Галактика
Галактика – это система звезд , остатков звезд , межзвездного газа , пыли и темной материи, связанных между собой гравитацией . [1] [2] Это слово происходит от греческого слова «галаксия» ( γαλαξίας ), буквально «млечный», отсылка к галактике Млечный Путь , в которой находится Солнечная система . Галактики, насчитывающие в среднем около 100 миллионов звезд, [3] по размеру варьируются от карликов с менее чем тысячей звезд, [4] до крупнейших известных галактик – сверхгигантов со ста триллионами своей галактики звезд, каждая из которых вращается вокруг центра масс . Большая часть массы типичной галактики находится в форме темной материи , и лишь несколько процентов этой массы видимы в виде звезд и туманностей. Сверхмассивные черные дыры — обычное явление в центрах галактик.
галактики подразделяются В зависимости от визуальной морфологии на эллиптические , [5] спиральная или неправильная . [6] Млечный Путь является примером спиральной галактики. По оценкам, существует около 200 млрд. [7] ( 2 × 10 11 ) до 2 триллионов [8] galaxies in the observable universe. Most galaxies are 1,000 to 100,000 parsecs in diameter (approximately 3,000 to 300,000 light years) and are separated by distances in the order of millions of parsecs (or megaparsecs). For comparison, the Milky Way has a diameter of at least 26,800 parsecs (87,400 ly)[9][a] and is separated from the Andromeda Galaxy, its nearest large neighbour, by just over 750,000 parsecs (2.5 million ly.)[12]
The space between galaxies is filled with a tenuous gas (the intergalactic medium) with an average density of less than one atom per cubic metre. Most galaxies are gravitationally organised into groups, clusters and superclusters. The Milky Way is part of the Local Group, which it dominates along with the Andromeda Galaxy. The group is part of the Virgo Supercluster. At the largest scale, these associations are generally arranged into sheets and filaments surrounded by immense voids.[13] Both the Local Group and the Virgo Supercluster are contained in a much larger cosmic structure named Laniakea.[14]
Etymology
The word galaxy was borrowed via French and Medieval Latin from the Greek term for the Milky Way, galaxías (kúklos) γαλαξίας (κύκλος)[15][16] 'milky (circle)', named after its appearance as a milky band of light in the sky. In Greek mythology, Zeus places his son, born by a mortal woman, the infant Heracles, on Hera's breast while she is asleep so the baby will drink her divine milk and thus become immortal. Hera wakes up while breastfeeding and then realises she is nursing an unknown baby: she pushes the baby away, some of her milk spills, and it produces the band of light known as the Milky Way.[17][18]
In the astronomical literature, the capitalised word "Galaxy" is often used to refer to the Milky Way galaxy, to distinguish it from the other galaxies in the observable universe. The English term Milky Way can be traced back to a story by Geoffrey Chaucer c. 1380:
See yonder, lo, the Galaxyë
Which men clepeth the Milky Wey,
For hit is whyt.— Geoffrey Chaucer, The House of Fame[16]
Galaxies were initially discovered telescopically and were known as spiral nebulae. Most 18th- to 19th-century astronomers considered them as either unresolved star clusters or anagalactic nebulae, and were just thought of as a part of the Milky Way, but their true composition and natures remained a mystery. Observations using larger telescopes of a few nearby bright galaxies, like the Andromeda Galaxy, began resolving them into huge conglomerations of stars, but based simply on the apparent faintness and sheer population of stars, the true distances of these objects placed them well beyond the Milky Way. For this reason they were popularly called island universes, but this term quickly fell into disuse, as the word universe implied the entirety of existence. Instead, they became known simply as galaxies.[19]
Nomenclature

Millions of galaxies have been catalogued, but only a few have well-established names, such as the Andromeda Galaxy, the Magellanic Clouds, the Whirlpool Galaxy, and the Sombrero Galaxy. Astronomers work with numbers from certain catalogues, such as the Messier catalogue, the NGC (New General Catalogue), the IC (Index Catalogue), the CGCG (Catalogue of Galaxies and of Clusters of Galaxies), the MCG (Morphological Catalogue of Galaxies), the UGC (Uppsala General Catalogue of Galaxies), and the PGC (Catalogue of Principal Galaxies, also known as LEDA). All the well-known galaxies appear in one or more of these catalogues but each time under a different number. For example, Messier 109 (or "M109") is a spiral galaxy having the number 109 in the catalogue of Messier. It also has the designations NGC 3992, UGC 6937, CGCG 269–023, MCG +09-20-044, and PGC 37617 (or LEDA 37617), among others.[20] Millions of fainter galaxies are known by their identifiers in sky surveys such as the Sloan Digital Sky Survey.[21]
Observation history
Milky Way
Greek philosopher Democritus (450–370 BCE) proposed that the bright band on the night sky known as the Milky Way might consist of distant stars.[22]Aristotle (384–322 BCE), however, believed the Milky Way was caused by "the ignition of the fiery exhalation of some stars that were large, numerous and close together" and that the "ignition takes place in the upper part of the atmosphere, in the region of the World that is continuous with the heavenly motions."[23] Neoplatonist philosopher Olympiodorus the Younger (c. 495–570 CE) was critical of this view, arguing that if the Milky Way was sublunary (situated between Earth and the Moon) it should appear different at different times and places on Earth, and that it should have parallax, which it did not. In his view, the Milky Way was celestial.[24]
