST-1, the habitable zone[ah] is located closer to the star than for the Sun.[157] Three or four[57] planets might be located in the habitable zone; these include e, f, and g;[157] or d, e, and f.[75] As of 2017[update], this is the largest-known number of planets within the habitable zone of any known star or star system.[158] The presence of liquid water on any of the planets depends on several other factors, such as albedo (reflectivity),[159] the presence of an atmosphere[160] and any greenhouse effect.[161] Surface conditions are difficult to constrain without better knowledge of the planets' atmospheres.[160] A synchronously rotating planet might not entirely freeze over if it receives too little radiation from its star because the day-side could be sufficiently heated to halt the progress of glaciation.[162] Other factors for the occurrence of liquid water include the presence of oceans and vegetation;[163] the reflective properties of the land surface; the configuration of continents and oceans;[164] the presence of clouds;[165] and sea ice dynamics.[166] The effects of volcanic activity may extend the system's habitable zone to TRAPPIST-1h.[167] Even if the outer planets are too cold to be habitable, they may have ice-covered subsurface oceans[168] that may harbour life.[169]
Intense extreme ultraviolet (XUV) and X-ray radiation[170] can split water into its component parts of hydrogen and oxygen, and heat the upper atmosphere until they escape from the planet. This was thought to have been particularly important early in the star's history, when radiation was more intense and could have heated every planet's water to its boiling point.[154] This process is believed to have removed water from Venus.[171] In the case of TRAPPIST-1, different studies with different assumptions on the kinetics, energetics, and XUV emissions have come to different conclusions on whether any TRAPPIST-1 planet may retain substantial amounts of water. Because the planets are most likely synchronised to their host star, any water present could become trapped on the planets' night sides and would be unavailable to support life unless heat transport by the atmosphere[172] or tidal heating are intense enough to melt ice.[173]
Moons
[edit]No moons with a size comparable to Earth's have been detected in the TRAPPIST-1 system,[174] and they are unlikely in such a densely packed planetary system. This is because moons would likely be either destroyed by their planet's gravity after entering its Roche limit[ai] or stripped from the planet by leaving its Hill radius[aj][177] Although the TRAPPIST-1 planets appear in an analysis of potential exomoon hosts, they do not appear in the list of habitable-zone exoplanets that could host a moon for at least one Hubble time,[178] a timeframe slightly longer than the current age of the Universe.[179] Despite these factors, it is possible the planets could host moons.[180]
Magnetic effects
[edit]The TRAPPIST-1 planets are expected to be within the Alfvén surface of their host star,[181] the area around the star within which any planet would directly magnetically interact with the corona of the star, possibly destabilising any atmosphere the planet has.[182] Stellar energetic particles would not create a substantial radiation hazard for organisms on TRAPPIST-1 planets if atmospheres reached pressures of about 1 bar.[183] Estimates of radiation fluxes have considerable uncertainties due to the lack of knowledge about the structure of TRAPPIST-1's magnetic field.[184] Induction heating from the star's time-varying electrical and magnetic fields[145][185] may occur on its planets[186] but this would make no substantial contribution to their energy balance[147] and is vastly exceeded by tidal heating.[138]
Formation history
[edit]The TRAPPIST-1 planets most likely formed further from the star and migrated inwards,[187] although it is possible they formed in their current locations.[188] According to the most popular theory on the formation of the TRAPPIST-1 planets (Ormel et al. (2017)),[189] the planets formed when a streaming instability[ak] at the water-ice line gave rise to precursor bodies, which accumulated additional fragments and migrated inwards, eventually giving rise to planets.[191] The migration may initially have been fast and later slowed,[192] and tidal effects may have further influenced the formation processes.[193] The distribution of the fragments would have controlled the final mass of the planets, which would consist of approximately 10% water consistent with observational inference.[191] Resonant chains of planets like those of TRAPPIST-1 usually become unstable when the gas disk that gave rise to them dissipates, but in this case, the planets remained in resonance.[194] The resonance may have been either present from the system's formation and was preserved when the planets simultaneously moved inwards,[195] or it might have formed later when inward-migrating planets accumulated at the outer edge of the gas disk and interacted with each other.[188] Inward-migrating planets would contain substantial amounts of water – too much for it to entirely escape – whereas planets that formed in their current location would most likely lose all water.[196][197] According to Flock et al. (2019), the orbital distance of the innermost planet TRAPPIST-1b is consistent with the expected radius of an inward-moving planet around a star that was one order of magnitude brighter in the past,[198] and with the cavity in the protoplanetary disc created by TRAPPIST-1's magnetic field.[199] Alternatively, TRAPPIST-1h may have formed in or close to its current location.[200]
The presence of other bodies and planetesimals early in the system's history would have destabilised the TRAPPIST-1 planets' resonance if the bodies were massive enough.[201] Raymond et al. (2021) concluded the TRAPPIST-1 planets assembled in 1–2 million years, after which time little additional mass was accreted.[202] This would limit any late delivery of water to the planets[203] and also implies the planets cleared the neighbourhood[al] of any additional material.[204] The lack of giant impact events (the rapid formation of the planets would have quickly exhausted pre-planetary material) would help the planets preserve their volatile materials,[205] only once the planet formation process was complete.[206]
Due to a combination of high insolation, the greenhouse effect of water vapour atmospheres and remnant heat from the process of planet assembly, the TRAPPIST-1 planets would likely have initially had molten surfaces. Eventually the surfaces would cool until the magma oceans solidified, which in the case of TRAPPIST-1b may have taken between a few billions of years, or a few millions of years. The outer planets would then have become cold enough for water vapour to condense.[207]
List of planets
[edit]TRAPPIST-1b
[edit]TRAPPIST-1b has a semi-major axis of 0.0115 astronomical units (1,720,000 km)[208] and an orbital period of 1.51 Earth days. It is tidally locked to its star. The planet is outside the habitable zone;[209] its expected irradiation is more than four times that of Earth[209] and the James Webb Space Telescope (JWST) has measured a brightness temperature of 508+26
−27 K on the day side.[210] TRAPPIST-1b has a slightly larger measured radius and mass than Earth but estimates of its density imply it does not exclusively consist of rock.[211] Owing to its black-body temperature of 124 °C (397 K), TRAPPIST-1b may have had a runaway greenhouse effect similar to that of Venus;[75] JWST observations indicate that it has either no atmosphere at all or one nearly devoid of CO2.[212] Based on several climate models, the planet would have been desiccated by TRAPPIST-1's stellar wind and radiation;[213][214] it could be quickly losing hydrogen and therefore any hydrogen-dominated atmosphere.[am] Water, if any exists, could persist only in specific settings on the planet,[216] whose surface temperature could be as high as 1,200 °C (1,470 K), making TRAPPIST-1b a candidate magma ocean planet.[217] According to JWST observations, the planet has an albedo of about zero.[218]
TRAPPIST-1c
[edit]
TRAPPIST-1c has a semi-major axis of 0.0158 AU (2,360,000 km)[208] and orbits its star every 2.42 Earth days. It is close enough to TRAPPIST-1 to be tidally locked.[209] JWST observations have ruled out the existence of CO2-rich atmospheres,[219] Venus-like atmospheres, but water vapour- or oxygen-rich atmospheres or no-atmosphere scenarios are possible.[220] These data imply that relative to Earth or Venus, TRAPPIST-1 c has a lower carbon content.[221] TRAPPIST-1c is outside the habitable zone[209] as it receives about twice as much stellar irradiation as Earth[222] and thus either is or has been a runaway greenhouse.[75] Based on several climate models, the planet would have been desiccated by TRAPPIST-1's stellar wind and radiation.[213] TRAPPIST-1c could harbour water only in specific settings on its surface.[216] Observations in 2017 showed no escaping hydrogen,[58] but observations by the Hubble Space Telescope (HST) in 2020 indicated that hydrogen may be escaping at a rate of 1.4×107 g/s.[215]
TRAPPIST-1d
[edit]TRAPPIST-1d has a semi-major axis of 0.022 AU (3,300,000 km) and an orbital period of 4.05 Earth days. It is more massive but less dense than Mars.[223] Based on fluid dynamical arguments, TRAPPIST-1d is expected to have weak temperature gradients on its surface if it is tidally locked,[224] and may have significantly different stratospheric dynamics than that of Earth.[225] Several climate models suggest that the planet may[213] or may not have been desiccated by TRAPPIST-1's stellar wind and radiation;[213] density estimates, if confirmed, indicate it is not dense enough to consist solely of rock.[211] The current state of TRAPPIST-1d depends on its rotation and climatic factors like cloud feedback;[an][227] it is close to the inner edge of the habitable zone, but the existence of either liquid water or alternatively a runaway greenhouse effect (that would render it uninhabitable) are dependent on detailed atmospheric conditions.[228] Water could persist in specific settings on the planet.[216]
TRAPPIST-1e
[edit]TRAPPIST-1e has a semi-major axis of 0.029 AU (4,300,000 km)[208] and orbits its star every 6.10 Earth days.[229] It has density similar that of Earth.[230] Based on several climate models, the planet is the most likely of the system to have retained its water,[213] and the most likely to have liquid water for many climate states. A dedicated climate model project called TRAPPIST-1 Habitable Atmosphere Intercomparison (THAI) has been launched to study its potential climate states.[231] Based on observations of its Lyman-alpha radiation emissions, TRAPPIST-1e may be losing hydrogen at a rate of 0.6×107 g/s.[215]
TRAPPIST-1e is in a comparable position within the habitable zone to that of Proxima Centauri b,[ao][233][234] which also has an Earth-like density.[230] TRAPPIST-1e could have retained masses of water equivalent to several of Earth's oceans.[75] Moderate quantities of carbon dioxide could warm TRAPPIST-1e to temperatures suitable for the presence of liquid water.[214]
TRAPPIST-1f
[edit]TRAPPIST-1f has a semi-major axis of 0.038 AU (5,700,000 km)[208] and orbits its star every 9.21 Earth days.[229] It is likely too distant from its host star to sustain liquid water, being instead an entirely glaciated snowball planet[213] that might host a subsurface ocean.[235] Moderate quantities of CO2 could warm TRAPPIST-1f to temperatures suitable for the presence of liquid water.[216] Lakes or ponds with liquid water might form in places where tidal heating is concentrated.[236] TRAPPIST-1f may have retained masses of water equivalent to several of Earth's oceans[75] and which could comprise up to half of the planet's mass;[237] it could thus be an ocean planet.[ap][239]
TRAPPIST-1g
[edit]TRAPPIST-1g has a semi-major axis of 0.047 AU (7,000,000 km)[208] and orbits its star every 12.4 Earth days.[229] It is likely too distant from its host star to sustain liquid water, being instead a snowball planet[213] that might host a subsurface ocean.[235] Moderate quantities of CO2[216] or internal heat from radioactive decay and tidal heating may warm its surface to above the melting point of water.[240][115] TRAPPIST-1g may have retained masses of water equivalent to several of Earth's oceans;[75] density estimates of the planet, if confirmed, indicate it is not dense enough to consist solely of rock.[211] Up to half of its mass may be water.[237]
TRAPPIST-1h
[edit]TRAPPIST-1h has a semi-major axis of 0.062 astronomical units (9,300,000 km); it is the system's least massive known planet[208] and orbits its star every 18.9 Earth days.[229] It is likely too distant from its host star to sustain liquid water and may be a snowball planet,[213][115] or have a methane/nitrogen atmosphere resembling that of Titan.[241] It might host a subsurface ocean.[235] Large quantities of CO2, hydrogen or methane,[242] or internal heat from radioactive decay and tidal heating,[240] would be needed to warm TRAPPIST-1h to the point where liquid water could exist.[242] TRAPPIST-1h could have retained masses of water equivalent to several of Earth's oceans.[75]
Data table
[edit]Planet | Mass (ME) | Semi-major axis | Orbital period (days) | Orbital eccentricity[87] | Orbital inclination[86] | Radius (R🜨) | Radiant flux[86] | Temperature [87] | Surface gravity (g)[86] | ORb [aq] | ORi [ar] |
---|---|---|---|---|---|---|---|---|---|---|---|
b | 1.374 ±0.069 | 0.01154 ±0.0001 | 1.510826 ±0.000006 | 0.00622 ±0.00304 | 89.728 ±0.165° | 1.116 +0.014 −0.012 | 4.153 ±0.160 | 397.6±3.8K (124.5 ± 3.8 °C; 256.0 ± 6.8 °F)[as] | 1.102 ±0.052 | — | — |
c | 1.308 ±0.056 | 0.01580 ±0.00013 | 2.421937 ±0.000018 | 0.00654 ±0.00188 | 89.778 ±0.118° | 1.097 +0.014 −0.012 | 2.214 ±0.085 | 339.7±3.3K (66.6 ± 3.3 °C; 151.8 ± 5.9 °F) | 1.086 ±0.043 | 5:8 | 5:8 |
d | 0.388 ±0.012 | 0.02227 ±0.00019 | 4.049219 ±0.000026 | 0.00837 ±0.00093 | 89.896 ±0.077° | 0.770 +0.011 −0.010 | 1.115 ±0.04 | 286.2±2.8K (13.1 ± 2.8 °C; 55.5 ± 5.0 °F) | 0.624 ±0.019 | 3:8 | 3:5 |
e | 0.692 ±0.022 | 0.02925 ±0.00025 | 6.101013 ±0.000035 | 0.00510 ±0.00058 | 89.793 ±0.048° | 0.920 +0.013 −0.012 | 0.646 ±0.025 | 249.7±2.4K (−23.5 ± 2.4 °C; −10.2 ± 4.3 °F) | 0.817 ±0.024 | 1:4 | 2:3 |
f | 1.039 ±0.031 | 0.03849 ±0.00033 | 9.207540 ±0.000032 | 0.01007 ±0.00068 | 89.740 ±0.019° | 1.045 +0.013 −0.012 | 0.373 ±0.014 | 217.7±2.1K (−55.5 ± 2.1 °C; −67.8 ± 3.8 °F) | 0.951 ±0.024 | 1:6 | 2:3 |
g | 1.321 ±0.038 | 0.04683 ±0.0004 | 12.352446 ±0.000054 | 0.00208 ±0.00058 | 89.742 ±0.012° | 1.129 +0.015 −0.013 | 0.252 ±0.010 | 197.3±1.9K (−75.8 ± 1.9 °C; −104.5 ± 3.4 °F) | 1.035 ±0.026 | 1:8 | 3:4 |
h | 0.326 ±0.020 | 0.06189 ±0.00053 | 18.772866 ±0.000214 | 0.00567 ±0.00121 | 89.805 ±0.013° | 0.775 +0.014 −0.014 | 0.144 ±0.006 | 171.7±1.7K (−101.5 ± 1.7 °C; −150.6 ± 3.1 °F) | 0.570 ±0.038 | 1:12 | 2:3 |
Potential planetary atmospheres
[edit]
As of 2023[update], the existence of an atmosphere around TRAPPIST-1b has been ruled out by James Webb Space Telescope observations, and there is no evidence for the other planets in the system,[at][245] but atmospheres are not ruled out[219][au] and could be detected in the future.[247] The outer planets are more likely to have atmospheres than the inner planets.[187] Several studies have simulated how different atmospheric scenarios would look to observers, and the chemical processes underpinning these atmospheric compositions.[248] The visibility of an exoplanet and of its atmosphere scale with the inverse square of the radius of its host star.[247]Detection of individual components of the atmospheres – in particular CO2, ozone, and water[249] – would also be possible, although different components would require different conditions and different numbers of transits.[250] A contamination of the atmospheric signals through patterns in the stellar photosphere is a further impediment to detection.[251][252]
The existence of atmospheres around TRAPPIST-1's planets depends on the balance between the amount of atmosphere initially present, its rate of evaporation, and the rate at which it is built back up by meteorite impacts[av],[90] incoming material from a protoplanetary disk[aw],[255] and outgassing and volcanic activity.[256] Impact events may be particularly important in the outer planets because they can both add and remove volatiles; addition is likely dominant in the outermost planets where impact velocities are slower.[257][258] The formation conditions of the planets would give them large initial quantities of volatile materials,[187] including oceans over 100 times larger than those of Earth.[259]
If the planets are tidally locked to TRAPPIST-1, surfaces that permanently face away from the star can cool sufficiently for any atmosphere to freeze out on the night side.[260] This frozen-out atmosphere could be recycled through glacier-like flows to the day side with assistance from tidal or geothermal heating from below, or could be stirred by impact events. These processes could allow an atmosphere to persist.[261] In a carbon dioxide (CO2) atmosphere, carbon-dioxide ice is denser than water ice, under which it tends to be buried. CO2-water compounds named clathrates[ax] can form. Further complications are a potential runaway feedback loop between melting ice and evaporation, and the greenhouse effect.[263]
Numerical modelling and observations constrain the properties of hypothetical atmospheres around TRAPPIST-1 planets:[187]
- Theoretical calculations[264] and observations have ruled out the possibility the TRAPPIST-1 planets have hydrogen-rich[239][265] or helium-rich atmospheres.[266] Hydrogen-rich exospheres[ay] may be detectable[268] but have not been reliably detected,[269] except perhaps for TRAPPIST-1b and 1c by Bourrier et al. (2017).[200][14]
- Water-dominated atmospheres, though suggested by some density estimates, are improbable for the planets because they are expected to be unstable under the conditions around TRAPPIST-1, especially early in the star's life.[211] The spectral properties of the planets imply they do not have a cloud-free, water-rich atmosphere.[270]
- Oxygen-dominated atmospheres can form when radiation splits water into hydrogen and oxygen, and the hydrogen escapes due to its lighter mass. The existence of such an atmosphere and its mass depends on the initial water mass, on whether the oxygen is dragged out of the atmosphere by escaping hydrogen and of the state of the planet's surface; a partially molten surface could absorb sufficient quantities of oxygen to remove an atmosphere.[271][272]
- Atmospheres formed by ammonia and/or methane near TRAPPIST-1 would be destroyed by the star's radiation at a sufficient rate to quickly remove an atmosphere. The rate at which ammonia or methane are produced, possibly by organisms, would have to be considerably larger than that on Earth to sustain such an atmosphere. It is possible the development of organic hazes from ammonia or methane photolysis could shield the remaining molecules from degradation caused by radiation.[273] Ducrot et al. (2020) interpreted observational data as implying methane-dominated atmospheres are unlikely around TRAPPIST-1 planets.[274]
- Nitrogen-dominated atmospheres are particularly unstable with respect to atmospheric escape, especially on the innermost planets, although the presence of CO2 may slow evaporation.[275] Unless the TRAPPIST-1 planets initially contained far more nitrogen than Earth, they are unlikely to have retained such atmospheres.[276]
- CO2-dominated atmospheres escape slowly because CO2 effectively radiates away energy and thus does not readily reach escape velocity; on a synchronously rotating planet, however, CO2 can freeze out on the night side, especially if there are no other gases in the atmosphere. The decomposition of CO2 caused by radiation could yield substantial amounts of oxygen, carbon monoxide (CO),[214] and ozone.[277]
Theoretical modelling by Krissansen-Totton and Fortney (2022) suggests the inner planets most likely have oxygen-and-CO2-rich atmospheres, if any.[278] If the planets have an atmosphere, the amount of precipitation, its form and location would be determined by the presence and position of mountains and oceans, and the rotation period.[279] Planets in the habitable zone are expected to have an atmospheric circulation regime resembling Earth's tropical regions with largely uniform temperatures.[280] Whether greenhouse gases can accumulate on the outer TRAPPIST-1 planets in sufficient quantities to warm them to the melting point of water is controversial; on a synchronously rotating planet, CO2 could freeze and precipitate on the night side, and ammonia and methane would be destroyed by XUV radiation from TRAPPIST-1.[75] Carbon dioxide freezing-out can occur only on the outermost planets unless special conditions are met, and other volatiles do not freeze out.[281]
Stability
[edit]
The emission of extreme ultraviolet (XUV) radiation by a star has an important influence on the stability of its planets' atmospheres, their composition and the habitability of their surfaces.[281] It can cause the ongoing removal of atmospheres from planets.[90] XUV radiation-induced atmospheric escape has been observed on gas giants.[282] M dwarfs emit large amounts of XUV radiation;[281] TRAPPIST-1 and the Sun emit about the same amount of XUV radiation[az] and because TRAPPIST-1's planets are much closer to the star than the Sun's, they receive much more intense irradiation.[55] TRAPPIST-1 has been emitting radiation for much longer than the Sun.[284] The process of atmospheric escape has been modelled mainly in the context of hydrogen-rich atmospheres and little quantitative research has been done on those of other compositions such as water and CO2.[265]
TRAPPIST-1 has moderate to high stellar activity[ba],[32] and this may be another difficulty for the persistence of atmospheres and water on the planets:[27]
- Dwarfs of the spectral class M have intense flares;[281] TRAPPIST-1 averages about 0.38 flares per day[75] and four to six superflares[bb] per year.[287] Such flares would have only small impacts on atmospheric temperatures but would substantially affect the stability and chemistry of atmospheres.[90] According to Samara, Patsourakos and Georgoulis (2021), the TRAPPIST-1 planets are unlikely to be able to retain atmospheres against coronal mass ejections.[288]
- The stellar wind from TRAPPIST-1 may have a pressure 1,000 times larger than that of the Sun at Earth's orbit, which could destabilise atmospheres of the star's planets[289] up to planet f. The pressure would push the wind deep into the atmospheres,[213] facilitating loss of water and evaporation of the atmospheres.[90][241] Stellar wind-driven escape in the Solar System is largely independent from planetary properties such as mass,[290] scaling instead with the stellar wind mass flux impacting the planet.[291] Stellar wind from TRAPPIST-1 could remove the atmospheres of its planets on a timescale of 100 million to 10 billion years.[292]
- Ohmic heating[bc] of the atmosphere of TRAPPIST-1e, f, and g amounts to 5–15 times the heating from XUV radiation; if the heat is effectively absorbed, it could destabilise the atmospheres.[294]
The star's history also influences the atmospheres of its planets.[295] Immediately after its formation, TRAPPIST-1 would have been in a pre-main-sequence state, which may have lasted between hundreds of millions[281] and two billion years.[251] While in this state, it would have been considerably brighter than it is today and the star's intense irradiation would have impacted the atmospheres of surrounding planets, vaporising all common volatiles such as ammonia, CO2, sulfur dioxide, and water.[296] Thus, all of the system's planets would have been heated to a runaway greenhouse[bd] for at least part of their existence.[281] The XUV radiation would have been even higher during the pre-main-sequence stage.[90]
Possible life
[edit]Life may be possible in the TRAPPIST-1 system, and some of the star's planets are considered promising targets for its detection.[27] On the basis of atmospheric stability, TRAPPIST-1e is theoretically the planet most likely to harbour life; the probability that it does is considerably less than that of Earth. There are an array of factors at play:[297][298]
- Due to multiple interactions, TRAPPIST-1 planets are expected to have intense tides.[299] If oceans are present,[be] the tides could: lead to alternate flooding and drying of coastal landscapes triggering chemical reactions conducive to the development of life;[301] favour the evolution of biological rhythms such as the day-night cycle that otherwise would not develop in a synchronously rotating planet;[302] mix oceans, thus supplying and redistributing nutrients;[303] and stimulate periodic expansions of marine organisms similar to red tides on Earth.[304]
- TRAPPIST-1 may not produce sufficient quantities of radiation for photosynthesis to support an Earth-like biosphere.[305][306][307] Mullan and Bais (2018) speculated that radiation from flares may increase the photosynthetic potential of TRAPPIST-1,[308] but according to Lingam and Loeb (2019), the potential would still be small.[309]
- Due to the proximity of the TRAPPIST-1 planets, it is possible rock-encased microorganisms ripped[bf] from one planet may arrive at another planet while still viable inside the rock, allowing life to spread between the planets if it originates on one.[310]
- Too much UV radiation from a star can sterilise the surface of a planet[112][157] but too little may not allow the formation of chemical compounds that give rise to life.[14][311] Inadequate production of hydroxyl radicals by low stellar-UV emission may allow gases such as carbon monoxide that are toxic to higher life to accumulate in the planets' atmospheres.[312] The possibilities range from UV fluxes from TRAPPIST-1 being unlikely to be much larger than these of early Earth – even in the event that TRAPPIST-1's emissions of UV radiation are high[313] – to being sufficient to sterilise the planets if they do not have protective atmospheres.[314] As of 2020[update] it is unclear which effect would predominate around TRAPPIST-1,[251] although observations with the Kepler Space Telescope and the Evryscope telescopes indicate the UV flux may be insufficient for the formation of life or its sterilisation. [287]
- The outer planets in the TRAPPIST-1 system could host subsurface oceans similar to those of Enceladus and Europa in the Solar System.[315][115] Chemolithotrophy, the growth of organisms based on non-organic reduced compounds,[316] could sustain life in such oceans.[144] Very deep oceans may be inimical to the development of life.[317]
- Some planets of the TRAPPIST-1 system may have enough water to completely submerge their surfaces.[318] If so, this would have important effects on the possibility of life developing on the planets, and on their climates,[319] as weathering would decrease, starving the oceans of nutrients like phosphorus as well as potentially leading to the accumulation of carbon dioxide in their atmospheres.[320]
In 2017, a search for technosignatures that would indicate the existence of past or present technology in the TRAPPIST-1 system found only signals coming from Earth.[321] In less than two millennia, Earth will be transiting in front of the Sun from the viewpoint of TRAPPIST-1, making the detection of life on Earth from TRAPPIST-1 possible.[322]
Reception and scientific importance
[edit]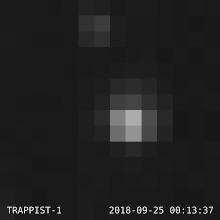
Public reaction and cultural impact
[edit]
The discovery of the TRAPPIST-1 planets drew widespread attention in major world newspapers, social media, streaming television and websites.[323][324] As of 2017[update], the discovery of TRAPPIST-1 led to the largest single-day web traffic to the NASA website.[325] NASA started a public campaign on Twitter to find names for the planets, which drew responses of varying seriousness, although the names of the planets will be decided by the International Astronomical Union.[326] The dynamics of the TRAPPIST-1 planetary system have been represented as music, such as Tim Pyle's Trappist Transits,[327] in Isolation's single Trappist-1 (A Space Anthem)[328] and Leah Asher's piano work TRAPPIST-1.[329] The alleged discovery of an SOS signal from TRAPPIST-1 was an April Fools prank by researchers at the High Energy Stereoscopic System in Namibia.[330] In 2018, Aldo Spadon created a giclée (digital artwork) named "TRAPPIST-1 Planetary System as seen from Space".[331] A website was dedicated to the TRAPPIST-1 system.[332]
Exoplanets are often featured in science-fiction works; books, comics and video games have featured the TRAPPIST-1 system, the earliest being The Terminator, a short story by Swiss author Laurence Suhner published in the academic journal that announced the system's discovery.[333] At least one conference was organised to recognise works of fiction featuring TRAPPIST-1.[334] The planets have been used as the basis of science education competitions[335] and school projects.[336][337] Websites offering TRAPPIST-1-like planets as settings of virtual reality simulations exist,[338] such as the "Exoplanet Travel Bureau"[339] and the "Exoplanets Excursion" – both by NASA.[340] Scientific accuracy has been a point of discussion for such cultural depictions of TRAPPIST-1 planets.[341]
Scientific importance
[edit]TRAPPIST-1 has drawn intense scientific interest.[342] Its planets are the most easily studied exoplanets within their star's habitable zone owing to their relative closeness, the small size of their host star, and because from Earth's perspective they frequently pass in front of their host star.[33] Future observations with space-based observatories and ground-based facilities may allow further insights into their properties such as density, atmospheres, and biosignatures.[bg] TRAPPIST-1 planets[344][345] are considered an important observation target for the James Webb Space Telescope[bh][342] and other telescopes under construction;[163] JWST began investigating the TRAPPIST-1 planets in 2023.[245] Together with the discovery of Proxima Centauri b, the discovery of the TRAPPIST-1 planets and the fact that three of the planets are within the habitable zone has led to an increase in studies on planetary habitability.[348] The planets are considered prototypical for the research on habitability of M dwarfs.[349] The star has been the subject of detailed studies[105] of its various aspects[350] including the possible effects of vegetation on its planets; the possibility of detecting oceans on its planets using starlight reflected off their surfaces;[351] possible efforts to terraform its planets;[352] and difficulties any inhabitants of the planets would encounter with discovering the law of gravitation[353] and with interstellar travel.[354]
The role EU funding played in the discovery of TRAPPIST-1 has been cited as an example of the importance of EU projects,[35] and the involvement of a Moroccan observatory as an indication of the Arab world's role in science. The original discoverers were affiliated with universities spanning Africa, Europe, and North America,[355] and the discovery of TRAPPIST-1 is considered to be an example of the importance of co-operation between observatories.[356] It is also one of the major astronomical discoveries from Chilean observatories.[357]
Exploration
[edit]TRAPPIST-1 is too distant from Earth to be reached by humans with current or expected technology.[358] Проектам космических кораблей с использованием современных ракет и гравитационных средств потребуются сотни тысячелетий, чтобы достичь TRAPPIST-1; даже теоретическому межзвездному зонду, путешествующему со скоростью света, потребуются десятилетия, чтобы достичь звезды. Спекулятивное предложение Breakthrough Starshot по отправке небольших беспилотных зондов с лазерным ускорением потребует около двух столетий, чтобы достичь TRAPPIST-1. [359]
См. также
[ редактировать ]- HD 10180 , звезда, имеющая как минимум шесть известных планет и еще три кандидата в экзопланеты.
- HD 110067 , звезда с шестью известными планетами, вращающимися в ритмическом резонансе.
- LHS 1140 , еще одна звезда с планетной системой, подходящей для изучения атмосферы.
- Список потенциально обитаемых экзопланет
- LP 890-9 , еще одна крутая звезда с планетной системой.
- Звезда Табби , еще одна звезда с заметными данными о транзите.
Примечания
[ редактировать ]- ^ Логарифм ( g ) 2,992 для Земли указывает на то, что TRAPPIST-1 имеет поверхностную гравитацию примерно в 177 раз сильнее, чем земная.
- ↑ Внутреннее название звезды, используемое проектом SPECULOOS , поскольку эта планетная система была его первым открытием.
- ^ Красный карлик — очень маленькая и холодная звезда. Это самый распространенный тип звезд в Млечном Пути . [15]
- ^ TRAPPIST — 60-сантиметровый (24 дюйма) телескоп. [11] задуман как прототип проекта «Поиск обитаемых планет, затмевающих ультрахолодные звезды» (SPECULOOS), цель которого - идентифицировать планеты вокруг близких холодных звезд. [20] [21] TRAPPIST используется для поиска экзопланет и преимущественно используется для звезд с температурой ниже 3000 К (2730 °C; 4940 °F). [22]
- ^ Когда планета движется перед своей звездой, она поглощает часть излучения звезды, которое можно наблюдать в телескопы. [29]
- ^ Небесный экватор — это проекция экватора на небо. [36]
- ^ На основе параллакса ; измерений [1] параллакс — это положение небесного объекта относительно других небесных объектов для данного положения Земли. Его можно использовать для определения расстояния объекта от Земли. [39]
- ^ Движение звезды по небу относительно звезд на заднем плане. [40]
- ^ Красные карлики включают спектральные классы M и K. [42] Спектральные типы используются для классификации звезд по их температуре. [43]
- ^ Эффективная температура — это температура, которую имело бы черное тело , излучающее такое же количество радиации. [49]
- ^ Фотосфера — это тонкий слой на поверхности звезды, где производится большая часть ее света. [51]
- ^ Солнечный цикл — это 11-летний период Солнца, в течение которого солнечная активность изменяется примерно на 0,1%. [53]
- ^ Включая Лайман-альфа-излучение. [59]
- ↑ Главная последовательность — это самая длинная стадия жизни звезды, когда она синтезирует водород . [67]
- ^ Факелы — яркие пятна на фотосфере. [69]
- ^ Вспышки — это предположительно магнитные явления, длящиеся несколько минут или часов, в течение которых части звезды излучают больше радиации, чем обычно. [69] В случае TRAPPIST-1 температуры вспышек достигают не более 9000 К (8730 °C; 15740 °F). [73]
- ^ Для сравнения: сильный магнит на холодильник имеет силу около 100 Гс, а магнитное поле Земли — около 0,5 Гс. [76]
- ^ Хромосфера — внешний слой звезды. [69]
- ^ Корональный выброс массы — это извержение коронального материала за пределы звезды. [69] [78]
- ^ Экзопланеты названы в порядке открытия как «b», «c» и так далее; если одновременно обнаружено несколько планет, они называются в порядке возрастания орбитального периода. [83] Термин «TRAPPIST-1a» используется для обозначения самой звезды. [84]
- ^ Одна астрономическая единица (АЕ) — это среднее расстояние между Землей и Солнцем. [88]
- ^ Для сравнения: орбита Земли вокруг Солнца наклонена примерно на 1,578 градуса. [95]
- ^ Орбиты двух внутренних планет могут быть круговыми; остальные могли иметь небольшой эксцентриситет. [98]
- ^ Летучие вещества — это элемент или соединение с низкой температурой кипения, например аммиак, диоксид углерода, метан, азот, диоксид серы или вода. [106]
- ^ Состав мантии каменистых планет обычно приближен к силикатам магния. [110]
- ^ Резонанс Лапласа — это орбитальный резонанс, состоящий из трех тел, похожих на галилеевы спутники Европу , Ганимед и Ио вокруг Юпитера. [121]
- ^ Где планета, а не симметричная сфера, имеет разный радиус для каждой из трех основных осей. [127]
- ^ Это приводит к тому, что одна половина планеты постоянно обращена к звезде в постоянный день, а другая половина постоянно обращена от звезды в постоянную ночь. [131]
- ^ Дегазация — это выброс газов из мантии или магмы, которые в конечном итоге могут сформировать атмосферу. [135]
- ^ Криовулканизм возникает, когда пар, жидкая вода или водные жидкости извергаются на поверхность планеты, которая обычно слишком холодна, чтобы содержать жидкую воду. [141]
- ^ Гидротермальные источники — это горячие источники, которые находятся под водой и предположительно являются местами, где могла зародиться жизнь. [143]
- ^ Без учета гравитационного сжатия . [150]
- ^ То есть внутренние планеты никогда не смогут покрыть весь диск TRAPPIST-1 с точки зрения этих планет. [85]
- ^ Обитаемая зона — это область вокруг звезды, где температура не слишком высокая и не слишком низкая для существования жидкой воды; ее еще называют « зоной Златовласки ». [29] [75]
- ^ Предел Роша — это расстояние, на котором тело разрывается приливом. [175]
- ^ Радиус холма — это максимальное расстояние, на котором гравитация планеты может удерживать луну без того, чтобы гравитация звезды отрывала луну. [176]
- ^ Неустойчивость потока — это процесс, при котором взаимодействие между газом и твердыми частицами приводит к тому, что последние слипаются в нити. Эти нити могут дать начало телам-предшественникам планет. [190]
- ^ Согласно критериям Международного астрономического союза , тело должно очистить свое окружение, чтобы квалифицироваться как планета Солнечной системы. [204]
- ^ Судя по выбросам излучения Лайман-альфа , TRAPPIST-1b может терять водород со скоростью 4,6 × 10. 7 г/с . [215]
- ^ Облака на дневной стороне, отражающие звездный свет, могут охладить TRAPPIST-1d до температур, допускающих присутствие жидкой воды. [226]
- ^ Экзопланета Проксима Центавра b находится в обитаемой зоне звезды . ближайшей к Солнечной системе [232]
- ^ Океанские тела все еще можно называть таковыми, даже если они покрыты льдом. [238]
- ^ Приблизительный орбитальный резонанс с TRAPPIST-1b
- ^ Приблизительный орбитальный резонанс с внутренней планетой.
- ^ Измеренная температура поверхности 503 К (230 ° C; 446 ° F). [244]
- ^ Бурье и др. (2017) интерпретировали данные об УФ-поглощении, полученные космическим телескопом Хаббл, как предполагающие, что внешние планеты TRAPPIST-1 все еще имеют атмосферу. [14]
- ^ Компьютерное моделирование показывает, что отсутствие атмосферы вокруг TRAPPIST-1 b и c не означает отсутствия ее вокруг других планет. [246]
- ^ События удара также могут удалять атмосферу, но высокая скорость такой «ударной эрозии» подразумевает наличие массы метеоритов, несовместимой со свойствами системы TRAPPIST-1. [253]
- ^ Протопланетный диск — это диск материи, окружающий звезду. Считается, что планеты формируются в таких дисках. [254]
- ^ Клатрат — это химическое соединение, в котором одно соединение (или химический элемент), например, диоксид углерода (или ксенон), заключено в клетку, состоящую из молекул другого соединения. [262]
- ^ Экзосфера — это область атмосферы, плотность которой настолько низка, что атомы и молекулы больше не сталкиваются. Он образуется в результате выхода атмосферы , и наличие богатой водородом экзосферы предполагает наличие воды. [267]
- ^ По оценкам различных источников, TRAPPIST-1 излучает столько же, сколько Солнце в солнечном минимуме . [14] та же сумма [251] или больше, чем Солнце. [283]
- ^ Звездная активность — это возникновение изменений светимости, в основном в рентгеновских диапазонах, вызванное магнитным полем звезды. [285]
- ^ Вспышки с энергией более 1 × 10 34 эргов (1,0 × 10 27 Дж). [286]
- ^ Омический нагрев происходит, когда электрические токи, возбуждаемые звездным ветром, проходят через части атмосферы, нагревая ее. [293]
- ^ В безудержной теплице вся вода на планете находится в форме пара. [296]
- ^ Планеты, не несущие океанских территорий, также могут подвергаться приливному нагреву (или изгибу), что приводит к структурной деформации. [300]
- ^ Например, удары метеорита могут отколоть камни от планет с достаточной скоростью, чтобы они покинули гравитацию. [310]
- ^ Биосигнатуры — это свойства планеты, которые можно обнаружить издалека и которые предполагают существование жизни, например, атмосферные газы, образующиеся в результате биологических процессов. [343]
- ^ По состоянию на 2017 год. [update] они были одними из самых маленьких известных планет, на которых JWST мог обнаруживать атмосферу. [346] Вполне возможно, что у JWST не будет времени надежно обнаружить определенные биосигнатуры, такие как метан и озон. [347]
Ссылки
[ редактировать ]- ^ Перейти обратно: а б с д и ж г час Гайя EDR3 2021 .