According to Mohani Mohamed, Arabian astronomer Ibn al-Haytham (965–1037) made the first attempt at observing and measuring the Milky Way's parallax,[25] and he thus "determined that because the Milky Way had no parallax, it must be remote from the Earth, not belonging to the atmosphere."[26] Persian astronomer al-Biruni (973–1048) proposed the Milky Way galaxy was "a collection of countless fragments of the nature of nebulous stars."[27] Andalusian astronomer Avempace (d. 1138) proposed that it was composed of many stars that almost touched one another, and appeared to be a continuous image due to the effect of refraction from sublunary material,[23][28] citing his observation of the conjunction of Jupiter and Mars as evidence of this occurring when two objects were near.[23] In the 14th century, Syrian-born Ibn Qayyim al-Jawziyya proposed the Milky Way galaxy was "a myriad of tiny stars packed together in the sphere of the fixed stars."[29]
Actual proof of the Milky Way consisting of many stars came in 1610 when the Italian astronomer Galileo Galilei used a telescope to study it and discovered it was composed of a huge number of faint stars.[30][31] In 1750, English astronomer Thomas Wright, in his An Original Theory or New Hypothesis of the Universe, correctly speculated that it might be a rotating body of a huge number of stars held together by gravitational forces, akin to the Solar System but on a much larger scale, and that the resulting disk of stars could be seen as a band on the sky from a perspective inside it.[b][33][34] In his 1755 treatise, Immanuel Kant elaborated on Wright's idea about the Milky Way's structure.[35]

The first project to describe the shape of the Milky Way and the position of the Sun was undertaken by William Herschel in 1785 by counting the number of stars in different regions of the sky. He produced a diagram of the shape of the galaxy with the Solar System close to the center.[36][37] Using a refined approach, Kapteyn in 1920 arrived at the picture of a small (diameter about 15 kiloparsecs) ellipsoid galaxy with the Sun close to the center. A different method by Harlow Shapley based on the cataloguing of globular clusters led to a radically different picture: a flat disk with diameter approximately 70 kiloparsecs and the Sun far from the centre.[34] Both analyses failed to take into account the absorption of light by interstellar dust present in the galactic plane; but after Robert Julius Trumpler quantified this effect in 1930 by studying open clusters, the present picture of the Milky Way galaxy emerged.[38]
Distinction from other nebulae
A few galaxies outside the Milky Way are visible on a dark night to the unaided eye, including the Andromeda Galaxy, Large Magellanic Cloud, Small Magellanic Cloud, and the Triangulum Galaxy. In the 10th century, Persian astronomer Abd al-Rahman al-Sufi made the earliest recorded identification of the Andromeda Galaxy, describing it as a "small cloud".[39] In 964, he probably mentioned the Large Magellanic Cloud in his Book of Fixed Stars, referring to "Al Bakr of the southern Arabs",[40] since at a declination of about 70° south it was not visible where he lived. It was not well known to Europeans until Magellan's voyage in the 16th century.[41][40] The Andromeda Galaxy was later independently noted by Simon Marius in 1612.[39]
In 1734, philosopher Emanuel Swedenborg in his Principia speculated that there might be other galaxies outside that were formed into galactic clusters that were minuscule parts of the universe that extended far beyond what could be seen. These views "are remarkably close to the present-day views of the cosmos."[42]In 1745, Pierre Louis Maupertuis conjectured that some nebula-like objects were collections of stars with unique properties, including a glow exceeding the light its stars produced on their own, and repeated Johannes Hevelius's view that the bright spots were massive and flattened due to their rotation.[35]In 1750, Thomas Wright correctly speculated that the Milky Way was a flattened disk of stars, and that some of the nebulae visible in the night sky might be separate Milky Ways.[34][43]
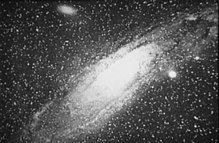
Toward the end of the 18th century, Charles Messier compiled a catalog containing the 109 brightest celestial objects having nebulous appearance. Subsequently, William Herschel assembled a catalog of 5,000 nebulae.[34] In 1845, Lord Rosse examined the nebulae catalogued by Herschel and observed the spiral structure of Messier object M51, now known as the Whirlpool Galaxy.[44][45]
In 1912, Vesto M. Slipher made spectrographic studies of the brightest spiral nebulae to determine their composition. Slipher discovered that the spiral nebulae have high Doppler shifts, indicating that they are moving at a rate exceeding the velocity of the stars he had measured. He found that the majority of these nebulae are moving away from us.[46][47]
In 1917, Heber Doust Curtis observed nova S Andromedae within the "Great Andromeda Nebula", as the Andromeda Galaxy, Messier object M31, was then known. Searching the photographic record, he found 11 more novae. Curtis noticed that these novae were, on average, 10 magnitudes fainter than those that occurred within this galaxy. As a result, he was able to come up with a distance estimate of 150,000 parsecs. He became a proponent of the so-called "island universes" hypothesis, which holds that spiral nebulae are actually independent galaxies.[48]
In 1920 a debate took place between Harlow Shapley and Heber Curtis, the Great Debate, concerning the nature of the Milky Way, spiral nebulae, and the dimensions of the universe. To support his claim that the Great Andromeda Nebula is an external galaxy, Curtis noted the appearance of dark lanes resembling the dust clouds in the Milky Way, as well as the significant Doppler shift.[49]
In 1922, the Estonian astronomer Ernst Öpik gave a distance determination that supported the theory that the Andromeda Nebula is indeed a distant extra-galactic object.[50] Using the new 100-inch Mt. Wilson telescope, Edwin Hubble was able to resolve the outer parts of some spiral nebulae as collections of individual stars and identified some Cepheid variables, thus allowing him to estimate the distance to the nebulae: they were far too distant to be part of the Milky Way.[51] In 1926 Hubble produced a classification of galactic morphology that is used to this day.[52][53]
Multi-wavelength observation
Advances in astronomy have always been driven by technology. After centuries of success in optical astronomy, recent decades have seen major progress in other regions of the electromagnetic spectrum.[54]
The dust present in the interstellar medium is opaque to visual light. It is more transparent to far-infrared, which can be used to observe the interior regions of giant molecular clouds and galactic cores in great detail.[55] Infrared is also used to observe distant, red-shifted galaxies that were formed much earlier. Water vapor and carbon dioxide absorb a number of useful portions of the infrared spectrum, so high-altitude or space-based telescopes are used for infrared astronomy.[56]
The first non-visual study of galaxies, particularly active galaxies, was made using radio frequencies. The Earth's atmosphere is nearly transparent to radio between 5 MHz and 30 GHz. The ionosphere blocks signals below this range.[57] Large radio interferometers have been used to map the active jets emitted from active nuclei.
Ultraviolet and X-ray telescopes can observe highly energetic galactic phenomena. Ultraviolet flares are sometimes observed when a star in a distant galaxy is torn apart from the tidal forces of a nearby black hole.[58] The distribution of hot gas in galactic clusters can be mapped by X-rays. The existence of supermassive black holes at the cores of galaxies was confirmed through X-ray astronomy.[59]
Modern research

In 1944, Hendrik van de Hulst predicted that microwave radiation with wavelength of 21 cm would be detectable from interstellar atomic hydrogen gas;[61] and in 1951 it was observed. This radiation is not affected by dust absorption, and so its Doppler shift can be used to map the motion of the gas in this galaxy. These observations led to the hypothesis of a rotating bar structure in the center of this galaxy.[62] With improved radio telescopes, hydrogen gas could also be traced in other galaxies.In the 1970s, Vera Rubin uncovered a discrepancy between observed galactic rotation speed and that predicted by the visible mass of stars and gas. Today, the galaxy rotation problem is thought to be explained by the presence of large quantities of unseen dark matter.[63][64]
Beginning in the 1990s, the Hubble Space Telescope yielded improved observations. Among other things, its data helped establish that the missing dark matter in this galaxy could not consist solely of inherently faint and small stars.[65] The Hubble Deep Field, an extremely long exposure of a relatively empty part of the sky, provided evidence that there are about 125 billion (1.25×1011) galaxies in the observable universe.[66] Improved technology in detecting the spectra invisible to humans (radio telescopes, infrared cameras, and x-ray telescopes) allows detection of other galaxies that are not detected by Hubble. Particularly, surveys in the Zone of Avoidance (the region of sky blocked at visible-light wavelengths by the Milky Way) have revealed a number of new galaxies.[67]
A 2016 study published in The Astrophysical Journal, led by Christopher Conselice of the University of Nottingham, used 20 years of Hubble images to estimate that the observable universe contained at least two trillion (2×1012) galaxies.[68][69] However, later observations with the New Horizons space probe from outside the zodiacal light reduced this to roughly 200 billion (2×1011).[70][71]
Types and morphology

Galaxies come in three main types: ellipticals, spirals, and irregulars. A slightly more extensive description of galaxy types based on their appearance is given by the Hubble sequence. Since the Hubble sequence is entirely based upon visual morphological type (shape), it may miss certain important characteristics of galaxies such as star formation rate in starburst galaxies and activity in the cores of active galaxies.[6]
Many galaxies are thought to contain a supermassive black hole at their center. This includes the Milky Way, whose core region is called the Galactic Center.[72]
Ellipticals
The Hubble classification system rates elliptical galaxies on the basis of their ellipticity, ranging from E0, being nearly spherical, up to E7, which is highly elongated. These galaxies have an ellipsoidal profile, giving them an elliptical appearance regardless of the viewing angle. Their appearance shows little structure and they typically have relatively little interstellar matter. Consequently, these galaxies also have a low portion of open clusters and a reduced rate of new star formation. Instead, they are dominated by generally older, more evolved stars that are orbiting the common center of gravity in random directions. The stars contain low abundances of heavy elements because star formation ceases after the initial burst. In this sense they have some similarity to the much smaller globular clusters.[73]
Type-cD galaxies