- ^ Перейти обратно: а б с Коста и др. 2006 , с. 1240
- ^ Коста и др. 2006 , с. 1234.
- ^ Перейти обратно: а б с Кутри и др. 2003 , с. II/246.
- ^ Перейти обратно: а б с д и Агол и др. 2021 , с. 1.
- ^ Перейти обратно: а б с Дельрес и др. 2018 , стр. 3577–3597.
- ^ Жизнь и др. 2017 , с. 7.
- ^ Барнс и др. 2014 , стр. 3094–3113.
- ^ Бургассер и Мамаек 2017 , с. 7.
- ^ Мартинес-Родригес и др. 2019 , с. 3.
- ^ Перейти обратно: а б с д Турбет и др. 2020 , с. 2.
- ^ Медоуз и Шмидт 2020 , с. 727.
- ^ Дельрес и др. 2022 , с. 2.
- ^ Перейти обратно: а б с д и Харбах и др. 2021 , с. 3.
- ^ Гарго и др. 2011 , Красный Карлик.
- ^ Перейти обратно: а б Гизис и др. 2000 , с. 1088.
- ^ Перейти обратно: а б Гиллон и др. 2016 , с. 225.
- ^ Гизис и др. 2000 , с. 1085.
- ^ Гизис и др. 2000 , с. 1086.
- ^ Барстоу и Ирвин 2016 , с. 95.
- ^ Гиллон и др. 2013 , с. 1.
- ^ Шилдс, Баллард и Джонсон, 2016 , с. 7.
- ^ Голдсмит 2018 , с. 118.
- ^ Ринальди и Нуньес Феррер 2017 , с. 1.
- ^ Перейти обратно: а б Ангосто, Сарагоса и Дыня 2017 , с. 85.
- ^ Перейти обратно: а б с Ангосто, Сарагоса и Дыня 2017 , с. 86.
- ^ Перейти обратно: а б с Маров и Шевченко 2020 , с. 865.
- ^ Линский 2019 , с. 105.
- ^ Перейти обратно: а б Цисевский 2017 , с. 23.
- ^ Гиллон и др. 2017 , с. 461.
- ^ Дюкро 2021 , с. 4.
- ^ Перейти обратно: а б с д Гиллон и др. 2016 , с. 221.
- ^ Перейти обратно: а б Турбет и др. 2020 , с. 3.
- ^ Перейти обратно: а б Агол и др. 2021 , с. 2.
- ^ Перейти обратно: а б Ринальди и Нуньес Феррер, 2017 , стр. 101-1. 1–2
- ^ Гарго и др. 2011 , Небесный экватор.
- ^ Барстоу и Ирвин 2016 , с. 93.
- ^ Перейти обратно: а б Хауэлл и др. 2016 , с. 1.
- ^ Гарго и др. 2011 , Параллакс.
- ^ Гарго и др. 2011 , Собственное движение.
- ^ Хауэлл и др. 2016 , стр. 1, 4.
- ^ Энциклопедия астрономии SAO 2022 , Красный карлик.
- ^ Гарго и др. 2011 , Спектральный тип.
- ^ Клотье и Трио, 2016 , с. 4019.
- ^ Перейти обратно: а б с Линхард и др. 2020 , стр. 3790–3808.
- ^ Голдсмит 2018 , с. 82.
- ^ Фишер и Саур 2019 , с. 2.
- ^ Гиллон и др. 2020 , с. 10.
- ^ Гарго и др. 2011 , Эффективная температура.
- ^ Дельрес и др. 2022 , с. 21.
- ^ Гарго и др. 2011 , Фотосфера.
- ^ Майлз-Паес и др. 2019 , с. 38.
- ^ Гарго и др. 2011 , Изменчивость (Звездный).
- ^ Глейзер и др. 2020 , с. 2.
- ^ Перейти обратно: а б Фаббиан и др. 2017 , с. 770.
- ^ Уилсон и др. 2021 , с. 10.
- ^ Перейти обратно: а б с Уилсон и др. 2021 , с. 1.
- ^ Перейти обратно: а б Уилсон и др. 2021 , с. 2.
- ^ Пинеда и Халлинан 2018 , с. 2.
- ^ Пинеда и Халлинан 2018 , с. 7.
- ^ Рёттенбахер и Кейн, 2017 , с. 2.
- ^ Гюнтер и др. 2022 , с. 13.
- ^ Бургассер и Мамаек 2017 , с. 1.
- ^ Актон и др. 2017 , с. 32.
- ^ Перейти обратно: а б Снеллен 2017 , с. 423.
- ^ Актон и др. 2017 , с. 34.
- ^ Гарго и др. 2011 , Основная часть.
- ^ Перейти обратно: а б Моррис и др. 2018 , с. 1.
- ^ Перейти обратно: а б с д и Гарго и др. 2011 , Солнце (и Молодое Солнце).
- ^ Моррис и др. 2018 , с. 5.
- ^ Линский 2019 , с. 250.
- ^ Моррис и др. 2018 , с. 6.
- ^ Ховард и др. 2023 , с. 17.
- ^ Гиллон и др. 2020 , с. 5.
- ^ Перейти обратно: а б с д и ж г час я дж к л Айрапетян и др. 2020 , с. 159.
- ^ МагЛаб 2022 .
- ^ Кочухов 2021 , с. 28.
- ^ Перейти обратно: а б Муллан и Паудель 2019 , с. 2.
- ^ Перейти обратно: а б Сакауэ и Сибата 2021 , с. 1.
- ^ Линский 2019 , стр. 147–150.
- ^ Фишер и Саур 2019 , с. 6.
- ^ Гонсалес и др. 2019 , с. 2.
- ^ Шнайдер и др. 2011 , с. 8.
- ^ Харбах и др. 2021 , с. 2.
- ^ Перейти обратно: а б с Верас и Бридт 2017 , с. 2677.
- ^ Перейти обратно: а б с д Агол и др. 2021 , Таблицы.
- ^ Перейти обратно: а б с д Гримм и др. 2018 .
- ^ Фрайр и др. 2019 , с. 1657.
- ^ Голдсмит 2018 , с. 120.
- ^ Перейти обратно: а б с д и ж г час Турбет и др. 2020 , с. 8.
- ^ Крал и др. 2018 , с. 2650
- ^ Чайлдс, Мартин и Ливио 2022 , с. 4.
- ^ Мартин и Ливио 2022 , с. 6.
- ^ Марино и др. 2020 , с. 6071.
- ^ Справочник научных таблиц 2022 , с. 2.
- ^ Агол и др. 2021 , с. 14.
- ^ Хейсинг и др. 2021 , с. 1.
- ^ Брассер и др. 2022 , с. 2373.
- ^ Демори и др. 2020 , с. 19.
- ^ Перейти обратно: а б Мальтальяти 2017 , с. 1.
- ^ Кейн и др. 2021 , с. 1.
- ^ Шринивас 2017 , с. 17.
- ^ Мадхусудхан 2020 , с. 6-5.
- ^ Макдонаф и Йошизаки 2021 , с. 9.
- ^ Перейти обратно: а б Линский 2019 , с. 198.
- ^ Гарго и др. 2011 , Неустойчивый.
- ^ Агол и др. 2021 , с. 30
- ^ Перейти обратно: а б Гиллон и др. 2020 , с. 11.
- ^ Schlichting & Young 2022 , с. 16.
- ^ Хаким и др. 2018 , с. 3.
- ^ Хаким и др. 2018 , с. 70.
- ^ Перейти обратно: а б Барт и др. 2021 , с. 1326.
- ^ Гримм и др. 2018 , с. 8.
- ^ Лингам и Леб 2021 , с. 594.
- ^ Перейти обратно: а б с д Квик и др. 2023 .
- ^ Ван Холст, Ноак и Риволдини 2019 , стр. 598.
- ^ Линский 2019 , с. 253.
- ^ Линский 2019 , с. 254.
- ^ Ашванден и др. 2018 , с. 6.
- ^ Гримм и др. 2018 , с. 3.
- ^ Мадхусудхан 2020 , с. 11-2.
- ^ Гримм и др. 2018 , с. 2.
- ^ Дюкро 2021 , с. 5.
- ^ Медоуз и Шмидт 2020 , с. 4.
- ^ Турбет и др. 2020 , с. 13.
- ^ Винсон, Тамайо и Хансен 2019 , с. 5747.
- ^ Эльшабури и др. 2016 , с. 5.
- ^ Занацци и Лай 2017 , с. 2879.
- ^ Перейти обратно: а б Турбет и др. 2020 , стр. 12–13.
- ^ Лингам и Леб 2021 , с. 144.
- ^ Голдсмит 2018 , с. 123.
- ^ Вольф 2017 , с. 1.
- ^ Турбет и др. 2018 , с. 7.
- ^ Барр, Добос и Кисс 2018 , стр. 1–2.
- ^ Гарго и др. 2011 , Дегазация.
- ^ Кислякова и др. 2017 , с. 880.
- ^ Люгер и др. 2017 , стр. 2.
- ^ Перейти обратно: а б Квик и др. 2020 , с. 19.
- ^ Квик и др. 2023 , с. 13.
- ^ Турбет и др. 2018 , с. 8.
- ^ Квик и др. 2023 , с. 2.
- ^ Квик и др. 2023 , с. 14.
- ^ Гарго и др. 2011 , Микробиология горячих источников.
- ^ Перейти обратно: а б Кендалл и Бирн, 2020 , с. 1.
- ^ Перейти обратно: а б Кислякова и др. 2017 , с. 878.
- ^ Барр, Добос и Кисс 2018 , с. 12.
- ^ Перейти обратно: а б Турбет и др. 2020 , с. 14.
- ^ Занацци и Трио 2019 , с. 61.
- ^ Лаборатория реактивного движения 2021 .
- ^ Шринивас 2017 , с. 16.
- ^ Радноти 2021 , с. 4.
- ^ Перейти обратно: а б О'Мэлли-Джеймс и Калтенеггер 2017 , с. 27.
- ^ Перейти обратно: а б Бурье и др. 2017 , с. 7.
- ^ Шилдс и Карнс 2018 , с. 1.
- ^ Игер и др. 2020 , стр. 10.
- ^ Перейти обратно: а б с О'Мэлли-Джеймс и Калтенеггер 2017 , с. 26.
- ^ Авифан 2018 , с. 13.
- ^ Гарго и др. 2011 , Альбедо.
- ^ Перейти обратно: а б Альберт и др. 2017 , с. 6.
- ^ Барстоу и Ирвин 2016 , с. 92.
- ^ Чеклер, Мену и Эббот, 2017 , с. 9.
- ^ Перейти обратно: а б Крал и др. 2018 , с. 2649.
- ^ Рашби и др. 2020 , с. 13.
- ^ Кароне и др. 2018 , с. 4677
- ^ Ян и Цзи 2018 , с. 1.
- ^ О'Мэлли-Джеймс и Калтенеггер 2019 , стр. 4542.
- ^ Квик и др. 2023 , с. 9.
- ^ Квик и др. 2023 , с. 1.
- ^ Бурье и др. 2017 , с. 2.
- ^ Болмонт и др. 2017 , с. 3729.
- ^ Болмонт и др. 2017 , с. 3739.
- ^ Болмонт и др. 2017 , с. 3740.
- ^ Кейн 2017 , с. 4.
- ^ Гарго и др. 2011 , Рош Лимит.
- ^ Гарго и др. 2011 , Радиус холма/Сфера.
- ^ Кейн 2017 , с. 3.
- ^ Мартинес-Родригес и др. 2019 , с. 8.
- ^ Мартинес-Родригес и др. 2019 , с. 6.
- ^ Аллен, Беккер и Фьюз 2018 , стр. 1.
- ^ Фарриш и др. 2019 , с. 7.
- ^ Фарриш и др. 2019 , с. 6.
- ^ Айрапетян и др. 2020 , с. 164.
- ^ Фраскетти и др. 2019 , с. 11.
- ^ Грейвер и др. 2022 , с. 9.
- ^ Чао и др. 2021 , с. 5.
- ^ Перейти обратно: а б с д Турбет и др. 2020 , с. 36.
- ^ Перейти обратно: а б Турбет и др. 2020 , с. 9.
- ^ Чайлдс и др. 2023 , с. 3750.
- ^ Ормель, Лю и Шуненберг 2017 , с. 3.
- ^ Перейти обратно: а б Лю и Цзи 2020 , с. 24.
- ^ Огихара и др. 2022 , с. 6.
- ^ Брассер и др. 2022 , с. 2374.
- ^ Бин, Рэймонд и Оуэн, 2021 , с. 9.
- ^ Гримм и др. 2018 , с. 13.
- ^ Марино и др. 2020 , с. 6067.
- ^ Турбет и др. 2020 , стр. 9–10.
- ^ Флок и др. 2019 , с. 10.
- ^ Хейсинг и др. 2021 , с. 5.
- ^ Перейти обратно: а б Грессье и др. 2022 , с. 2.
- ^ Раймонд и др. 2021 , с. 1.
- ^ Раймонд и др. 2021 , с. 2.
- ^ Раймонд и др. 2021 , с. 3.
- ^ Перейти обратно: а б Раймонд и др. 2021 , с. 4.
- ^ Габриэль и Хорн, 2021 , с. 6.
- ^ Чайлдс и др. 2023 , с. 3762.
- ^ Криссансен-Тоттон и Фортни 2022 , с. 8.
- ^ Перейти обратно: а б с д и ж Гримм и др. 2018 , с. 6.
- ^ Перейти обратно: а б с д Гиллон и др. 2016 , с. 222.
- ^ Лим и др. 2023 , с. 2.
- ^ Перейти обратно: а б с д Турбет и др. 2020 , с. 24
- ^ Я и др. 2023 , с. 5.
- ^ Перейти обратно: а б с д и ж г час я Линский 2019 , стр. 198–199.
- ^ Перейти обратно: а б с Турбет и др. 2020 , с. 28
- ^ Перейти обратно: а б с Гренфелл и др. 2020 , с. 11.
- ^ Перейти обратно: а б с д и Турбет и др. 2020 , с. 29.
- ^ Гренфелл и др. 2020 , с. 18.
- ^ Лим и др. 2023 , с. 7.
- ^ Перейти обратно: а б Лим и др. 2023 , с. 9.
- ^ Линковски и др. 2023 , с. 8.
- ^ Тейшейра и др. 2023 , с. 12.
- ^ Агол и др. 2021 , с. 21.
- ^ Стивенсон 2019 , с. 329.
- ^ Пьерумберт и Хаммонд 2019 , с. 285.
- ^ Кароне и др. 2018 , с. 4683
- ^ Турбет и др. 2018 , с. 17.
- ^ Турбет и др. 2018 , с. 1.
- ^ Турбет и др. 2020 , стр. 5–6.
- ^ Перейти обратно: а б с д Агол и др. 2021 , с. 10.
- ^ Перейти обратно: а б Стивенсон 2019 , с. 327.
- ^ Турбет и др. 2020 , стр. 29–30.
- ^ Медоуз и др. 2018 , с. 133.
- ^ Янич 2017 , с. 61.
- ^ Медоуз и др. 2018 , с. 141.
- ^ Перейти обратно: а б с Квик и др. 2023 , с. 4.
- ^ Квик и др. 2023 , с. 11.
- ^ Перейти обратно: а б Кейн и др. 2021 , с. 16.
- ^ Кейн и др. 2021 , с. 14.
- ^ Перейти обратно: а б Кейн и др. 2021 , с. 17.
- ^ Перейти обратно: а б Айрапетян и др. 2020 , с. 171.
- ^ Перейти обратно: а б Турбет и др. 2018 , с. 2.
- ^ Перейти обратно: а б Турбет и др. 2020 , с. 30
- ^ Агол и др. 2021 .
- ^ Грин и др. 2023 .
- ^ Перейти обратно: а б Я и др. 2023 , с. 1.
- ^ Тейшейра и др. 2023 , стр. 8, 9.
- ^ Перейти обратно: а б Фортни 2018 , с. 17.
- ^ Вундерлих и др. 2020 , стр. 26–27.
- ^ Чжан и др. 2018 , с. 1.
- ^ Турбет и др. 2020 , с. 33.
- ^ Перейти обратно: а б с д Дюкро и др. 2020 , с. 2.
- ^ Ховард и др. 2023 , с. 2.
- ^ Тейшейра и др. 2023 , с. 10.
- ^ Гарго и др. 2011 , Протопланетный диск.
- ^ Крал, Даву и Шарне, 2020 , с. 770.
- ^ Хори и Огихара 2020 , с. 1.
- ^ Турбет и др. 2020 , с. 10.
- ^ Крал и др. 2018 , с. 2670.
- ^ Лингам и Леб 2019a , с. 8.
- ^ Турбет и др. 2018 , с. 9.
- ^ Турбет и др. 2018 , с. 10.
- ^ Турбет и др. 2018 , с. 14.
- ^ Турбет и др. 2018 , стр. 14–15.
- ^ Турбет и др. 2020 , с. 23.
- ^ Перейти обратно: а б Гиллон и др. 2020 , с. 14.
- ^ Грессье и др. 2022 , с. 6.
- ^ дос Сантос и др. 2019 , с. 1.
- ^ дос Сантос и др. 2019 , с. 11.
- ^ Гиллон и др. 2020 , с. 15.
- ^ Эдвардс и др. 2020 , с. 11.
- ^ Турбет и др. 2020 , стр. 24–26.
- ^ Тейшейра и др. 2023 , с. 2.
- ^ Турбет и др. 2020 , стр. 26–27.
- ^ Дюкро и др. 2020 , с. 19.
- ^ Турбет и др. 2020 , стр. 27–28.
- ^ Турбет и др. 2020 , с. 37.
- ^ Вундерлих и др. 2020 , с. 2.
- ^ Криссансен-Тоттон и Фортни 2022 , с. 14.
- ^ Стивенсон 2019 , стр. 330–332.
- ^ Чжан 2020 , с. 57.
- ^ Перейти обратно: а б с д и ж Турбет и др. 2020 , с. 6.
- ^ Уитли и др. 2017 , с. 74.
- ^ Турбет и др. 2020 , стр. 7–8.
- ^ Актон и др. 2017 , с. 33.
- ^ Гарго и др. 2011 , Активность (Магнитная).
- ^ Глейзер и др. 2020 , с. 1.
- ^ Перейти обратно: а б Глейзер и др. 2020 , с. 9.
- ^ Самара, Пацуракос и Георгулис 2021 , с. 1.
- ^ Линский 2019 , с. 191.
- ^ Донг и др. 2018 , с. 262.
- ^ Тейшейра и др. 2023 , с. 5.
- ^ Донг и др. 2018 , с. 264.
- ^ Коэн и др. 2018 , с. 1.
- ^ Линский 2019 , с. 189.
- ^ Турбет и др. 2020 , стр. 3, 5
- ^ Перейти обратно: а б Турбет и др. 2020 , с. 5.
- ^ Лингам и Леб 2018a , с. 122.
- ^ Пидгородецкая и др. 2020 , с. 2.
- ^ Лингам и Леб 2018b , с. 973.
- ^ Барр, Добос и Кисс 2018 , с. 6.
- ^ Лингам и Леб 2018b , стр. 969–970.
- ^ Лингам и Леб 2018b , с. 971.
- ^ Лингам и Леб 2018b , с. 972.
- ^ Лингам и Леб 2018b , с. 975.
- ^ Лингам и Леб 2019a , с. 11.
- ^ Ковон и др. 2021 , стр. 3332.
- ^ Лингам и Леб 2021 , с. 347.
- ^ Муллан и Бэйс 2018 , с. 11.
- ^ Лингам и Леб 2019b , с. 5926.
- ^ Перейти обратно: а б Голдсмит 2018 , с. 124.
- ^ Ранджан, Вордсворт и Саселов, 2017 , стр. 2, 9.
- ^ Швитерман и др. 2019 , с. 5.
- ^ О'Мэлли-Джеймс и Калтенеггер 2017 , с. 30.
- ^ Валио и др. 2018 , с. 179.
- ^ Лингам и Леб 2019c , с. 112.
- ^ Гарго и др. 2011 , Хемолитотроф.
- ^ Барт и др. 2021 , с. 1344.
- ^ Guimond, Rudge & Shorttle 2022 , стр. 16–17.
- ^ Гимонд, Радж и Шорттл, 2022 , с. 1.
- ^ Глейзер и др. 2020 , стр. 7.
- ^ Пинчук и др. 2019 , с. 1.
- ^ Кальтенеггер и Фахерти, 2021 , с. 505.
- ^ Short & Stapelfeldt 2017 , стр. 1, 28.
- ^ Диас 2017 , стр. 185–186.
- ^ Short & Stapelfeldt 2017 , с. 28.
- ^ Мир физики 2017 , с. 1.
- ^ Рибер 2018 , стр. 1.
- ^ Хауэлл 2020 , с. 3–34.
- ^ Маккей 2021 , с. 14.
- ^ Янич 2017 , с. 57.
- ^ Канас 2019 , с. 488.
- ^ Гибб 2022 , с. 2.
- ^ Гиллон 2020a , с. 35.
- ^ Гиллон 2020b , с. 50.
- ^ Сейн и др. 2021 , с. 3.
- ^ Хьюз 2022 , с. 148.
- ^ Лейн и др. 2022 , с. 5.
- ^ Паладини 2019 , стр. 239, 254.
- ^ Бюро путешествий Экзопланеты 2021 .
- ^ AAS 2020 , с. 309.
- ^ Фидрик и др. 2020 , стр. 1–2.
- ^ Перейти обратно: а б Деминг и Кнутсон, 2020 , с. 459.
- ^ Гренфелл 2017 , с. 2.
- ^ Мадхусудхан 2019 , с. 652.
- ^ Турбет и др. 2020 , с. 31.
- ^ Морли и др. 2017 , с. 1.
- ^ Цзяо 2019 , с. 880.
- ^ Лингам и Леб 2018a , с. 116.
- ^ Мадхусудхан 2020 , с. Я-7.
- ^ Дельрес и др. 2022 , с. 32.
- ^ Коппарла и др. 2018 , стр. 1.
- ^ Sleator & Smith 2017 , стр. 1–2.
- ^ Деньги 2022 , с. 10.
- ^ Лингам и Леб 2018c .
- ^ Детерманн 2019 , стр. 168–169.
- ^ Гутьеррес и др. 2019 , с. 41.
- ^ Гуриди, Пертузе и Пфотенхауэр 2020 , стр. 5.
- ^ Еврошкола 2018 , с. 10.
- ^ Шринивас 2017 , с. 19.
Источники
[ редактировать ]- «Специальная сессия 001» (PDF) . Тезисы 235-го заседания AAS . Гонолулу, Гавайи : Американское астрономическое общество. Январь 2020.
- Актон, К.; Славней, С.; Арвидсон, Р.Э.; Гэддис, ЛР; Гордон, М.; Лавуа, С. (2017). «Планетарная система данных» (PDF) . Лунная планета. Инф. Бык . 150 : 2–11.
- Агол, Эрик; Дорн, Кэролайн; Гримм, Саймон Л.; Тюрбет, Мартин; Дюкро, Эльза; Дельрес, Летиция; Гиллон, Майкл; Демори, Брис-Оливье; Бурданов Артем; Баркауи, Халид; Бенхалдун, Зухайр; Болмонт, Эмелин; Бургассер, Адам; Кэри, Шон; де Вит, Жюльен; Фабрики, Дэниел; Форман-Макки, Дэниел; Хальдеманн, Йонас; Эрнандес, Дэвид М.; Ингаллс, Джеймс; Жехин, Эммануэль; Лэнгфорд, Закари; Леконт, Жереми; Ледерер, Сьюзен М.; Люгер, Родриго; Малхотра, Рену; Медоуз, Виктория С.; Моррис, Бретт М.; Посуэлос, Франсиско Дж.; Кело, Дидье; Раймонд, Шон Н.; Селсис, Франк; Шестович, Марко; Трио, Амори HMJ; Ван Гроотель, Валери (1 февраля 2021 г.). «Уточнение времени прохождения и фотометрического анализа TRAPPIST-1: массы, радиусы, плотности, динамика и эфемериды» . Планетарный научный журнал . 2 (1): 1. arXiv : 2010.01074 . Бибкод : 2021PSJ.....2....1A . дои : 10.3847/psj/abd022 . ISSN 2632-3338 . S2CID 222125312 .
- Айрапетян, В.С.; Барнс, Р.; Коэн, О.; Коллинсон, Джорджия; Данчи, туалет; Донг, CF; Дель Генио, AD; Франция, К.; Гарсия-Сейдж, К.; Глоцер, А.; Гопалсвами, Н.; Гренфелл, Дж.Л.; Гронов Г.; Гюдель, М.; Хербст, К.; Хеннинг, В.Г.; Джекман, Швейцария; Джин, М.; Джонстон, CP; Кальтенеггер, Л.; Кей, компакт-диск; Кобаяши, К.; Куанг, В.; Ли, Г.; Линч, Би Джей; Люфтингер, Т.; Луманн, Дж.Г.; Маэхара, Х.; Млынчак, М.Г.; Ноцу, Ю.; Остен, РА; Рамирес, РМ; Ругхаймер, С.; Шойхер, М.; Шлидер, Дж. Э.; Шибата, К.; Соуза-Сильва, К.; Стаменкович, В.; Стрейнджвей, Р.Дж.; Усманов А.В.; Вергадос, П.; Верхоглядова, ОП; Видотто, А.А.; Войтек, М.; Путь, MJ; Занк, врач общей практики; Ямасики, Ю. (апрель 2020 г.). «Влияние космической погоды на климат и обитаемость экзопланет земного типа». Международный журнал астробиологии . 19 (2): 136–194. arXiv : 1905.05093 . Бибкод : 2020IJAsB..19..136A . дои : 10.1017/S1473550419000132 . ISSN 1475-3006 . S2CID 152282234 .
- Альберти, Томмазо; Карбоне, Винченцо; Лепрети, Фабио; Веккьо, Антонио (18 июля 2017 г.). «Сравнительный климат планетарной системы Траппист-1: результаты простой модели климата и растительности» . Астрофизический журнал . 844 (1): 19. arXiv : 1706.06005 . Бибкод : 2017ApJ...844...19A . дои : 10.3847/1538-4357/aa78a2 . S2CID 118972556 .
- Аллен, Джон; Беккер, Кристофер; Фьюз, Кристофер (1 января 2018 г.). Стабильность спутников в системе Траппист-1 . Американское астрономическое общество, собрание AAS № 231. Тезисы докладов собрания Американского астрономического общества № 231 . Том. 231. Нэшнл-Харбор, Мэриленд . п. 148.21. Бибкод : 2018AAS...23114821A .
- Ангосто, Мария Каскалес; Сарагоса, Федерико Майор; Дыня, Хосе Мигель Ортис (2017). Нобелевская премия 2016 г. [Нобелевская премия 2016 г.] (PDF) (Отчет) (на испанском языке). Учебный центр Рамона Аресеса.
- Ашванден, Маркус Дж.; Шолькманн, Феликс; Бетьюн, Уильям; Шмутц, Вернер; Абраменко Валентина; Чунг, Марк CM; Мюллер, Даниэль; Бенц, Арнольд; Чернов, Геннади; Крицук Алексей Георгиевич; Скаргл, Джеффри Д.; Мелатос, Эндрю; Вагонер, Роберт В.; Тримбл, Вирджиния; Грин, Уильям Х. (март 2018 г.). «Порядок из случайности: процессы самоорганизации в астрофизике». Обзоры космической науки . 214 (2): 55. arXiv : 1708.03394 . Бибкод : 2018ССРв..214...55А . дои : 10.1007/s11214-018-0489-2 . ISSN 1572-9672 . S2CID 119064521 .
- Авифан, Супачай (2018). Экзун в структуре галактики . Спрингеровские тезисы. дои : 10.1007/978-3-319-90957-8 . ISBN 978-3-319-90956-1 .
- Барнс, младший; Дженкинс, Дж. С.; Джонс, HRA; Джефферс, СВ; Рохо, П.; Арриагада, П.; Джордан, А.; Миннити, Д.; Туоми, М.; Пинфилд, Д.; Англада-Эскуде, Г. (11 апреля 2014 г.). «Точные лучевые скорости 15 карликов М5–М9» . Ежемесячные уведомления Королевского астрономического общества . 439 (3): 3094–3113. arXiv : 1401.5350 . Бибкод : 2014MNRAS.439.3094B . дои : 10.1093/mnras/stu172 . S2CID 16005221 .
- Барр, Эми С.; Добос, Вера; Поцелуй, Ласло Л. (1 мая 2018 г.). «Внутренние структуры и приливное нагревание на планетах TRAPPIST-1». Астрономия и астрофизика . 613 : А37. arXiv : 1712.05641 . Бибкод : 2018A&A...613A..37B . дои : 10.1051/0004-6361/201731992 . ISSN 0004-6361 . S2CID 119516532 .
- Барстоу, Дж. К.; Ирвин, PGJ (1 сентября 2016 г.). «Обитаемые миры с JWST: транзитная спектроскопия системы TRAPPIST-1?» . Ежемесячные уведомления Королевского астрономического общества: письма . 461 (1): L92–L96. arXiv : 1605.07352 . Бибкод : 2016MNRAS.461L..92B . дои : 10.1093/mnrasl/slw109 . ISSN 1745-3933 . S2CID 17058804 .
- Барт, Патрик; Кароне, Людмила; Барнс, Рори; Ноак, Лена; Мольер, Поль; Хеннинг, Томас (1 ноября 2021 г.). «Эволюция магматического океана планет TRAPPIST-1». Астробиология . 21 (11): 1325–1349. arXiv : 2008.09599 . Бибкод : 2021AsBio..21.1325B . дои : 10.1089/ast.2020.2277 . ISSN 1531-1074 . ПМИД 34314604 . S2CID 221246323 .
- Бин, Джейкоб Л.; Раймонд, Шон Н.; Оуэн, Джеймс Э. (2021). «Природа и происхождение планет размером с субнептун» . Журнал геофизических исследований: Планеты . 126 (1): e2020JE006639. arXiv : 2010.11867 . Бибкод : 2021JGRE..12606639B . дои : 10.1029/2020JE006639 . ISSN 2169-9100 . ПМК 7900964 . ПМИД 33680689 .
- Болмонт, Э.; Селсис, Ф.; Оуэн, Дж. Э.; Рибас, И.; Раймонд, С.Н.; Леконт, Дж.; Гиллон, М. (21 января 2017 г.). «Потеря воды с планет земной группы, вращающихся вокруг ультрахолодных карликов: последствия для планет TRAPPIST-1» . Ежемесячные уведомления Королевского астрономического общества . 464 (3): 3728–3741. arXiv : 1605.00616 . Бибкод : 2017MNRAS.464.3728B . дои : 10.1093/mnras/stw2578 . ISSN 1365-2966 . S2CID 53687987 .
- Бурье, В.; де Вит, Дж.; Болмонт, Э.; Стаменкович, В.; Уитли, Пи Джей; Бургассер, AJ; Дельрес, Л.; Демори, Б.-О.; Эренрайх, Д.; Гиллон, М.; Джехин, Э.; Леконт, Дж.; Ледерер, С.М.; Льюис, Н.; Трио, AHMJ; Ван Гроотель, В. (31 августа 2017 г.). «Временная эволюция высокоэнергетического облучения и содержания воды на экзопланетах TRAPPIST-1» . Астрономический журнал . 154 (3): 121. arXiv : 1708.09484 . Бибкод : 2017AJ....154..121B . дои : 10.3847/1538-3881/aa859c . hdl : 1721.1/112267 . S2CID 44398519 .
- Брассер, Р.; Пикьерри, Дж.; Добос, В.; Барр, AC (29 июля 2022 г.). «Долгосрочная приливная эволюция системы TRAPPIST-1» . Ежемесячные уведомления Королевского астрономического общества . 515 (2): 2373–2385. arXiv : 2207.05336 . дои : 10.1093/mnras/stac1907 . ISSN 1365-2966 .
- Бургассер, Адам Дж.; Мамаек, Эрик Э. (17 августа 2017 г.). «Об эпохе системы TRAPPIST-1» . Астрофизический журнал . 845 (2): 110. arXiv : 1706.02018 . Бибкод : 2017ApJ...845..110B . дои : 10.3847/1538-4357/aa7fea . S2CID 119464994 .
- Кароне, Л.; Кеппенс, Р.; Дечин, Л.; Хеннинг, Т. (1 февраля 2018 г.). «Стратосферная циркуляция на экзоземлях, заблокированных приливами» . Ежемесячные уведомления Королевского астрономического общества . 473 (4): 4672–4685. arXiv : 1711.11446 . Бибкод : 2018MNRAS.473.4672C . дои : 10.1093/mnras/stx2732 . ISSN 1365-2966 . S2CID 119049536 .
- Чао, Кенг-Сянь; де Граффенрид, Ребекка; Лах, Маккензи; Нельсон, Уильям; Труакс, Келли; Гайдос, Эрик (1 мая 2021 г.). «Лавовые миры: от ранней Земли до экзопланет». Геохимия . 81 (2): 125735. arXiv : 2012.07337 . Бибкод : 2021ЧЭГ...81л5735С . doi : 10.1016/j.chemer.2020.125735 . ISSN 0009-2819 . S2CID 229153893 .
- Чеклер, Джейд; Мену, Кристен; Эббот, Дориан С. (18 августа 2017 г.). «Нет снежного кома на обитаемых планетах с приливной блокировкой» . Астрофизический журнал . 845 (2): 132. arXiv : 1705.08904 . Бибкод : 2017ApJ...845..132C . дои : 10.3847/1538-4357/aa80e1 . S2CID 13719958 .
- Цзяо, май (октябрь 2019 г.). «Инфракрасное селфи» . Природная астрономия . 3 (10): 880. Бибкод : 2019НатАс...3..880С . дои : 10.1038/s41550-019-0919-4 . ISSN 2397-3366 . S2CID 204718432 .
- Чайлдс, Анна С.; Мартин, Ребекка Г.; Ливио, Марио (1 октября 2022 г.). «Жизнь на экзопланетах в обитаемой зоне М-карликов?» . Письма астрофизического журнала . 937 (2): Л41. arXiv : 2209.02860 . Бибкод : 2022ApJ...937L..41C . дои : 10.3847/2041-8213/ac9052 . S2CID 252110686 .
- Чайлдс, Анна С.; Шекспир, Коди; Райс, Дэвид Р.; Ян, Чао-Чин; Штеффен, Джейсон Х. (24 июля 2023 г.). «Ограничения состава планет TRAPPIST-1 с момента их формирования» . Ежемесячные уведомления Королевского астрономического общества . 524 (3): 3749–3768. arXiv : 2307.04989 . дои : 10.1093/mnras/stad2110 .
- Цисевски, Джесси (2017). «В поисках земных аналогов» . Значение . 14 (2): 22–25. дои : 10.1111/j.1740-9713.2017.01017.x . ISSN 1740-9713 . S2CID 157345539 .
- Клотье, Райан; Трио, Амори HMJ (11 ноября 2016 г.). «Перспективы обнаружения эффекта Росситера-Маклафлина у планет земного типа: тестовый пример TRAPPIST-1b и c» . Ежемесячные уведомления Королевского астрономического общества . 462 (4): 4018–4027. arXiv : 1608.01334 . Бибкод : 2016MNRAS.462.4018C . дои : 10.1093/mnras/stw1953 . ISSN 0035-8711 . S2CID 16147394 .
- Коэн, Офер; Глосер, Алекс; Гарраффо, Сесилия; Дрейк, Джереми Дж.; Белл, Джаред М. (23 марта 2018 г.). «Диссипация энергии в верхних атмосферах планет TRAPPIST-1» . Астрофизический журнал . 856 (1): Л11. arXiv : 1803.05089 . Бибкод : 2018ApJ...856L..11C . дои : 10.3847/2041-8213/aab5b5 . ПМК 7493050 . ПМИД 32944211 .
- Коста, Э.; Мендес, РА; Джао, В.-К.; Генри, Ти Джей; Субасаваж, JP; Янна, Пенсильвания (4 августа 2006 г.). «Солнечное соседство. XVI. Параллаксы от CTIOPI: окончательные результаты программы 1,5-метрового телескопа». Астрономический журнал . 132 (3): 1234. Бибкод : 2006AJ....132.1234C . CiteSeerX 10.1.1.622.2310 . дои : 10.1086/505706 . S2CID 18952940 .
- Ковоне, Джованни; Иенко, Риккардо М.; Каччапуоти, Лука; Инно, Лаура (11 августа 2021 г.). «Эффективность кислородного фотосинтеза на планетах земного типа в обитаемой зоне» . Ежемесячные уведомления Королевского астрономического общества . 505 (3): 3329–3335. arXiv : 2104.01425 . Бибкод : 2021MNRAS.505.3329C . дои : 10.1093/mnras/stab1357 . ISSN 1365-2966 . S2CID 233025250 .
- Кутри, РМ; Скрутские, М.Ф.; Ван Дайк, С.; Бейхман, Калифорния; Карпентер, Дж. М.; Честер, Т.; Камбрези, Л.; Эванс, Т.; Фаулер, Дж.; Гизис, Дж.; Ховард, Э.; Хухра, Дж.; Джарретт, Т.; Копан, Эл; Киркпатрик, доктор юридических наук; Лайт, РМ; Марш, Калифорния; Маккаллон, Х.; Шнайдер, С.; Стенинг, Р.; Сайкс, М.; Вайнберг, М.; Уитон, Вашингтон; Уилок, С.; Закариас, Н. (июнь 2003 г.). «Онлайн-каталог данных VizieR: Каталог точечных источников всего неба 2MASS (Cutri + 2003)». Сборник электронных каталогов CDS/ADC (2246): II/246. Бибкод : 2003yCat.2246....0C .
- Дельрес, Л.; Гиллон, М.; Трио, AHMJ; Демори, Бо.; де Вит, Дж.; Ингаллс, Дж. Г.; Агол, Э.; Болмонт, Э.; Бурданов А.; Бургассер, AJ; Кэри, С.Дж.; Джехин, Э.; Леконт, Дж.; Ледерер, С.; Келос, Д.; Селсис, Ф.; Ван Гроотел, В. (11 апреля 2018 г.). «Наблюдения TRAPPIST-1 со Спитцером в начале 2017 года» . Ежемесячные уведомления Королевского астрономического общества . 475 (3): 3577–3597. arXiv : 1801.02554 . Бибкод : 2018MNRAS.475.3577D . дои : 10.1093/mnras/sty051 . ISSN 1365-2966 . S2CID 54649681 .