The largest galaxies are the type-cD galaxies.First described in 1964 by a paper by Thomas A. Matthews and others,[74] they are a subtype of the more general class of D galaxies, which are giant elliptical galaxies, except that they are much larger. They are popularly known as the supergiant elliptical galaxies and constitute the largest and most luminous galaxies known. These galaxies feature a central elliptical nucleus with an extensive, faint halo of stars extending to megaparsec scales.[75] The profile of their surface brightnesses as a function of their radius (or distance from their cores) falls off more slowly than their smaller counterparts.[76]
The formation of these cD galaxies remains an active area of research, but the leading model is that they are the result of the mergers of smaller galaxies in the environments of dense clusters, or even those outside of clusters with random overdensities.[77] These processes are the mechanisms that drive the formation of fossil groups or fossil clusters, where a large, relatively isolated, supergiant elliptical resides in the middle of the cluster and are surrounded by an extensive cloud of X-rays as the residue of these galactic collisions. Another older model posits the phenomenon of cooling flow, where the heated gases in clusters collapses towards their centers as they cool, forming stars in the process,[78] a phenomenon observed in clusters such as Perseus,[79] and more recently in the Phoenix Cluster.[80]
Shell galaxy

A shell galaxy is a type of elliptical galaxy where the stars in its halo are arranged in concentric shells. About one-tenth of elliptical galaxies have a shell-like structure, which has never been observed in spiral galaxies. These structures are thought to develop when a larger galaxy absorbs a smaller companion galaxy—that as the two galaxy centers approach, they start to oscillate around a center point, and the oscillation creates gravitational ripples forming the shells of stars, similar to ripples spreading on water. For example, galaxy NGC 3923 has over 20 shells.[81]
Spirals

Spiral galaxies resemble spiraling pinwheels. Though the stars and other visible material contained in such a galaxy lie mostly on a plane, the majority of mass in spiral galaxies exists in a roughly spherical halo of dark matter which extends beyond the visible component, as demonstrated by the universal rotation curve concept.[82]
Spiral galaxies consist of a rotating disk of stars and interstellar medium, along with a central bulge of generally older stars. Extending outward from the bulge are relatively bright arms. In the Hubble classification scheme, spiral galaxies are listed as type S, followed by a letter (a, b, or c) which indicates the degree of tightness of the spiral arms and the size of the central bulge. An Sa galaxy has tightly wound, poorly defined arms and possesses a relatively large core region. At the other extreme, an Sc galaxy has open, well-defined arms and a small core region.[83] A galaxy with poorly defined arms is sometimes referred to as a flocculent spiral galaxy; in contrast to the grand design spiral galaxy that has prominent and well-defined spiral arms.[84] The speed in which a galaxy rotates is thought to correlate with the flatness of the disc as some spiral galaxies have thick bulges, while others are thin and dense.[85][86]

In spiral galaxies, the spiral arms do have the shape of approximate logarithmic spirals, a pattern that can be theoretically shown to result from a disturbance in a uniformly rotating mass of stars. Like the stars, the spiral arms rotate around the center, but they do so with constant angular velocity. The spiral arms are thought to be areas of high-density matter, or "density waves".[87] As stars move through an arm, the space velocity of each stellar system is modified by the gravitational force of the higher density. (The velocity returns to normal after the stars depart on the other side of the arm.) This effect is akin to a "wave" of slowdowns moving along a highway full of moving cars. The arms are visible because the high density facilitates star formation, and therefore they harbor many bright and young stars.[88]

Barred spiral galaxy
A majority of spiral galaxies, including the Milky Way galaxy, have a linear, bar-shaped band of stars that extends outward to either side of the core, then merges into the spiral arm structure.[89] In the Hubble classification scheme, these are designated by an SB, followed by a lower-case letter (a, b or c) which indicates the form of the spiral arms (in the same manner as the categorization of normal spiral galaxies). Bars are thought to be temporary structures that can occur as a result of a density wave radiating outward from the core, or else due to a tidal interaction with another galaxy.[90] Many barred spiral galaxies are active, possibly as a result of gas being channeled into the core along the arms.[91]
Our own galaxy, the Milky Way, is a large disk-shaped barred-spiral galaxy[92] about 30 kiloparsecs in diameter and a kiloparsec thick. It contains about two hundred billion (2×1011)[93] stars and has a total mass of about six hundred billion (6×1011) times the mass of the Sun.[94]
Super-luminous spiral
Recently, researchers described galaxies called super-luminous spirals. They are very large with an upward diameter of 437,000 light-years (compared to the Milky Way's 87,400 light-year diameter). With a mass of 340 billion solar masses, they generate a significant amount of ultraviolet and mid-infrared light. They are thought to have an increased star formation rate around 30 times faster than the Milky Way.[95][96]
Other morphologies
- Peculiar galaxies are galactic formations that develop unusual properties due to tidal interactions with other galaxies.
- A ring galaxy has a ring-like structure of stars and interstellar medium surrounding a bare core. A ring galaxy is thought to occur when a smaller galaxy passes through the core of a spiral galaxy.[97] Such an event may have affected the Andromeda Galaxy, as it displays a multi-ring-like structure when viewed in infrared radiation.[98]
- A lenticular galaxy is an intermediate form that has properties of both elliptical and spiral galaxies. These are categorized as Hubble type S0, and they possess ill-defined spiral arms with an elliptical halo of stars[99] (barred lenticular galaxies receive Hubble classification SB0).
- Irregular galaxies are galaxies that can not be readily classified into an elliptical or spiral morphology.
- An Irr-I galaxy has some structure but does not align cleanly with the Hubble classification scheme.
- Irr-II galaxies do not possess any structure that resembles a Hubble classification, and may have been disrupted.[100] Nearby examples of (dwarf) irregular galaxies include the Magellanic Clouds.[101]
- A dark or "ultra diffuse" galaxy is an extremely-low-luminosity galaxy. It may be the same size as the Milky Way, but have a visible star count only one percent of the Milky Way's. Multiple mechanisms for producing this type of galaxy have been proposed, and it is possible that different dark galaxies formed by different means.[102] One candidate explanation for the low luminosity is that the galaxy lost its star-forming gas at an early stage, resulting in old stellar populations.[103][104]
Dwarfs
Despite the prominence of large elliptical and spiral galaxies, most galaxies are dwarf galaxies.[105] They are relatively small when compared with other galactic formations, being about one hundredth the size of the Milky Way, with only a few billion stars. Ultra-compact dwarf galaxies have recently been discovered that are only 100 parsecs across.[106]
Many dwarf galaxies may orbit a single larger galaxy; the Milky Way has at least a dozen such satellites, with an estimated 300–500 yet to be discovered.[107]Most of the information we have about dwarf galaxies come from observations of the local group, containing two spiral galaxies, the Milky Way and Andromeda, and many dwarf galaxies. These dwarf galaxies are classified as either irregular or dwarf elliptical/dwarf spheroidal galaxies.[105]
A study of 27 Milky Way neighbors found that in all dwarf galaxies, the central mass is approximately 10 million solar masses, regardless of whether it has thousands or millions of stars. This suggests that galaxies are largely formed by dark matter, and that the minimum size may indicate a form of warm dark matter incapable of gravitational coalescence on a smaller scale.[108]
Variants
Interacting

Interactions between galaxies are relatively frequent, and they can play an important role in galactic evolution. Near misses between galaxies result in warping distortions due to tidal interactions, and may cause some exchange of gas and dust.[109][110]Collisions occur when two galaxies pass directly through each other and have sufficient relative momentum not to merge. The stars of interacting galaxies usually do not collide, but the gas and dust within the two forms interacts, sometimes triggering star formation. A collision can severely distort the galaxies' shapes, forming bars, rings or tail-like structures.[109][110]
At the extreme of interactions are galactic mergers, where the galaxies' relative momentums are insufficient to allow them to pass through each other. Instead, they gradually merge to form a single, larger galaxy. Mergers can result in significant changes to the galaxies' original morphology. If one of the galaxies is much more massive than the other, the result is known as cannibalism, where the more massive larger galaxy remains relatively undisturbed, and the smaller one is torn apart. The Milky Way galaxy is currently in the process of cannibalizing the Sagittarius Dwarf Elliptical Galaxy and the Canis Major Dwarf Galaxy.[109][110]
Starburst

Stars are created within galaxies from a reserve of cold gas that forms giant molecular clouds. Some galaxies have been observed to form stars at an exceptional rate, which is known as a starburst. If they continue to do so, they would consume their reserve of gas in a time span less than the galaxy's lifespan. Hence starburst activity usually lasts only about ten million years, a relatively brief period in a galaxy's history. Starburst galaxies were more common during the universe's early history,[112] but still contribute an estimated 15% to total star production.[113]
Starburst galaxies are characterized by dusty concentrations of gas and the appearance of newly formed stars, including massive stars that ionize the surrounding clouds to create H II regions.[114] These stars produce supernova explosions, creating expanding remnants that interact powerfully with the surrounding gas. These outbursts trigger a chain reaction of star-building that spreads throughout the gaseous region. Only when the available gas is nearly consumed or dispersed does the activity end.[112]
Starbursts are often associated with merging or interacting galaxies. The prototype example of such a starburst-forming interaction is M82, which experienced a close encounter with the larger M81. Irregular galaxies often exhibit spaced knots of starburst activity.[115]
Radio galaxy

A radio galaxy is a galaxy with giant regions of radio emission extending well beyond its visible structure. These energetic radio lobes are powered by jets from its active galactic nucleus.[116] Radio galaxies are classified according to their Fanaroff–Riley classification. The FR I class have lower radio luminosity and exhibit structures which are more elongated; the FR II class are higher radio luminosity. The correlation of radio luminosity and structure suggests that the sources in these two types of galaxies may differ.[117]