- Дельрес, Л.; Мюррей, Калифорния; Посуэлос, Ф.Дж.; Нарита, Н.; Дюкро, Э.; Тиммерманс, М.; Ватанабэ, Н.; Бургассер, AJ; Хирано, Т.; Рэкхэм, БВ; Стассон, КГ; Ван Гроотель, В.; Аганзе, Дж.; Коинтепас, М.; Хауэлл, С.; Кальтенеггер, Л.; Нираула, П.; Себастьян, Д.; Альменара, Дж. М.; Баркауи, К.; Бэйкрофт, штат Техас; Бонфилс, X.; Буши, Ф.; Бурданов А.; Колдуэлл, округ Колумбия; Шарбонно, Д.; Чарди, ДР; Коллинз, Калифорния; Дайлан, Т.; Демори, Б.-О.; де Вит, Дж.; Дрансфилд, Г.; Фахардо-Акоста, SB; Фаусно, М.; Фукуи, А.; Фурлан, Э.; Гарсия, LJ; Гнилка, КЛ; Гомес Макео Чу, Ю.; Гомес-Муньос, Массачусетс; Гюнтер, Миннесота; Харакава, Х.; Хэн, К.; Хутон, MJ; Хори, Ю.; Икома, М.; Джехин, Э.; Дженкинс, Дж. М.; Кагетани, Т.; Каваучи, К.; Кимура, Т.; Кодама, Т.; Котани, Т.; Кришнамурти, В.; Хорс, Т.; Куновац, В.; Кусакабе, Н.; Лэтэм, Д.В.; Литтлфилд, К.; МакКормак, Дж.; Мелис, Дж.; Мори, М.; Мургас, Ф.; Палле, Э.; Педерсен, ПП; Келос, Д.; Рикер, Г.; Сабин, Л.; Шанче, Н.; Шроффенеггер, У.; Сигер, С.; Шиао, Б.; Сохи, С.; Стоя, MR; Тамура, М.; Тайссен, Калифорния; Томпсон, С.Дж.; Трио, AHMJ; Вандерспек, Р.; Вивард, С.; Уэллс, Р.Д.; Винн, JN; Цзоу, Ю.; Суньига-Фернандес, С.; Гиллон, М. (8 сентября 2022 г.). «Две суперземли умеренного пояса проходят транзитом через ближайшего карлика позднего типа М» . Астрономия и астрофизика . 667 : А59. arXiv : 2209.02831 . Бибкод : 2022A&A...667A..59D . дои : 10.1051/0004-6361/202244041 . ISSN 0004-6361 . S2CID 252110654 — через arXiv .
- Деминг, Дрейк; Натсон, Хизер А. (май 2020 г.). «Основные достижения экзопланетной науки со спутника Спитцер». Природная астрономия . 4 (5): 453–466. arXiv : 2005.11331 . Бибкод : 2020НатАс...4..453Д . дои : 10.1038/s41550-020-1100-9 . ISSN 2397-3366 . S2CID 218870017 .
- Демори, Б.-О.; Посуэлос, Ф.Дж.; Чу, Ю. Гомес Макео; Сабин, Л.; Петруччи, Р.; Шроффенеггер, У.; Гримм, СЛ; Шестович, М.; Гиллон, М.; МакКормак, Дж.; Баркауи, К.; Бенц, В.; Берила, А.; Буши, Ф.; Бурданов А.; Коллинз, Калифорния; Уайт, Дж. де; Туалетная, компакт-диск; Гарсия, LJ; Джакалоне, С.; Герра, П.; Халдеманн, Дж.; Хэн, К.; Джехин, Э.; Жоффре, Э.; Кейн, СР; Лилло-Бокс, Дж.; Менье, В.; Мордасини, Дж.; Моррис, Б.М.; Нираула, П.; Келос, Д.; Рэкхэм, БВ; Савел, АБ; Субкиу, А.; Срдок, Г.; Стассон, КГ; Трио, AHMJ; Замбелли, Р.; Рикер, Г.; Лэтэм, Д.В.; Сигер, С.; Винн, JN; Дженкинс, Дж. М.; Кальварио-Веласкес, Т.; Эррера, Х. А. Франко; Колорадо, Э.; Зепеда, Э.О. Кадена; Фигероа, Л.; Уотсон, AM; Луго-Ибарра, EE; Карги, Л. ; Гиса, Г.; Эррера, Дж.; Диас, Г. Сьерра; Суарес, Х.К.; Баррадо, Д.; Баттл, Нью-Мексико; Бенхалдун, З.; Чонтос, А.; Дай, Ф.; Эссак, З.; Гачуи, М.; Хуанг, CX; Хубер, Д.; Исааксон, Х.; Лиссауэр, Джей Джей; Моралес-Кальдерон, М.; Робертсон, П.; Рой, А.; Твикен, доктор медицинских наук; Вандербург, А.; Вайс, LM (1 октября 2020 г.). «СуперЗемля и субНептун, вращающиеся вокруг яркого и тихого карлика М3 TOI-1266». Астрономия и астрофизика . 642 : А49. arXiv : 2009.04317 . Бибкод : 2020A&A...642A..49D . дои : 10.1051/0004-6361/202038616 . ISSN 0004-6361 . S2CID 221554586 .
- Детерманн, Йорг Матиас (2019). Космическая наука и арабский мир: астронавты, обсерватории и национализм на Ближнем Востоке . Издательство Блумсбери. ISBN 978-1-83860-015-0 . OCLC 1122719747 .
- Диас, РФ (2017). «Исследование новых миров. Обзор наблюдений внесолнечных планет». Бюллетень Аргентинской астрономической ассоциации «Аргентинское серебро» . 59 : 183–189. Бибкод : 2017BAAA...59..183D .
- Донг, Чуанфэй; Джин, Мэн; Лингам, Манасви; Айрапетян Владимир Сергеевич; Ма, Инцзюань; ван дер Хольст, Барт (9 января 2018 г.). «Утечка атмосферы с планет TRAPPIST-1 и последствия для обитаемости» . Труды Национальной академии наук . 115 (2): 260–265. arXiv : 1705.05535 . Бибкод : 2018PNAS..115..260D . дои : 10.1073/pnas.1708010115 . ISSN 0027-8424 . ПМК 5777028 . ПМИД 29284746 . S2CID 31195274 .
- дос Сантос, Леонардо А.; Бурье, Винсент; Эренрайх, Дэвид; Камеда, Синго (1 февраля 2019 г.). «Наблюдаемость богатых водородом экзосфер на экзопланетах земного типа». Астрономия и астрофизика . 622 : А46. arXiv : 1812.02145 . Бибкод : 2019A&A...622A..46D . дои : 10.1051/0004-6361/201833392 . ISSN 0004-6361 . S2CID 119013251 .
- Дюкро, Э.; Гиллон, М.; Дельрес, Л.; Агол, Э.; Риммер, П.; Тюрбет, М.; Гюнтер, Миннесота; Демори, Б.-О.; Трио, AHMJ; Болмонт, Э.; Бургассер, А.; Кэри, С.Дж.; Ингаллс, Дж. Г.; Джехин, Э.; Леконт, Дж.; Ледерер, С.М.; Келос, Д.; Раймонд, С.Н.; Селсис, Ф.; Ван Гроотель, В.; Вит, Ж. де (1 августа 2020 г.). «TRAPPIST-1: Глобальные результаты научно-исследовательской программы Spitzer Red Worlds». Астрономия и астрофизика . 640 : А112. arXiv : 2006.13826 . Бибкод : 2020A&A...640A.112D . дои : 10.1051/0004-6361/201937392 . ISSN 0004-6361 . S2CID 220041987 .
- Дюкро, Эльза (2 апреля 2021 г.). «Краткая история системы TRAPPIST-1. Статья по приглашению – Приглашенный доклад» . Бюллетень Королевского общества наук Льежа . 90 . дои : 10.25518/0037-9565.10277 . ISSN 0037-9565 . S2CID 246354436 .
- Игер, Джейк К.; Райхельт, Дэвид Дж.; Мейн, Натан Дж.; Ламберт, Ф. Хьюго; Сергеев Денис Евгеньевич; Риджуэй, Роберт Дж.; Маннерс, Джеймс; Бутл, Ян А.; Лентон, Тимоти М.; Кохари, Кристиан (1 июля 2020 г.). «Последствия различных звездных спектров для климата приливно-зависимых экзопланет, подобных Земле». Астрономия и астрофизика . 639 : А99. arXiv : 2005.13002 . Бибкод : 2020A&A...639A..99E . дои : 10.1051/0004-6361/202038089 . ISSN 0004-6361 . S2CID 218900900 .
- Эдвардс, Билли; Чангат, Квентин; Мори, Маюко; Анисман, Лара О.; Морван, Марио; Да, Кай Хоу; Циарас, Ангелос; Аль-Рефаи, Ахмед; Вальдманн, Инго; Тинетти, Джованна (24 декабря 2020 г.). «Хаббл WFC3 Спектроскопия обитаемой зоны Суперземли LHS 1140 b» . Астрономический журнал . 161 (1): 44. arXiv : 2011.08815 . Бибкод : 2021AJ....161...44E . дои : 10.3847/1538-3881/abc6a5 . S2CID 226975730 .
- Эльшабури, СМ; Абуэльмагд, Эльбаз И.; Калантонис, В.С.; Пердиос, EA (25 августа 2016 г.). «Плоская ограниченная задача трех тел, когда оба основных тела являются трехосными твердыми телами: точки равновесия и периодические орбиты» . Астрофизика и космическая наука . 361 (9): 315. Бибкод : 2016Ap&SS.361..315E . дои : 10.1007/s10509-016-2894-x . ISSN 1572-946X . S2CID 254252200 .
- «Исследуй поверхность – ТРАППИСТ 1d» . Бюро путешествий Экзопланета . НАСА . Проверено 16 ноября 2021 г.
- Фаббиан, Д.; Симониелло, Р.; Колле, Р.; Крискуоли, С.; Корхонен, Х.; Кривова, Н.А.; Ола, К.; Жув, Л.; Соланки, СК; Альварадо-Гомес, доктор юридических наук; Бут, Р.; Гарсия, РА; Лехтинен, Дж.; См. В. (2017). «Переменность магнитной активности звезд солнечного типа» . Астрономические новости . 338 (7): 753–772. Бибкод : 2017AN....338..753F . дои : 10.1002/asna.201713403 . ISSN 1521-3994 . S2CID 53572712 .
- Фарриш, Элисон О.; Александр, Дэвид; Маруо, Мэй; ДеРоса, Марк; Тоффолетто, Фрэнк; Шиола, Энтони М. (30 октября 2019 г.). «Характеристика магнитного окружения звездных систем экзопланет» . Астрофизический журнал . 885 (1): 51. Бибкод : 2019ApJ...885...51F . дои : 10.3847/1538-4357/ab4652 . S2CID 209907654 .
- Фидрик, Рассвет; Юнг, Джи; Нимак, Боб; Диксон, Дон (17 августа 2020 г.). Искусство и наука изображения миров: планетарий обсерватории Гриффита демонстрирует признаки жизни . Переговоры ACM SIGGRAPH 2020. стр. 1–2. дои : 10.1145/3388767.3411060 . ISBN 9781450379717 . S2CID 221178064 .
- Фишер, Кристиан; Саур, Иоахим (14 февраля 2019 г.). «Изменчивое во времени электромагнитное взаимодействие звезды и планеты: система TRAPPIST-1 как образцовый случай» . Астрофизический журнал . 872 (1): 113. arXiv : 1901.02747 . Бибкод : 2019ApJ...872..113F . дои : 10.3847/1538-4357/aafaf2 . S2CID 119326120 .
- Стая, Марио; Тернер, Нил Дж.; Малдерс, Гийс Д.; Хасэгава, Ясухиро; Нельсон, Ричард П.; Битч, Бертрам (1 октября 2019 г.). «Формирование и миграция планет вблизи фронта сублимации силиката в протопланетных дисках». Астрономия и астрофизика . 630 : А147. arXiv : 1910.03901 . Бибкод : 2019A&A...630A.147F . дои : 10.1051/0004-6361/201935806 . ISSN 0004-6361 . S2CID 203027318 .
- Фортни, Джонатан Дж. (2018). «Моделирование экзопланетной атмосферы: обзор». В Боззе, Валерио; Манчини, Луиджи; Соццетти, Алессандро (ред.). Астрофизика экзопланетных атмосфер . Библиотека астрофизики и космических наук. Том. 450. Чам: Springer International Publishing. стр. 51–88. arXiv : 1804.08149 . дои : 10.1007/978-3-319-89701-1_2 . ISBN 978-3-319-89700-4 . S2CID 59406383 .
- Фрайр, Хуан Андрес; Фельдманн, Мариус; Уолтер, Феликс; Фантино, Елена; Берли, Скотт К. (август 2019 г.). «Сеть в межзвездных измерениях: общение с TRAPPIST-1» . Транзакции IEEE по аэрокосмическим и электронным системам . 55 (4): 1656–1665. Бибкод : 2019ITAES..55.1656F . дои : 10.1109/TAES.2018.2874149 . hdl : 11336/105240 . ISSN 1557-9603 . S2CID 117702090 .
- Фраскетти, Ф.; Дрейк, Джей-Джей; Альварадо-Гомес, доктор юридических наук; Мощо, СП; Гарраффо, К.; Коэн, О. (18 марта 2019 г.). «Звёздные энергетические частицы в магнитно-турбулентных обитаемых зонах планетных систем типа TRAPPIST-1» . Астрофизический журнал . 874 (1): 21. arXiv : 1902.03732 . Бибкод : 2019ApJ...874...21F . дои : 10.3847/1538-4357/ab05e4 . hdl : 10150/633277 . S2CID 119081355 .
- Габриэль, Трэвис С.Дж.; Хорн, Харрисон В. (1 июля 2021 г.). «Зависимости ударного нагрева мантии при парной аккреции» . Письма астрофизического журнала . 915 (2): Л32. Бибкод : 2021ApJ...915L..32G . дои : 10.3847/2041-8213/abffd1 . S2CID 235817461 .
- «Выпуск ранних данных Gaia 3 (EDR3)» . Архив ЕКА Гайя . Европейское космическое агентство . 2021 . Проверено 1 сентября 2023 г.
- Гарго, Мюриэль; Амилс, Рикардо; Кинтанилья, Хосе Серничаро; Кливс, Хендерсон Джеймс; Ирвин, Уильям М.; Пинти, Даниэле Л.; Визо, Мишель, ред. (2011). Энциклопедия астробиологии . Берлин, Гейдельберг: Springer Berlin Heidelberg. Бибкод : 2011eab..book.....G . дои : 10.1007/978-3-642-11274-4 . ISBN 978-3-642-11271-3 . S2CID 90186988 .
- Гибб, Брюс К. (27 октября 2022 г.). «За пределами Хаббла» . Природная химия . 14 (11): 1207–1209. Бибкод : 2022НатЧ..14.1207Г . дои : 10.1038/s41557-022-01080-2 . ISSN 1755-4349 . ПМИД 36302866 . S2CID 253184326 .
- Гиллон, Майкл (февраль 2020 г.). «Жизнь под другим солнцем: от научной фантастики к науке». Европейский обзор . 28 (1): 18–39. дои : 10.1017/S1062798719000267 . hdl : 2268/254712 . ISSN 1062-7987 . S2CID 210575064 .
- Гиллон, Майкл (2020). TRAPPIST-1: К сравнительному изучению наземных миров умеренного пояса (Отчет). стр. 49–52.
- Гиллон, М.; Джехин, Э.; Дельрес, Л.; Магейн, П.; Опитом, К.; Сохи, С. (июль 2013 г.). SPECULOOS: поиск обитаемых планет, затмевающих УЛЬТРАХОЛОДНЫЕ звезды (PDF) . Протозвезды и планеты VI. Гейдельберг. hdl : 2268/159868 .
- Гиллон, Майкл; Жехин, Эммануэль; Ледерер, Сьюзен М.; Дельрес, Летиция; де Вит, Жюльен; Бурданов Артем; Ван Гроотель, Валери; Бургассер, Адам Дж.; Трио, Амори HMJ; Опитом, Сириэль; Демори, Брис-Оливье; Саху, Девендра К.; Бардалес Гальюффи, Даниэлла; Магейн, Пьер; Кело, Дидье (май 2016 г.). «Планеты умеренного климата размером с Землю, проходящие транзитом через ближайшую ультрахолодную карликовую звезду» . Природа . 533 (7602): 221–224. arXiv : 1605.07211 . Бибкод : 2016Natur.533..221G . дои : 10.1038/nature17448 . ISSN 1476-4687 . ПМК 5321506 . ПМИД 27135924 .
- Гиллон, Майкл; Трио, Амори HMJ; Демори, Брис-Оливье; Жехин, Эммануэль; Агол, Эрик; Дек, Кэтрин М.; Ледерер, Сьюзен М.; де Вит, Жюльен; Бурданов Артем; Ингаллс, Джеймс Г.; Болмонт, Эмелин; Леконт, Джереми; Раймонд, Шон Н.; Селсис, Франк; Тюрбет, Мартин; Баркауи, Халид; Бургассер, Адам; Берли, Мэтью Р.; Кэри, Шон Дж.; Чаушев, Александр; Коппервит, Крис М.; Дельрес, Летиция; Фернандес, Катарина С.; Холдсворт, Дэниел Л.; Котце, Энрико Дж.; Ван Гроотель, Валери; Алмлики, Ясин; Бенхалдун, Зухайр; Магейн, Пьер; Кело, Дидье (февраль 2017 г.). «Семь планет земной группы с умеренным климатом вокруг близлежащей ультрахолодной карликовой звезды TRAPPIST-1» . Природа . 542 (7642): 456–460. arXiv : 1703.01424 . Бибкод : 2017Natur.542..456G . дои : 10.1038/nature21360 . ISSN 1476-4687 . ПМК 5330437 . ПМИД 28230125 . S2CID 4391722 .
- Гиллон, Майкл; Медоуз, Виктория; Агол, Эрик; Бургассер, Адам Дж.; Деминг, Дрейк; Дойон, Рене; Фортни, Джонатан; Крейдберг, Лаура; Оуэн, Джеймс; Селсис, Франк; де Вит, Жюльен; Люстиг-Йегер, Джейкоб; Рэкхэм, Бенджамин В. (2 декабря 2020 г.). «Инициатива сообщества TRAPPIST-1 JWST» . Бюллетень ААС . 52 (2): 0208. arXiv : 2002.04798 . Бибкод : 2020BAAS...52.0208G . дои : 10.3847/25c2cfeb.afbf0205 . S2CID 211082517 .
- Гизис, Джон Э.; Моне, Дэвид Г.; Рид, И. Нил; Киркпатрик, Дж. Дэви; Либерт, Джеймс; Уильямс, Рик Дж. (август 2000 г.). «Новые соседи из 2MASS: активность и кинематика внизу главной последовательности». Астрономический журнал . 120 (2): 1085–1099. arXiv : astro-ph/0004361 . Бибкод : 2000AJ....120.1085G . дои : 10.1086/301456 . S2CID 18819321 .
- Глейзер, Дональд М.; Хартнетт, Хилэри Эллен; Деш, Стивен Дж.; Унтерборн, Кайман Т.; Анбар, Ариэль; Бюсеккер, Штеффен; Фишер, Тереза; Глейзер, Стивен; Кейн, Стивен Р.; Лиссе, Кэри М.; Миллсапс, Камерариан; Нойер, Сюзанна; О'Рурк, Джозеф Г.; Сантос, Нуно; Уокер, Сара Имари; Золотов Михаил (апрель 2020 г.). «Обнаруживаемость жизни с использованием кислорода на пелагических планетах и водных мирах» . Астрофизический журнал . 893 (2): 163. arXiv : 2004.03631 . Бибкод : 2020ApJ...893..163G . дои : 10.3847/1538-4357/ab822d . ISSN 0004-637X .
- Стекольщик, Эми Л.; Ховард, Уорд С.; Корбетт, Хэнк; Закон, Николас М.; Рацлофф, Джеффри К.; Форс, Октави; дель Сер, Даниэль (27 августа 2020 г.). «Ограничения Evryscope и K2 на возникновение супервспышек TRAPPIST-1 и обитаемость планет» . Астрофизический журнал . 900 (1): 27. arXiv : 2006.14712 . Бибкод : 2020ApJ...900...27G . дои : 10.3847/1538-4357/aba4a6 . S2CID 220128346 .
- Голдсмит, Дональд (10 сентября 2018 г.). Экзопланеты: скрытые миры и поиски внеземной жизни . Издательство Гарвардского университета. Бибкод : 2018ehwq.book.....G . дои : 10.4159/9780674988897 . ISBN 978-0-674-98889-7 . S2CID 240182683 .
- Гонсалес, Эйлин С.; Фаэрти, Жаклин К .; Ганье, Джонатан; Теске, Йоханна; Маквильям, Эндрю; Круз, Келле (29 ноября 2019 г.). «Повторный анализ фундаментальных параметров и возраста TRAPPIST-1» . Астрофизический журнал . 886 (2): 131. arXiv : 1909.13859 . Бибкод : 2019ApJ...886..131G . дои : 10.3847/1538-4357/ab48fc . S2CID 203594024 .
- Грейвер, Александр; Бауэр, Дэн Дж.; Саур, Иоахим; Дорн, Кэролайн; Моррис, Бретт М. (7 декабря 2022 г.). «Внутренний нагрев скалистых экзопланет от звездных вспышек с применением к TRAPPIST-1» . Письма астрофизического журнала . 941 (1): L7. arXiv : 2211.06140 . Бибкод : 2022ApJ...941L...7G . дои : 10.3847/2041-8213/aca287 . S2CID 253499175 .
- Гренфелл, Джон Ли (13 ноября 2017 г.). «Обзор экзопланетных биосигнатур». Отчеты по физике . 713 : 1–17. arXiv : 1710.03976 . Бибкод : 2017ФР...713....1Г . doi : 10.1016/j.physrep.2017.08.003 . ISSN 0370-1573 . S2CID 119400674 .
- Гренфелл, Джон Ли; Леконт, Джереми; Забудь, Франсуа; Годольт, Марейке; Каррион-Гонсалес, Оскар; Ноак, Лена; Тянь, Фэн; Рауэр, Хайке; Гайяр, Фабрис; Больмонт, Эмелин; Шарне, Бенджамин; Тюрбет, Мартин (август 2020 г.). «Возможное атмосферное разнообразие экзопланет малой массы - некоторые центральные аспекты». Обзоры космической науки . 216 (5): 98. arXiv : 2101.01277 . Бибкод : 2020ССРв..216...98Г . дои : 10.1007/s11214-020-00716-4 . ISSN 1572-9672 . S2CID 225473867 .
- Грессье, А.; Мори, М.; Чангат, К.; Эдвардс, Б.; Болье, Ж.-П.; Марк, Э.; Чарней, Б. (2022). «Спектр пропускания TRAPPIST-1 h в ближнем инфракрасном диапазоне с использованием наблюдений Hubble WFC3 G141». Астрономия и астрофизика . 658 : А133. arXiv : 2112.05510 . Бибкод : 2022A&A...658A.133G . дои : 10.1051/0004-6361/202142140 . ISSN 0004-6361 . S2CID 245091619 — через arXiv .
- Гримм, Саймон Л.; Демори, Брис-Оливье; Гиллон, Майкл; Дорн, Кэролайн; Агол, Эрик; Бурданов Артем; Дельрес, Летиция; Шестович, Марко; Трио, Амори HMJ; Тюрбет, Мартин; Больмонт, Эмелин; Калдас, Энтони; Уайт, Жюльен де; Жехин, Эммануэль; Леконт, Джереми; Раймонд, Шон Н.; Ван Гроотель, Валери; Бургассер, Адам Дж.; Кэри, Шон; Фабрики, Дэниел; Хенг, Кевин; Эрнандес, Дэвид М.; Ингаллс, Джеймс Г.; Ледерер, Сьюзен; Цельсис, Франк; Кело, Дидье (1 мая 2018 г.). «Природа экзопланет TRAPPIST-1». Астрономия и астрофизика . 613 : А68. arXiv : 1802.01377 . Бибкод : 2018A&A...613A..68G . дои : 10.1051/0004-6361/201732233 . ISSN 0004-6361 . S2CID 3441829 .
- Гимон, Клэр Мари; Радж, Джон Ф.; Шорттл, Оливер (1 марта 2022 г.). «Синий мрамор, застойная крышка: может ли динамическая топография предотвратить водный мир?» . Планетарный научный журнал . 3 (3): 66. arXiv : 2201.05636 . Бибкод : 2022PSJ.....3...66G . дои : 10.3847/psj/ac562e . ISSN 2632-3338 . S2CID 246015582 .
- Гуриди, Хосе А.; Пертуз, Хулио А.; Пфотенхауэр, Себастьян М. (1 марта 2020 г.). «Естественные лаборатории как инструменты политики для технологического обучения и наращивания институционального потенциала: пример астрономического кластера Чили». Исследовательская политика . 49 (2): 103899. doi : 10.1016/j.respol.2019.103899 . ISSN 0048-7333 . S2CID 197453914 .
- Гюнтер, Максимилиан Н.; Берардо, Дэвид А.; Дюкро, Эльза; Мюррей, Катриона А.; Стассон, Кейван Г.; Ола, Каталин; Баума, LG; Раппапорт, Саул; Винн, Джошуа Н.; Файнштейн, Адина Д.; Мэтьюз, Элизабет Дж.; Себастьян, Дэниел; Рэкхэм, Бенджамин В.; Клетка, Балинт; Ж. Трио, Амори Х.М.; Гиллен, Эдвард; Левин, Алан М.; Демори, Брис-Оливье; Гиллон, Майкл; Кело, Дидье; Рикер, Джордж Р.; Вандерспек, Роланд К.; Сигер, Сара; Лэтэм, Дэвид В.; Дженкинс, Джон М.; Брассер, CE; Колон, Книколь Д.; Дилан, Коппер; Дельрес, Летиция; Фаусно, Майкл; Гарсия, Лайонел Дж.; Джаяраман, Рахул; Жехин, Эммануэль; Кристиансен, Мартти Х.; Круйссен, Дж. М. Дидерик; Педерсен, Питер Пильманн; Посуэлос, Франсиско Дж.; Родригес, Джозеф Э.; Волер, Билл; Чжан, Чжучан (1 апреля 2022 г.). «Сложная модуляция быстро вращающихся молодых M-карликов: добавление частей к головоломке» . Астрономический журнал . 163 (4): 144. arXiv : 2008.11681 . Бибкод : 2022AJ....163..144G . дои : 10.3847/1538-3881/ac503c . S2CID 221319588 .
- Гутьеррес, CM; Арнольд, Д.; Копли, Д.; Медная крупа, СМ; Харви, Э.; Джермак, Х.; Кнапен, Дж.; МакГрат, А.; Ория, А.; Реболо, Р.; Стил, Айова; Торрес, М. (2019). «Новый 4-метровый роботизированный телескоп» . Астрономические Нахрихтен . 340 (1–3): 40–45. Бибкод : 2019AN....340...40G . дои : 10.1002/asna.201913556 . ISSN 1521-3994 . S2CID 133136386 .
- Хаким, Каустуб; Ривольдини, Аттилио; Ван Холст, Тим; Коттенье, Стефан; Джейкен, Ян; Чуст, Томас; Штайнле-Нойманн, Герд (1 октября 2018 г.). «Новое ab initio уравнение состояния ГПУ-Fe и его влияние на внутреннюю структуру и соотношение массы и радиуса скалистых суперземель». Икар . 313 : 61–78. arXiv : 1805.10530 . Бибкод : 2018Icar..313...61H . дои : 10.1016/j.icarus.2018.05.005 . ISSN 0019-1035 . S2CID 119442637 .
- Национальная астрономическая обсерватория Японии (май 2022 г.). Справочник научных таблиц . дои : 10.1142/9789813278523_0001 . ISBN 978-981-3278-53-0 .
- Харбах, Лаура М.; Мощёва, София П.; Гарраффо, Сесилия; Дрейк, Джереми Дж.; Альварадо-Гомес, Хулиан Д.; Коэн, Офер; Фраскетти, Федерико (1 июня 2021 г.). «Звездные ветры вызывают сильные изменения в характере испарения экзопланеты и характеристиках транзитного поглощения» . Астрофизический журнал . 913 (2): 130. arXiv : 2012.05922 . Бибкод : 2021ApJ...913..130H . дои : 10.3847/1538-4357/abf63a . S2CID 228375956 .
- Хейсинг, Мэтью З.; Саселов, Димитар Д.; Эрнквист, Ларс; Луиза Тио Хамфри, Ана (1 июня 2021 г.). «Насколько плоской может стать планетная система? I. Случай TRAPPIST-1» . Астрофизический журнал . 913 (2): 126. Бибкод : 2021ApJ...913..126H . дои : 10.3847/1538-4357/abf8a8 . S2CID 219262616 .
- Хори, Ясунори; Огихара, Масахиро (28 января 2020 г.). «Есть ли на планетах TRAPPIST-1 атмосфера, богатая водородом?» . Астрофизический журнал . 889 (2): 77. arXiv : 1912.05749 . Бибкод : 2020ApJ...889...77H . дои : 10.3847/1538-4357/ab6168 . S2CID 209324289 .
- Ховард, Уорд С.; Ковальски, Адам Ф.; Флэгг, Лаура; МакГрегор, Мередит А.; Лим, Оливия; Радика, Майкл; Пиоле, Кэролайн; Рой, Пьер-Алексис; Лафреньер, Давид; Беннеке, Бьёрн; Браун, Александр; Эспиноза, Нестор; Дойон, Рене; Куломб, Луи-Филипп; Джонстон, Дуг; Коуэн, Николас Б.; Джаявардхана, Рэй; Тернер, Джейк Д.; Данг, Лиза (1 декабря 2023 г.). «Характеристика спектров вспышек в ближнем инфракрасном диапазоне от TRAPPIST-1 во время наблюдений транзитной спектроскопии JWST» . Астрофизический журнал . 959 (1): 64. arXiv : 2310.03792 . Бибкод : 2023ApJ...959...64H . дои : 10.3847/1538-4357/acfe75 .
- Хауэлл, Стив Б.; Эверетт, Марк Э.; Хорч, Эллиотт П.; Уинтерс, Дженнифер Г.; Хирш, Леа; Нусдео, Дэн; Скотт, Николас Дж. (13 сентября 2016 г.). «Визуализация спеклов исключает спутники малой массы, вращающиеся вокруг родительской звезды экзопланеты Траппист-1» . Астрофизический журнал . 829 (1): Л2. arXiv : 1610.05269 . Бибкод : 2016ApJ...829L...2H . дои : 10.3847/2041-8205/829/1/l2 . S2CID 119183657 .
- Хауэлл, Стив Б., изд. (сентябрь 2020 г.). Миссия НАСА «Кеплер» . Издательство ИОП. дои : 10.1088/2514-3433/ab9823ch3 . ISBN 978-0-7503-2296-6 . S2CID 224941774 .
- Хьюз, Джанетт (2022). Хьюз, Джанетт (ред.). Создание, создатели, творческие пространства: переход к созданию в 20 школах . Чам: Спрингер. дои : 10.1007/978-3-031-09819-2 . ISBN 978-3-031-09819-2 . S2CID 251731356 .
- Эх, Джегуг; Кемптон, Элиза М.-Р.; Уиттакер, Эмили А.; Лессард, Мэдлин (июль 2023 г.). «Ограничение толщины атмосферы TRAPPIST-1 b по данным наблюдений вторичного затмения JWST на длине волны 15 мкм» . Письма астрофизического журнала . 952 (1): Л4. arXiv : 2305.10414 . Бибкод : 2023ApJ...952L...4I . дои : 10.3847/2041-8213/ace03b . ISSN 2041-8205 .
- Янич, Александр (2017). Вселенная среды обитания . Нагрудный код : 2017leun.book.....J . дои : 10.1007/978-3-662-54787-8 . ISBN 978-3-662-54786-1 .
- «Сравнение TRAPPIST-1 с Солнечной системой» . Лаборатория реактивного движения. 22 января 2021 г. Проверено 31 августа 2023 г.
- Кальтенеггер, Л.; Фаэрти, Дж. К. (июнь 2021 г.). «Звезды прошлого, настоящего и будущего, которые могут видеть Землю как транзитную экзопланету». Природа . 594 (7864): 505–507. arXiv : 2107.07936 . Бибкод : 2021Natur.594..505K . дои : 10.1038/s41586-021-03596-y . ISSN 1476-4687 . ПМИД 34163055 . S2CID 235626242 .
- Канас, Ник (2019). Звездные карты: история, искусство и картография . Международное издательство Спрингер. дои : 10.1007/978-3-030-13613-0 . ISBN 978-3-030-13612-3 . S2CID 239353025 .
- Кейн, Стивен Р.; Арни, Джада Н.; Бирн, Пол К.; Далба, Пол А.; Деш, Стивен Дж.; Хорнер, Джонти; Изенберг, Ноам Р.; Мандт, Кэтлин Э.; Медоуз, Виктория С.; Квик, Линнэ К. (февраль 2021 г.). «Фундаментальные связи между Солнечной системой и экзопланетной наукой». Журнал геофизических исследований: Планеты . 126 (2). arXiv : 2012.11628 . Бибкод : 2021JGRE..12606643K . дои : 10.1029/2020JE006643 . ISSN 2169-9100 . S2CID 233442891 .
- Кейн, Стивен Р. (13 апреля 2017 г.). «Миры без лун: ограничения экзолуны для компактных планетных систем» . Астрофизический журнал . 839 (2): Л19. arXiv : 1704.01688 . Бибкод : 2017ApJ...839L..19K . дои : 10.3847/2041-8213/aa6bf2 . S2CID 119380874 .
- Кейн, Стивен Р.; Янсен, Тиффани; Фошез, Томас; Селсис, Франк; Сеха, Альма Ю. (6 января 2021 г.). «Фазовое моделирование планетарных атмосфер TRAPPIST-1» . Астрономический журнал . 161 (2): 53. arXiv : 2012.00080 . Бибкод : 2021AJ....161...53K . дои : 10.3847/1538-3881/abcfbe . S2CID 227238721 .
- Кендалл, М.; Бирн, ПК (1 марта 2020 г.). Оценка геологических условий на дне океана скалистых планет TRAPPIST-1 (PDF) . 51-я конференция по науке о Луне и планетах. Вудлендс, Техас . п. 3030. Бибкод : 2020LPI....51.3030K .
- Кислякова, К.Г.; Ноак, Л.; Джонстон, CP; Зайцев В.В.; Фоссати, Л.; Ламмер, Х.; Ходаченко М.Л.; Одерт, П.; Гюдель, М. (декабрь 2017 г.). «Магматические океаны и усиленный вулканизм на планетах TRAPPIST-1 из-за индукционного нагрева». Природная астрономия . 1 (12): 878–885. arXiv : 1710.08761 . Бибкод : 2017НатАс...1..878К . дои : 10.1038/s41550-017-0284-0 . ISSN 2397-3366 . S2CID 119429870 .
- Кочухов, Олег (декабрь 2021 г.). «Магнитные поля М-карликов». Обзор астрономии и астрофизики . 29 (1): 1. arXiv : 2011.01781 . Бибкод : 2021A&ARv..29....1K . дои : 10.1007/s00159-020-00130-3 . ISSN 1432-0754 . S2CID 226237078 .
- Коппарла, Пушкар; Натрадж, Виджай; Крисп, Дэвид; Ботт, Кимберли; Суэйн, Марк Р.; Юнг, Юк Л. (10 сентября 2018 г.). «Наблюдение океанов в плотно упакованных планетных системах: перспективы поляризационного моделирования системы TRAPPIST-1» . Астрономический журнал . 156 (4): 143. Бибкод : 2018AJ....156..143K . дои : 10.3847/1538-3881/aad9a1 . S2CID 125467757 .
- Крал, Квентин; Вятт, Марк С.; Трио, Амори HMJ; Марино, Себастьян; Тебо, Филипп; Шорттл, Оливер (11 сентября 2018 г.). «Кометные ударники на планетах TRAPPIST-1 могут разрушить все планетарные атмосферы и восстановить вторичные атмосферы на планетах f, g и h» . Ежемесячные уведомления Королевского астрономического общества . 479 (2): 2649–2672. arXiv : 1802.05034 . Бибкод : 2018MNRAS.479.2649K . дои : 10.1093/mnras/sty1677 . ISSN 1365-2966 . S2CID 118880067 .
- Крал, Квентин; Даву, Жанна; Шарне, Бенджамин (август 2020 г.). «Формирование вторичных атмосфер на планетах земной группы в результате поздней дисковой аккреции». Природная астрономия . 4 (8): 769–775. arXiv : 2004.02496 . Бибкод : 2020НатАс...4..769К . дои : 10.1038/s41550-020-1050-2 . ISSN 2397-3366 . S2CID 214802025 .
- Криссансен-Тоттон, Дж.; Фортни, Джей Джей (1 июля 2022 г.). «Прогнозы наблюдаемых атмосфер планет траппист-1 на основе модели полностью связанной атмосферы и внутренней эволюции» . Астрофизический журнал . 933 (1): 115. arXiv : 2207.04164 . Бибкод : 2022ApJ...933..115K . дои : 10.3847/1538-4357/ac69cb . S2CID 250374670 .
- Лейн, Х. Чад; Гэдбери, Мэтью; Джинджер, Джефф; Йи, Шерри; Коминс, Нил; Хенхапл, Джек; Ривера-Роджерс, Эйдан (28 ноября 2022 г.). «Вызов интереса к STEM с помощью Minecraft в гибридном летнем лагере» . Инновации в дистанционном обучении . 3 (4). дои : 10.1037/tmb0000077 . S2CID 254344269 .