Radio galaxies can also be classified as giant radio galaxies (GRGs), whose radio emissions can extend to scales of megaparsecs (3.26 million light-years). Alcyoneus is an FR II class low-excitation radio galaxy which has the largest observed radio emission, with lobed structures spanning 5 megaparsecs (16×106 ly). For comparison, another similarly sized giant radio galaxy is 3C 236, with lobes 15 million light-years across. It should however be noted that radio emissions are not always considered part of the main galaxy itself.[118]
A giant radio galaxy is a special class of objects characterized by the presence of radio lobes generated by relativistic jets powered by the central galaxy's supermassive black hole. Giant radio galaxies are different from ordinary radio galaxies in that they can extend to much larger scales, reaching upwards to several megaparsecs across, far larger than the diameters of their host galaxies.[119]
A "normal" radio galaxy do not have a source that is a supermassive black hole or monster neutron star; instead the source is synchrotron radiation from relativistic electrons accelerated by supernova. These sources are comparatively short lived, making the radio spectrum from normal radio galaxies an especially good way to study star formation. [120]
Active galaxy
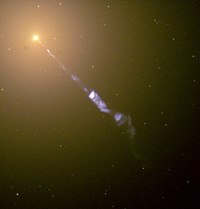
Some observable galaxies are classified as "active" if they contain an active galactic nucleus (AGN).[121] A significant portion of the galaxy's total energy output is emitted by the active nucleus instead of its stars, dust and interstellar medium. There are multiple classification and naming schemes for AGNs, but those in the lower ranges of luminosity are called Seyfert galaxies, while those with luminosities much greater than that of the host galaxy are known as quasi-stellar objects or quasars. Models of AGNs suggest that a significant fraction of their light is shifted to far-infrared frequencies because optical and UV emission in the nucleus is absorbed and remitted by dust and gas surrounding it.[122]
The standard model for an active galactic nucleus is based on an accretion disc that forms around a supermassive black hole (SMBH) at the galaxy's core region. The radiation from an active galactic nucleus results from the gravitational energy of matter as it falls toward the black hole from the disc.[123][124] The AGN's luminosity depends on the SMBH's mass and the rate at which matter falls onto it.In about 10% of these galaxies, a diametrically opposed pair of energetic jets ejects particles from the galaxy core at velocities close to the speed of light. The mechanism for producing these jets is not well understood.[125]
Seyfert galaxy
Seyfert galaxies are one of the two largest groups of active galaxies, along with quasars. They have quasar-like nuclei (very luminous, distant and bright sources of electromagnetic radiation) with very high surface brightnesses; but unlike quasars, their host galaxies are clearly detectable.[126] Seen through a telescope, a Seyfert galaxy appears like an ordinary galaxy with a bright star superimposed atop the core. Seyfert galaxies are divided into two principal subtypes based on the frequencies observed in their spectra.[127]
Quasar
Quasars are the most energetic and distant members of active galactic nuclei. Extremely luminous, they were first identified as high redshift sources of electromagnetic energy, including radio waves and visible light, that appeared more similar to stars than to extended sources similar to galaxies. Their luminosity can be 100 times that of the Milky Way.[128] The nearest known quasar, Markarian 231, is about 581 million light-years from Earth,[129] while others have been discovered as far away as UHZ1, roughly 13.2 billion light-years distant.[130][131] Quasars are noteworthy for providing the first demonstration of the phenomenon that gravity can act as a lens for light.[132]
Other AGNs
Blazars are believed to be active galaxies with a relativistic jet pointed in the direction of Earth. A radio galaxy emits radio frequencies from relativistic jets. A unified model of these types of active galaxies explains their differences based on the observer's position.[125]
Possibly related to active galactic nuclei (as well as starburst regions) are low-ionization nuclear emission-line regions (LINERs). The emission from LINER-type galaxies is dominated by weakly ionized elements. The excitation sources for the weakly ionized lines include post-AGB stars, AGN, and shocks.[133] Approximately one-third of nearby galaxies are classified as containing LINER nuclei.[124][133][134]
Luminous infrared galaxy
Luminous infrared galaxies (LIRGs) are galaxies with luminosities—the measurement of electromagnetic power output—above 1011 L☉ (solar luminosities). In most cases, most of their energy comes from large numbers of young stars which heat surrounding dust, which reradiates the energy in the infrared. Luminosity high enough to be a LIRG requires a star formation rate of at least 18 M☉ yr−1. Ultra-luminous infrared galaxies (ULIRGs) are at least ten times more luminous still and form stars at rates >180 M☉ yr−1. Many LIRGs also emit radiation from an AGN.[135][136] Infrared galaxies emit more energy in the infrared than all other wavelengths combined, with peak emission typically at wavelengths of 60 to 100 microns. LIRGs are believed to be created from the strong interaction and merger of spiral galaxies.[137] While uncommon in the local universe, LIRGs and ULIRGS were more prevalent when the universe was younger.[136]
Physical diameters
Galaxies do not have a definite boundary by their nature, and are characterized by a gradually decreasing stellar density as a function of increasing distance from their center, making measurements of their true extents difficult. Nevertheless, astronomers over the past few decades have made several criteria in defining the sizes of galaxies.