- Линхард, Ф.; Келос, Д.; Гиллон, М.; Бурданов А.; Дельрес, Л.; Дюкро, Э.; Хэндли, В.; Джехин, Э.; Мюррей, Калифорния; Трио, AHMJ; Гиллен, Э.; Мортье, А.; Рэкхэм, Б.В. (2020). «Глобальный анализ исследования транзита ультра-холодных карликов TRAPPIST» . Ежемесячные уведомления Королевского астрономического общества . 497 (3): 3790–3808. arXiv : 2007.07278 . Бибкод : 2020MNRAS.497.3790L . дои : 10.1093/mnras/staa2054 . ISSN 1365-2966 . S2CID 220525769 .
- Лим, Оливия; Беннеке, Бьёрн; Дойон, Рене; Макдональд, Райан Дж.; Пиоле, Кэролайн; Артиго, Этьен; Куломб, Луи-Филипп; Радика, Майкл; L’Heureux, Александрин; Альберт, Лоик; Рэкхэм, Бенджамин В.; Остроумие, Жюльен де; Салхи, Сальма; Рой, Пьер-Алексис; Флэгг, Лаура; Фурнье-Тондро, Мэрилу; Тейлор, Джейк; Кук, Нил Дж.; Лафреньер, Дэвид; Коуэн, Николас Б.; Кальтенеггер, Лиза; Роу, Джейсон Ф.; Эспиноза, Нестор; Черт, Лиза; Дарво-Бернье, Антуан (сентябрь 2023 г.). «Атмосферная разведка TRAPPIST-1 b с помощью JWST/NIRISS: доказательства сильного звездного загрязнения в спектрах пропускания» . Письма астрофизического журнала . 955 (1): Л22. arXiv : 2309.07047 . Бибкод : 2023ApJ...955L..22L . дои : 10.3847/2041-8213/acf7c4 . ISSN 2041-8205 .
- Линковски, Эндрю П.; Медоуз, Виктория С.; Зиеба, Себастьян; Крейдберг, Лаура; Морли, Кэролайн; Гиллон, Майкл; Селсис, Франк; Агол, Эрик; Болмонт, Эмелин; Дюкро, Эльза; Ху, Реню; Колл, Дэниел Д.Б.; Лю, Синьтун; Манделл, Ави; Суисса, Габриэль; Тамбуро, Патрик (1 сентября 2023 г.). «Потенциальный состав атмосферы TRAPPIST-1c, определенный наблюдениями JWST/MIRI на длине волны 15 мкм» . Письма астрофизического журнала . 955 (1): L7. arXiv : 2308.05899 . Бибкод : 2023ApJ...955L...7L . дои : 10.3847/2041-8213/acee02 .
- Лингам, Манасви; Леб, Авраам (апрель 2018 г.). «Физические ограничения вероятности жизни на экзопланетах». Международный журнал астробиологии . 17 (2): 116–126. arXiv : 1707.02996 . Бибкод : 2018IJAsB..17..116L . дои : 10.1017/S1473550417000179 . ISSN 1475-3006 . S2CID 35978131 .
- Лингам, Манасви; Леб, Авраам (июль 2018 г.). «Последствия приливов для жизни на экзопланетах». Астробиология . 18 (7): 967–982. arXiv : 1707.04594 . Бибкод : 2018AsBio..18..967L . дои : 10.1089/ast.2017.1718 . ISSN 1531-1074 . ПМИД 30010383 . S2CID 51628150 .
- Лингам, Манасви; Леб, Авраам (август 2018 г.). «Ограничения химического движения для межзвездного побега из обитаемых зон вокруг звезд малой массы» . Исследовательские записки ААС . 2 (3): 154. arXiv : 1808.08141 . Бибкод : 2018RNAAS...2..154L . дои : 10.3847/2515-5172/aadcf4 . ISSN 2515-5172 . S2CID 119470444 .
- Лингам, Манасви; Леб, Авраам (11 июня 2019 г.). «Коллоквиум: Физические ограничения эволюции жизни на экзопланетах». Обзоры современной физики . 91 (2): 021002. arXiv : 1810.02007 . Бибкод : 2019RvMP...91b1002L . дои : 10.1103/RevModPhys.91.021002 . S2CID 85501199 .
- Лингам, Манасви; Леб, Авраам (1 июня 2019 г.). «Фотосинтез на обитаемых планетах вокруг маломассивных звезд» . Ежемесячные уведомления Королевского астрономического общества . 485 (4): 5924–5928. arXiv : 1901.01270 . Бибкод : 2019MNRAS.485.5924L . дои : 10.1093/mnras/stz847 . ISSN 1365-2966 . S2CID 84843940 .
- Лингам, Манасви; Леб, Авраам (апрель 2019 г.). «Подповерхностная экзожизнь». Международный журнал астробиологии . 18 (2): 112–141. arXiv : 1711.09908 . Бибкод : 2019IJAsB..18..112L . дои : 10.1017/S1473550418000083 . ISSN 1475-3006 . S2CID 102480854 .
- Лингам, Манасви; Леб, Ави (21 июня 2021 г.). Жизнь в Космосе . Издательство Гарвардского университета. дои : 10.4159/9780674259959 . ISBN 978-0-674-25995-9 . S2CID 242834912 .
- Лински, Джеффри (2019). Звезды-хозяева и их влияние на атмосферу экзопланеты: вводный обзор . Конспект лекций по физике. Том. 955. Международное издательство Спрингер. дои : 10.1007/978-3-030-11452-7 . ISBN 978-3-030-11451-0 . S2CID 181923774 .
- Лю, Бэйбэй; Цзи, Цзянхуэй (октябрь 2020 г.). «Повесть о формировании планет: от пыли к планетам». Исследования в области астрономии и астрофизики . 20 (10): 164. arXiv : 2009.02321 . Бибкод : 2020RAA....20..164L . дои : 10.1088/1674-4527/20/10/164 . S2CID 221507902 .
- Люгер, Родриго; Шестович, Марко; Круз, Итан; Гримм, Саймон Л.; Демори, Брис-Оливье; Агол, Эрик; Болмонт, Эмелин; Фабрики, Дэниел; Фернандес, Катарина С.; Ван Гроотель, Валери; Бургассер, Адам; Гиллон, Майкл; Ингаллс, Джеймс Г.; Жехин, Эммануэль; Раймонд, Шон Н.; Цельсис, Франк; Трио, Амори HMJ; Барклай, Томас; Баренц, Герт; Хауэлл, Стив Б.; Дельрес, Летиция; де Вит, Жюльен; Форман-Макки, Дэниел; Холдсворт, Дэниел Л.; Леконт, Джереми; Ледерер, Сьюзен; Тюрбет, Мартин; Алмлики, Ясин; Бенхалдун, Зухайр; Магейн, Пьер; Моррис, Бретт М.; Хенг, Кевин; Кело, Дидье (22 мая 2017 г.). «Резонансная цепочка из семи планет в TRAPPIST-1». Природная астрономия . 1 (6): 0129. arXiv : 1703.04166 . Бибкод : 2017НатАс...1Е.129Л . дои : 10.1038/s41550-017-0129 . ISSN 2397-3366 . S2CID 54770728 .
- Мадхусудхан, Никку (18 августа 2019 г.). «Экзопланетные атмосферы: ключевые идеи, проблемы и перспективы». Ежегодный обзор астрономии и астрофизики . 57 (1): 617–663. arXiv : 1904.03190 . Бибкод : 2019ARA&A..57..617M . doi : 10.1146/annurev-astro-081817-051846 . ISSN 0066-4146 . S2CID 102350577 .
- Мадхусудхан, Никку (2020). Экзофронтьеры: большие вопросы экзопланетной науки . Издательство ИОП. ISBN 978-0-7503-1472-5 . OCLC 1285004266 .
- Мальтальяти, Лука (27 марта 2017 г.). «Экзопланеты: почему нас должен волновать TRAPPIST-1?». Природная астрономия . 1 (4): 0104. Бибкод : 2017НатАс...1Е.104М . дои : 10.1038/s41550-017-0104 . ISSN 2397-3366 . S2CID 125667140 .
- Марино, С.; Вятт, MC; Кеннеди, генеральный директор; Кама, М.; Матра, Л.; Трио, AHMJ; Хеннинг, Т. (11 марта 2020 г.). «Поиски пылевого кометного пояса вокруг TRAPPIST-1 с помощью ALMA» . Ежемесячные уведомления Королевского астрономического общества . 492 (4): 6067–6073. arXiv : 1909.09158 . Бибкод : 2020MNRAS.492.6067M . дои : 10.1093/mnras/staa266 . ISSN 1365-2966 . S2CID 202712440 .
- Marov, M. Ya.; Shevchenko, I. I. (September 2020). "Exoplanets: nature and models". Physics-Uspekhi . 63 (9): 837–871. Bibcode : 2020PhyU...63..837M . doi : 10.3367/ufne.2019.10.038673 . ISSN 1063-7869 . S2CID 209965726 .
- Мартин, Ребекка Г.; Ливио, Марио (1 февраля 2022 г.). «Астероиды и жизнь: насколько особенна Солнечная система?» . Письма астрофизического журнала . 926 (2): Л20. arXiv : 2202.01352 . Бибкод : 2022ApJ...926L..20M . дои : 10.3847/2041-8213/ac511c . S2CID 246485608 .
- Мартинес-Родригес, Гектор; Найт, Джозеф Энтони; Сифуэнтес, Чарльз; Пиро, Энтони Л.; Барнс, Рори (26 декабря 2019 г.). «Экзуны в обитаемых зонах М-карликов » Астрофизический журнал . 887 (2): 261. arXiv : 1910.12054 . Бибкод : 2019ApJ... 887..261M дои : 10.3847/1538–4357/ab5640 . S2CID 204904780 .
- Макдонаф, Уильям Ф.; Ёсидзаки, Такаши (2 июля 2021 г.). «Состав планет земной группы, контролируемый магнитным полем аккреционного диска» . Прогресс в науке о Земле и планетах . 8 (1): 39. Бибкод : 2021PEPS....8...39M . дои : 10.1186/s40645-021-00429-4 . ISSN 2197-4284 . S2CID 235701559 .
- Маккей, Тристан (2021). Семиотический подход к открытым нотациям: двусмысленность как возможность . Элементы музыки с 1945 года. Издательство Кембриджского университета. ISBN 978-1-108-81332-7 .
- Медоуз, Виктория С.; Шмидт, Бритни Э. (2020). Планетарная астробиология . Издательство Университета Аризоны. ISBN 978-0-8165-4006-8 . OCLC 1096534611 .
- Медоуз, Виктория С.; Арни, Джада Н.; Швитерман, Эдвард В.; Люстиг-Йегер, Джейкоб; Линковски, Эндрю П.; Робинсон, Тайлер; Домагал-Голдман, Шон Д.; Дейтрик, Рассел; Барнс, Рори К.; Флеминг, Дэвид П.; Люгер, Родриго; Дрисколл, Питер Э.; Куинн, Томас Р.; Крисп, Дэвид (1 февраля 2018 г.). «Обитаемость Проксимы Центавра b: состояние окружающей среды и дискриминанты наблюдений» . Астробиология . 18 (2): 133–189. arXiv : 1608.08620 . Бибкод : 2018AsBio..18..133M . дои : 10.1089/ast.2016.1589 . ISSN 1531-1074 . ПМК 5820795 . ПМИД 29431479 .
- Майлз-Паес, Пенсильвания; Сапатеро Осорио, MR; Палле, Э.; Метчев, С.А. (21 марта 2019 г.). «Поляриметрия изображений с временным разрешением TRAPPIST-1 во время транзитов планет» . Ежемесячные уведомления Королевского астрономического общества: письма . 484 (1): L38–L42. arXiv : 1901.02041 . Бибкод : 2019MNRAS.484L..38M . дои : 10.1093/mnrasl/slz001 . ISSN 1745-3925 . S2CID 119095657 .
- Морли, Кэролайн В.; Крейдберг, Лаура; Рустамкулов, Зафар; Робинсон, Тайлер; Фортни, Джонатан Дж. (22 ноября 2017 г.). «Наблюдение за атмосферами известных планет размером с Землю с умеренным климатом с помощью JWST» . Астрофизический журнал . 850 (2): 121. arXiv : 1708.04239 . Бибкод : 2017ApJ...850..121M . дои : 10.3847/1538-4357/aa927b .
- Моррис, Бретт М.; Агол, Эрик; Давенпорт, Джеймс Р.А.; Хоули, Сюзанна Л. (11 апреля 2018 г.). «Возможные яркие звездные пятна на TRAPPIST-1» . Астрофизический журнал . 857 (1): 39. arXiv : 1803.04543 . Бибкод : 2018ApJ...857...39M . дои : 10.3847/1538-4357/aab6a5 . S2CID 55891098 .
- Моррис, Бретт М.; Агол, Эрик; Хебб, Лесли; Хоули, Сюзанна Л.; Гиллон, Майкл; Дюкро, Эльза; Дельрес, Летиция; Ингаллс, Джеймс; Демори, Брис-Оливье (17 августа 2018 г.). «Отсутствие обнаружения загрязнения звездной активностью на кривых транзитного блеска Спитцера TRAPPIST-1» . Письма астрофизического журнала . 863 (2): Л32. arXiv : 1808.02808 . Бибкод : 2018ApJ...863L..32M . дои : 10.3847/2041-8213/aad8aa . S2CID 53332500 .
- Муллан, диджей; Байс, HP (27 сентября 2018 г.). «Фотосинтез на планете, вращающейся вокруг М-карлика: повышенная эффективность во время вспышек» . Астрофизический журнал . 865 (2): 101. arXiv : 1807.05267 . Бибкод : 2018ApJ...865..101M . дои : 10.3847/1538-4357/aadfd1 . S2CID 119073856 .
- Муллан, диджей; Паудель, Р.Р. (27 февраля 2019 г.). «Происхождение радиотихих корональных выбросов массы во вспыхивающих звездах» . Астрофизический журнал . 873 (1): 1. arXiv : 1902.00810 . Бибкод : 2019ApJ...873....1M . дои : 10.3847/1538-4357/ab041b . S2CID 119420075 .
- «Определение Теслы» . Национальная лаборатория сильных магнитных полей . 18 ноября 2022 г. Проверено 16 мая 2023 г.
- Наварро, Томас; Мерлис, Тимоти М.; Коуэн, Николас Б.; Гомес, Наталья (15 июля 2022 г.). «Атмосферные гравитационные приливы планет земного типа, вращающихся вокруг маломассивных звезд» . Планетарный научный журнал . 3 (7): 162. arXiv : 2207.06974 . Бибкод : 2022PSJ.....3..162N . дои : 10.3847/PSJ/ac76cd . ISSN 2632-3338 . S2CID 250526799 .
- Огихара, Масахиро; Кокубо, Эйитиро; Накано, Рюуносукэ; Сузуки, Такеру К. (1 февраля 2022 г.). «Быстрая, затем медленная миграция воспроизводит массовое распространение системы TRAPPIST-1» . Астрономия и астрофизика . 658 : А184. arXiv : 2201.08840 . Бибкод : 2022A&A...658A.184O . дои : 10.1051/0004-6361/202142354 . ISSN 0004-6361 . S2CID 246210342 .
- О'Мэлли-Джеймс, Джек Т.; Калтенеггер, Л. (июль 2017 г.). «УФ-обитаемость поверхности системы TRAPPIST-1» . Ежемесячные уведомления Королевского астрономического общества: письма . 469 (1): L26–L30. arXiv : 1702.06936 . дои : 10.1093/mnrasl/slx047 . ISSN 1745-3933 .
- О'Мэлли-Джеймс, Джек Т.; Кальтенеггер, Лиза (1 октября 2019 г.). «Биофлуоресцентные миры – II. Биологическая флуоресценция, вызванная звездными УФ-вспышками, новая временная биосигнатура» . Ежемесячные уведомления Королевского астрономического общества . 488 (4): 4530–4545. arXiv : 1608.06930 . дои : 10.1093/mnras/stz1842 . ISSN 1365-2966 .
- Ормел, Крис В.; Лю, Бэйбэй; Шуненберг, Джоке (1 августа 2017 г.). «Формирование TRAPPIST-1 и других компактных систем». Астрономия и астрофизика . 604 : А1. arXiv : 1703.06924 . Бибкод : 2017A&A...604A...1O . дои : 10.1051/0004-6361/201730826 . ISSN 0004-6361 . S2CID 4606360 .
- Паладини, Стефания (2019). Новые границы космоса: экономические последствия, проблемы безопасности и развивающиеся сценарии . Спрингер. ISBN 978-3-030-19941-8 .
- «Большая идея Черчилля». Мир физики . 30 (4): 3 апреля 2017 г. doi : 10.1088/2058-7058/30/4/1 . ISSN 2058-7058 .
- Подгородецкая Дарья; Фошез, Томас Дж.; Вильянуэва, Джеронимо Л.; Домагал-Голдман, Шон Д.; Коппарапу, Рави К. (июль 2020 г.). «Обнаруживаемость молекулярных сигнатур на TRAPPIST-1e с помощью трансмиссионной спектроскопии, смоделированной для будущих космических обсерваторий» . Письма астрофизического журнала . 898 (2): Л33. arXiv : 2001.01338 . Бибкод : 2020ApJ...898L..33P . дои : 10.3847/2041-8213/aba4a1 . hdl : 11603/20595 . S2CID 209862793 .
- Пьеррембер, Раймонд Т.; Хаммонд, Марк (5 января 2019 г.). «Атмосферная циркуляция экзопланет, заблокированных приливами» . Ежегодный обзор механики жидкости . 51 (1): 275–303. Бибкод : 2019АнРФМ..51..275П . doi : 10.1146/annurev-fluid-010518-040516 . ISSN 0066-4189 . S2CID 125645319 .
- Пинчук, Павел; Марго, Жан-Люк; Гринберг, Адам Х.; Аялде, Томас; Блоксэм, Чад; Бодду, Арджун; Шиншилла-Гарсия, Луис Херардо; Клифф, Мика; Галлахер, Сара; Харт, Кира; Хесфорд, Брайден; Мизрахи, Инбал; Пайк, Рут; Роджер, Доминик; Сайки, Баде; Шнек, Уна; Тан, Айсен; Сяо, Иньсюэ «Иоланда»; Линч, Райан С. (19 февраля 2019 г.). «Поиск техносигнатур TRAPPIST-1, LHS 1140 и 10 планетных систем в поле Кеплера с помощью телескопа Грин-Бэнк на частоте 1,15–1,73 ГГц» . Астрономический журнал . 157 (3): 122. arXiv : 1901.04057 . Бибкод : 2019AJ....157..122P . дои : 10.3847/1538-3881/ab0105 . S2CID 113397518 .
- Пинеда, Дж. Себастьян; Халлинан, Грегг (24 октября 2018 г.). «Глубокий предел радиосвязи для системы TRAPPIST-1» . Астрофизический журнал . 866 (2): 155. arXiv : 1806.00480 . Бибкод : 2018ApJ...866..155P . дои : 10.3847/1538-4357/aae078 . S2CID 119209821 .
- Быстрая, Линн С.; Роберж, Аки; Млинар, Эми Барр; Хедман, Мэтью М. (август 2020 г.). «Прогнозирование темпов вулканической активности на земных экзопланетах и последствия для криовулканической активности на внесолнечных океанских мирах» . Публикации Тихоокеанского астрономического общества . 132 (1014): 084402. Бибкод : 2020PASP..132h4402Q . дои : 10.1088/1538-3873/ab9504 . ISSN 0004-6280 . S2CID 219964895 .
- Быстрая, Линн С.; Роберж, Аки; Мендоса, Гваделупе Товар; Кинтана, Элиза В.; Янгблад, Эллисон А. (1 октября 2023 г.). «Перспективы криовулканической активности на планетах холодного океана» . Астрофизический журнал . 956 (1): 29. Бибкод : 2023ApJ...956...29Q . дои : 10.3847/1538-4357/ace9b6 .
- Радноти, Каталин (1 мая 2021 г.). «Экзопланеты на уроках физики» . Физический журнал: серия конференций . 1929 (1): 012015. Бибкод : 2021JPhCS1929a2015R . дои : 10.1088/1742-6596/1929/1/012015 . ISSN 1742-6596 . S2CID 235591431 .
- Ранджан, Сукрит; Вордсворт, Робин; Саселов, Димитар Д. (11 июля 2017 г.). «Поверхностная УФ-среда на планетах, вращающихся вокруг M-карликов: значение для химии пребиотиков и необходимость последующих экспериментальных исследований» . Астрофизический журнал . 843 (2): 110. arXiv : 1705.02350 . Бибкод : 2017ApJ...843..110R . дои : 10.3847/1538-4357/aa773e . S2CID 119502156 .
- Раймонд, Шон Н.; Изидоро, Андре; Болмонт, Эмелин; Дорн, Кэролайн; Селсис, Франк; Тюрбет, Мартин; Агол, Эрик; Барт, Патрик; Кароне, Людмила; Дасгупта, Радждип; Гиллон, Майкл; Гримм, Саймон Л. (25 ноября 2021 г.). «Верхний предел поздней аккреции и доставки воды в экзопланетной системе TRAPPIST-1». Природная астрономия . 6 : 80–88. arXiv : 2111.13351 . дои : 10.1038/s41550-021-01518-6 . ISSN 2397-3366 . S2CID 244668317 .
- «Красный карлик» . КОСМОС — Астрономическая энциклопедия САО . Суинбернский технологический университет . Проверено 31 июля 2022 г.
- Рибер, Адриан Гарсия (июнь 2018 г.). ПЛАНЕТЕЗАЙЗЕР: КОНЦЕРТ СОНИФИКАЦИИ (PDF) . 24-я Международная конференция по слуховому дисплею (ICAD 2018). Мичиганский технологический университет .
- Ринальди, Дэвид; Нуньес Феррер, Хорхе (март 2017 г.). «Привет новой солнечной системе – и инвестиционной стратегии ЕС. Комментарий CEPS, 7 марта 2017 г.» . СЕПС .
- Реттенбахер, Рэйчел М.; Кейн, Стивен Р. (14 декабря 2017 г.). «Звездная активность TRAPPIST-1 и последствия для планетарных атмосфер» . Астрофизический журнал . 851 (2): 77. arXiv : 1711.02676 . Бибкод : 2017ApJ...851...77R . дои : 10.3847/1538-4357/aa991e . S2CID 73535657 .
- Рашби, Эндрю Дж.; Шилдс, Аомава Л.; Вольф, Эрик Т.; Лаге, Мариса; Бургассер, Адам (26 ноября 2020 г.). «Влияние альбедо суши на климат планет с преобладанием суши в системе TRAPPIST-1» . Астрофизический журнал . 904 (2): 124. arXiv : 2011.03621 . Бибкод : 2020ApJ...904..124R . дои : 10.3847/1538-4357/abbe04 . S2CID 226281770 .
- Сакауэ, Такахито; Сибата, Казунари (1 сентября 2021 г.). «Связь хромосферы, короны и ветра М-карлика через нелинейные альфвеновские волны» . Астрофизический журнал . 919 (1): 29. arXiv : 2106.12752 . Бибкод : 2021ApJ...919...29S . дои : 10.3847/1538-4357/ac0e34 . S2CID 235624132 .
- Самара, Евангелия; Патсуракос, Спирос; Георгулис, Манолис К. (1 марта 2021 г.). «Легко реализуемое ограничение устойчивости атмосферы для экзопланет земной группы, вращающихся вокруг магнитно активных звезд» . Письма астрофизического журнала . 909 (1): Л12. arXiv : 2102.07837 . Бибкод : 2021ApJ...909L..12S . дои : 10.3847/2041-8213/abe416 . S2CID 231933691 .
- Шлихтинг, Хильке Э.; Янг, Эдвард Д. (1 мая 2022 г.). «Химическое равновесие между ядрами, мантией и атмосферами суперземель и субнептунов и последствия для их состава, внутренней части и эволюции» . Планетарный научный журнал . 3 (5): 127. arXiv : 2107.10405 . Бибкод : 2022PSJ.....3..127S . дои : 10.3847/psj/ac68e6 . ISSN 2632-3338 . S2CID 236171388 .
- Шнайдер, Дж.; Дедье, К.; Сиданер, П. Ле; Саваль, Р.; Золотухин И. (1 августа 2011 г.). «Определение и каталогизация экзопланет: база данных exoplanet.eu» . Астрономия и астрофизика . 532 : А79. arXiv : 1106.0586 . Бибкод : 2011A&A...532A..79S . дои : 10.1051/0004-6361/201116713 . ISSN 0004-6361 . S2CID 55994657 .
- Швитерман, Эдвард В.; Рейнхард, Кристофер Т.; Олсон, Стефани Л.; Харман, Честер Э.; Лайонс, Тимоти В. (10 июня 2019 г.). «Ограниченная обитаемая зона для сложной жизни» . Астрофизический журнал . 878 (1): 19. arXiv : 1902.04720 . Бибкод : 2019ApJ...878...19S . дои : 10.3847/1538-4357/ab1d52 . S2CID 118948604 .
- Шайденбергер, Кристоф; Пфюцнер, Марек, ред. (2018). Еврошкола по экзотическим балкам - Том. 5 . Конспект лекций по физике. Том. 948. дои : 10.1007/978-3-319-74878-8 . ISBN 978-3-319-74878-8 . S2CID 220615062 .
- Сейн, Александр; Дункан, Колтон; Чжун, Патрик; Кук, Элиза; Ли, Вэйлон; Якубик, Коннор; МакГенри, Нил; Бруно, Эшли; Чамитов, Григорий (2021). «Образование STEM посредством конкурсов по проектированию виртуальных космических систем». Форум AIAA Scitech 2021 . Форум AIAA Scitech 2021. Американский институт аэронавтики и астронавтики. дои : 10.2514/6.2021-0481 . ISBN 978-1-62410-609-5 . S2CID 234272238 .
- Шилдс, Аомава Л.; Баллард, Сара; Джонсон, Джон Ашер (5 декабря 2016 г.). «Обитаемость планет, вращающихся вокруг звезд М-карликов». Отчеты по физике . 663 : 1–38. arXiv : 1610.05765 . Бибкод : 2016ФР...663....1С . дои : 10.1016/j.physrep.2016.10.003 . ISSN 0370-1573 . S2CID 119248081 .
- Шилдс, Аомава Л.; Карнс, Регина К. (25 октября 2018 г.). «Обратная связь гидрогалитовой соли и альбедо может охладить М-карликовые планеты» . Астрофизический журнал . 867 (1): 11. arXiv : 1808.09977 . Бибкод : 2018ApJ...867...11S . дои : 10.3847/1538-4357/aadcaa . S2CID 76652437 .
- Коротко, Кендра; Стапельфельдт, Карл (2017). Обновление программы исследования экзопланеты (PDF) (Отчет). Программа НАСА по исследованию экзопланет . Архивировано из оригинала (PDF) 9 декабря 2021 года.
- Слитор, Рой Д.; Смит, Найл (4 мая 2017 г.). «ТРАППИСТ-1: Заря эпохи Водолея» . Биоинженерия . 8 (3): 194–195. дои : 10.1080/21655979.2017.1306998 . ISSN 2165-5979 . ПМК 5470511 . ПМИД 28324663 .
- Снеллен, Ignas AG (февраль 2017 г.). «Семь сестер Земли». Природа . 542 (7642): 421–422. дои : 10.1038/542421а . hdl : 1887/75076 . ISSN 1476-4687 . ПМИД 28230129 . S2CID 205092857 .
- Шринивас, Сушила (август 2017 г.). «Есть ли там обитаемые миры? - В поисках экзопланет» (PDF) . Научный репортер . 54 (8): 14–20. ISSN 0036-8512 .
- Стивенсон, Дэвид С. (2019). Красные карлики: их геологический, химический и биологический потенциал для жизни . Международное издательство Спрингер. дои : 10.1007/978-3-030-25550-3 . ISBN 978-3-030-25549-7 . S2CID 203546646 .
- Тейшейра, Кэти Э.; Морли, Кэролайн В.; Фоли, Брэдфорд Дж.; Унтерборн, Кайман Т. (декабрь 2023 г.). «Эволюция TRAPPIST-1c с дефицитом углерода» . Астрофизический журнал . 960 (1): 44. arXiv : 2311.17699 . дои : 10.3847/1538-4357/ad0cec . ISSN 0004-637X .
- Тюрбет, Мартин; Болмонт, Эмелин; Леконт, Джереми; Забудь, Франсуа; Селсис, Франк; Тоби, Габриэль; Калдас, Энтони; Наар, Джозеф; Гиллон, Майкл (1 апреля 2018 г.). «Моделирование разнообразия климата, приливной динамики и судьбы летучих веществ на планетах TRAPPIST-1». Астрономия и астрофизика . 612 : А86. arXiv : 1707.06927 . Бибкод : 2018A&A...612A..86T . дои : 10.1051/0004-6361/201731620 . ISSN 0004-6361 . S2CID 53990543 .
- Тюрбет, Мартин; Болмонт, Эмелин; Бурье, Винсент; Демори, Брис-Оливье; Леконт, Жереми; Оуэн, Джеймс; Вольф, Эрик Т. (август 2020 г.). «Обзор возможных планетарных атмосфер в системе TRAPPIST-1» . Обзоры космической науки . 216 (5): 100. arXiv : 2007.03334 . Бибкод : 2020ССРв..216..100Т . дои : 10.1007/s11214-020-00719-1 . ISSN 1572-9672 . ПМЦ 7378127 . ПМИД 32764836 .
- Ван, Джесси (1 июня 2022 г.). «Закон гравитации, размытый возмущенными планетарными орбитами для инопланетных наблюдателей» . Физический журнал: серия конференций . 2287 (1): 012039. Бибкод : 2022JPhCS2287a2039W . дои : 10.1088/1742-6596/2287/1/012039 . ISSN 1742-6596 . S2CID 250290787 .
- Уитли, Питер Дж.; Лауден, Том; Бурье, Винсент; Эренрайх, Дэвид; Гиллон, Майкл (11 февраля 2017 г.). «Сильное XUV-облучение экзопланет размером с Землю, вращающихся вокруг ультрахолодного карлика TRAPPIST-1» . Ежемесячные уведомления Королевского астрономического общества: письма . 465 (1): L74–L78. arXiv : 1605.01564 . Бибкод : 2017MNRAS.465L..74W . дои : 10.1093/mnrasl/slw192 . ISSN 1745-3933 . S2CID 30087787 .
- Уилсон, Дэвид Дж.; Фронинг, Синтия С.; Дуввури, Гириш М.; Франция, Кевин; Янгблад, Эллисон; Шнайдер, П. Кристиан; Берта-Томпсон, Закори; Браун, Александр; Буччино, Андреа П.; Хоули, Сюзанна; Ирвин, Джонатан; Кальтенеггер, Лиза; Ковальски, Адам; Лински, Джеффри; Парк Лойд, Род-Айленд; Мигель, Ямила; Пинеда, Дж. Себастьян; Редфилд, Сет; Роберж, Аки; Ругхаймер, Сара; Тянь, Фэн; Виетес, Мариэла (1 апреля 2021 г.). «Спектральное распределение энергии TRAPPIST-1 в МЕГА-МЫШЦАХ» . Астрофизический журнал . 911 (1): 18. arXiv : 2102.11415 . Бибкод : 2021ApJ...911...18W . дои : 10.3847/1538-4357/abe771 . S2CID 232014177 .
- Вольф, Эрик Т. (6 апреля 2017 г.). «Оценка обитаемости системы TRAPPIST-1 с использованием 3D-модели климата» . Письма астрофизического журнала . 839 (1): Л1. arXiv : 1703.05815 . Бибкод : 2017ApJ...839L...1W . дои : 10.3847/2041-8213/aa693a . S2CID 119082049 .
- Вундерлих, Фабиан; Шойхер, Маркус; Годольт, М.; Гренфелл, Дж.Л.; Шрайер, Ф.; Шнайдер, ПК; Уилсон, диджей; Санчес-Лопес, А.; Лопес-Пуэртас, М.; Рауэр, Х. (29 сентября 2020 г.). «Различие влажной и сухой атмосферы TRAPPIST-1 e и f» . Астрофизический журнал . 901 (2): 126. arXiv : 2006.11349 . Бибкод : 2020ApJ...901..126W . дои : 10.3847/1538-4357/aba59c . S2CID 219966834 .
- Валио, Адриана; Эстрела, Раиса; Кабрал, Луиза; Гранжейро, Абель (август 2018 г.). «Биологическое воздействие супервспышек на планеты в обитаемой зоне». Труды Международного астрономического союза . 14 (С345): 176–180. дои : 10.1017/S1743921319002035 . ISSN 1743-9213 . S2CID 216905441 .
- Ван Холст, Тим; Ноак, Лена; Ривольдини, Аттилио (1 января 2019 г.). «Интерьеры и обитаемость экзопланет» . Достижения физики: X . 4 (1): 1630316. Бибкод : 2019AdPhX...430316V . дои : 10.1080/23746149.2019.1630316 . S2CID 198417434 .
- Верас, Дмитрий; Бридт, Эльме (1 июля 2017 г.). «Геометрия затмений, транзитов и затмений планетных систем в экзосизигии» . Ежемесячные уведомления Королевского астрономического общества . 468 (3): 2672–2683. arXiv : 1703.03414 . дои : 10.1093/mnras/stx614 . ISSN 1365-2966 .
- Вида, Кристиан; Ковари, Жолт; Пал, Андраш; Ола, Каталин; Крискович, Левенте (2 июня 2017 г.). «Частое сжигание в системе TRAPPIST-1 – непригодно для жизни?» . Астрофизический журнал . 841 (2): 124. arXiv : 1703.10130 . Бибкод : 2017ApJ...841..124В . дои : 10.3847/1538-4357/aa6f05 . S2CID 118827117 .
- Винсон, Алек М.; Тамайо, Дэниел; Хансен, Брэд М.С. (1 августа 2019 г.). «Хаотическая природа состояний планетарного вращения TRAPPIST-1» . Ежемесячные уведомления Королевского астрономического общества . 488 (4): 5739–5747. arXiv : 1905.11419 . Бибкод : 2019MNRAS.488.5739V . дои : 10.1093/mnras/stz2113 . ISSN 1365-2966 . S2CID 167217467 .
- Ян, Дж.; Джи, В. (1 декабря 2018 г.). Proxima b, TRAPPIST 1e и LHS 1140b: Увеличение ледового покрова за счет динамики морского льда . Американский геофизический союз, осеннее собрание 2018 г. Тезисы осеннего собрания AGU . Том. 2018. Вашингтон, округ Колумбия . стр. P43G–3826. Бибкод : 2018AGUFM.P43G3826Y .
- Занацци, Джей Джей; Лай, Донг (11 августа 2017 г.). «Трехосная деформация и асинхронное вращение каменистых планет в зоне обитаемости звезд малой массы» . Ежемесячные уведомления Королевского астрономического общества . 469 (3): 2879–2885. arXiv : 1702.07327 . Бибкод : 2017MNRAS.469.2879Z . дои : 10.1093/mnras/stx1076 . ISSN 1365-2966 . S2CID 119430179 .
- Занацци, Джей Джей; Трио, Амори HMJ (1 июня 2019 г.). «Способность значительного приливного стресса инициировать тектонику плит». Икар . 325 : 55–66. arXiv : 1711.09898 . Бибкод : 2019Icar..325...55Z . дои : 10.1016/j.icarus.2019.01.029 . ISSN 0019-1035 . S2CID 96450290 .
- Чжан, Си (июль 2020 г.). «Атмосферные режимы и тенденции на экзопланетах и коричневых карликах». Исследования в области астрономии и астрофизики . 20 (7): 099. arXiv : 2006.13384 . Бибкод : 2020RAA....20...99Z . дои : 10.1088/1674-4527/20/7/99 . ISSN 1674-4527 . S2CID 220042096 .
- Чжан, Чжанбо; Чжоу, Ифань; Рэкхэм, Бенджамин В.; Апай, Даниэль (4 октября 2018 г.). «Спектры пропускания ближнего инфракрасного диапазона планет TRAPPIST-1 b, c, d, e, f и g и звездное загрязнение в многоэпохальных транзитных спектрах» . Астрономический журнал . 156 (4): 178. arXiv : 1802.02086 . Бибкод : 2018AJ....156..178Z . дои : 10.3847/1538-3881/aade4f . hdl : 10150/631598 . S2CID 118938032 .
- Грин, Томас П.; Белл, Тейлор Дж.; Дюкро, Эльза; Дайрек, Акрена; Лагаж, Пьер-Оливье; Фортни, Джонатан Дж. (март 2023 г.). «Тепловое излучение экзопланеты размером с Землю TRAPPIST-1 b с использованием JWST». Природа . 618 (7963): 39–42. arXiv : 2303.14849 . Бибкод : 2023Natur.618...39G . дои : 10.1038/s41586-023-05951-7 . ПМИД 36972683 . S2CID 257767242 .
Дальнейшее чтение
[ редактировать ]- Арканд, Кимберли К.; Прайс, Сара Р.; Вацке, Меган (2020). «Держа космос в своих руках: разработка трубопроводов 3D-моделирования и печати для коммуникаций и исследований» . Границы в науках о Земле . 8 : 541. arXiv : 2012.02789 . Бибкод : 2020FrEaS...8..541A . дои : 10.3389/feart.2020.590295 . ISSN 2296-6463 .
- Дзомбета, Крстинья; Перси, Джон (31 октября 2019 г.). Вспыхивающие звезды: краткий обзор (отчет).
- Фошез, Томас Дж.; Тюрбет, Мартин; Вольф, Эрик Т.; Бутл, Ян; Путь, Майкл Дж.; Дель Дженио, Энтони Д.; Мейн, Натан Дж.; Цигаридис, Константинос; Коппарапу, Рави К.; Ян, Цзюнь; Забудь, Франсуа; Манделл, Ави; Домагал Голдман, Шон Д. (21 февраля 2020 г.). «Взаимное сравнение обитаемой атмосферы TRAPPIST-1 (THAI): мотивы и версия протокола 1.0» . Разработка геонаучной модели . 13 (2): 707–716. arXiv : 2002.10950 . Бибкод : 2020GMD....13..707F . doi : 10.5194/gmd-13-707-2020 . ISSN 1991-959Х . S2CID 211296491 .
Внешние ссылки
[ редактировать ]
- «Официальный сайт команды открытия» . ТРАППИСТ.one .
- «Ультракрутой карлик с планетами» . ESOcast 83. Европейская южная обсерватория.