Angular diameter
As early as the time of Edwin Hubble in 1936, there have been attempts to characterize the diameters of galaxies. The earliest efforts were based on the observed angle subtended by the galaxy and its estimated distance, leading to an angular diameter (also called "metric diameter").[138] This type of measurement is subject to two significant issues, namely that the estimated distance to the galaxy must be corrected for the redshift-related space expansion and that collections of angular-diameter data are subject to selection bias as more distant observations preferentially select the most luminous objects.[139]
Isophotal diameter
The isophotal diameter is introduced as a conventional way of measuring a galaxy's size based on its apparent surface brightness.[140] Isophotes are curves in a diagram - such as a picture of a galaxy - that adjoins points of equal brightnesses, and are useful in defining the extent of the galaxy. The apparent brightness flux of a galaxy is measured in units of magnitudes per square arcsecond (mag/arcsec2; sometimes expressed as mag arcsec−2), which defines the brightness depth of the isophote. To illustrate how this unit works, a typical galaxy has a brightness flux of 18 mag/arcsec2 at its central region. This brightness is equivalent to the light of an 18th magnitude hypothetical point object (like a star) being spread out evenly in a one square arcsecond area of the sky.[141] The isophotal diameter is typically defined as the region enclosing all the light down to 25 mag/arcsec2 in the blue B-band,[142] which is then referred to as the D25 standard.[143]
galaxy | diameter | reference |
---|---|---|
Large Magellanic Cloud | 9.96 kiloparsecs (32,500 light-years) | [144] |
Milky Way | 26.8 kiloparsecs (87,400 light-years) | [9] |
Messier 87 | 40.55 kiloparsecs (132,000 light-years) | |
Andromeda Galaxy | 46.58 kiloparsecs (152,000 light-years) | [146] |
Effective radius (half-light) and its variations
The half-light radius (also known as effective radius; Re) is a measure that is based on the galaxy's overall brightness flux. This is the radius upon which half, or 50%, of the total brightness flux of the galaxy was emitted. This was first proposed by Gérard de Vaucouleurs in 1948.[147] The choice of using 50% was arbitrary, but proved to be useful in further works by R. A. Fish in 1963,[148] where he established a luminosity concentration law that relates the brightnesses of elliptical galaxies and their respective Re, and by José Luis Sérsic in 1968[149] that defined a mass-radius relation in galaxies.[140]
In defining Re, it is necessary that the overall brightness flux galaxy should be captured, with a method employed by Bershady in 2000 suggesting to measure twice the size where the brightness flux of an arbitrarily chosen radius, defined as the local flux, divided by the overall average flux equals to 0.2.[150] Using half-light radius allows a rough estimate of a galaxy's size, but is not particularly helpful in determining its morphology.[151]
Variations of this method exist. In particular, in the ESO-Uppsala Catalogue of Galaxies values of 50%, 70%, and 90% of the total blue light (the light detected through a B-band specific filter) had been used to calculate a galaxy's diameter.[152]
Petrosian magnitude
First described by Vahe Petrosian in 1976,[153] a modified version of this method has been used by the Sloan Digital Sky Survey (SDSS). This method employs a mathematical model on a galaxy whose radius is determined by the azimuthally (horizontal) averaged profile of its brightness flux. In particular, the SDSS employed the Petrosian magnitude in the R-band (658 nm, in the red part of the visible spectrum) to ensure that the brightness flux of a galaxy would be captured as much as possible while counteracting the effects of background noise. For a galaxy whose brightness profile is exponential, it is expected to capture all of its brightness flux, and 80% for galaxies that follow a profile that follows de Vaucouleurs's law.[154]
Petrosian magnitudes have the advantage of being redshift and distance independent, allowing the measurement of the galaxy's apparent size since the Petrosian radius is defined in terms of the galaxy's overall luminous flux.[155]
A critique of an earlier version of this method has been issued by the Infrared Processing and Analysis Center,[156] with the method causing a magnitude of error (upwards to 10%) of the values than using isophotal diameter. The use of Petrosian magnitudes also have the disadvantage of missing most of the light outside the Petrosian aperture, which is defined relative to the galaxy's overall brightness profile, especially for elliptical galaxies, with higher signal-to-noise ratios on higher distances and redshifts.[157] A correction for this method has been issued by Graham et al. in 2005, based on the assumption that galaxies follow Sérsic's law.[155]
Near-infrared method
This method has been used by 2MASS as an adaptation from the previously used methods of isophotal measurement. Since 2MASS operates in the near infrared, which has the advantage of being able to recognize dimmer, cooler, and older stars, it has a different form of approach compared to other methods that normally use B-filter. The detail of the method used by 2MASS has been described thoroughly in a document by Jarrett et al., with the survey measuring several parameters.[158]
The standard aperture ellipse (area of detection) is defined by the infrared isophote at the Ks band (roughly 2.2 μm wavelength) of 20 mag/arcsec2. Gathering the overall luminous flux of the galaxy has been employed by at least four methods: the first being a circular aperture extending 7 arcseconds from the center, an isophote at 20 mag/arcsec2, a "total" aperture defined by the radial light distribution that covers the supposed extent of the galaxy, and the Kron aperture (defined as 2.5 times the first-moment radius, an integration of the flux of the "total" aperture).[158]
Larger-scale structures
Deep-sky surveys show that galaxies are often found in groups and clusters. Solitary galaxies that have not significantly interacted with other galaxies of comparable mass in the past few billion years are relatively scarce.[159] Only about 5% of the galaxies surveyed are isolated in this sense.[160][161] However, they may have interacted and even merged with other galaxies in the past,[162] and may still be orbited by smaller satellite galaxies.[163]
On the largest scale, the universe is continually expanding, resulting in an average increase in the separation between individual galaxies (see Hubble's law). Associations of galaxies can overcome this expansion on a local scale through their mutual gravitational attraction. These associations formed early, as clumps of dark matter pulled their respective galaxies together. Nearby groups later merged to form larger-scale clusters. This ongoing merging process, as well as an influx of infalling gas, heats the intergalactic gas in a cluster to very high temperatures of 30–100 megakelvins.[164] About 70–80% of a cluster's mass is in the form of dark matter, with 10–30% consisting of this heated gas and the remaining few percent in the form of galaxies.[165]
Most galaxies are gravitationally bound to a number of other galaxies. These form a fractal-like hierarchical distribution of clustered structures, with the smallest such associations being termed groups. A group of galaxies is the most common type of galactic cluster; these formations contain the majority of galaxies (as well as most of the baryonic mass) in the universe.[166][167] To remain gravitationally bound to such a group, each member galaxy must have a sufficiently low velocity to prevent it from escaping (see Virial theorem). If there is insufficient kinetic energy, however, the group may evolve into a smaller number of galaxies through mergers.[168]
Clusters of galaxies consist of hundreds to thousands of galaxies bound together by gravity.[169] Clusters of galaxies are often dominated by a single giant elliptical galaxy, known as the brightest cluster galaxy, which, over time, tidally destroys its satellite galaxies and adds their mass to its own.[170]

Superclusters contain tens of thousands of galaxies, which are found in clusters, groups and sometimes individually. At the supercluster scale, galaxies are arranged into sheets and filaments surrounding vast empty voids.[172] Above this scale, the universe appears to be the same in all directions (isotropic and homogeneous),[173] though this notion has been challenged in recent years by numerous findings of large-scale structures that appear to be exceeding this scale. The Hercules–Corona Borealis Great Wall, currently the largest structure in the universe found so far, is 10 billion light-years (three gigaparsecs) in length.[174][175][176]
The Milky Way galaxy is a member of an association named the Local Group, a relatively small group of galaxies that has a diameter of approximately one megaparsec. The Milky Way and the Andromeda Galaxy are the two brightest galaxies within the group; many of the other member galaxies are dwarf companions of these two.[177] The Local Group itself is a part of a cloud-like structure within the Virgo Supercluster, a large, extended structure of groups and clusters of galaxies centered on the Virgo Cluster.[178] In turn, the Virgo Supercluster is a portion of the Laniakea Supercluster.[179]
Magnetic fields
Galaxies have magnetic fields of their own. A galaxy's magnetic field influences its dynamics in multiple ways, including affecting the formation of spiral arms and transporting angular momentum in gas clouds. The latter effect is particularly important, as it is a necessary factor for the gravitational collapse of those clouds, and thus for star formation.[180]
The typical average equipartition strength for spiral galaxies is about 10 μG (microgauss) or 1 nT (nanotesla). By comparison, the Earth's magnetic field has an average strength of about 0.3 G (Gauss) or 30 μT (microtesla). Radio-faint galaxies like M 31 and M33, the Milky Way's neighbors, have weaker fields (about 5 μG), while gas-rich galaxies with high star-formation rates, like M 51, M 83 and NGC 6946, have 15 μG on average. In prominent spiral arms, the field strength can be up to 25 μG, in regions where cold gas and dust are also concentrated. The strongest total equipartition fields (50–100 μG) were found in starburst galaxies—for example, in M 82 and the Antennae; and in nuclear starburst regions, such as the centers of NGC 1097 and other barred galaxies.[180]
Formation and evolution
Formation
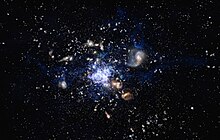
Current models of the formation of galaxies in the early universe are based on the ΛCDM model. About 300,000 years after the Big Bang, atoms of hydrogen and helium began to form, in an event called recombination. Nearly all the hydrogen was neutral (non-ionized) and readily absorbed light, and no stars had yet formed. As a result, this period has been called the "dark ages". It was from density fluctuations (or anisotropic irregularities) in this primordial matter that larger structures began to appear. As a result, masses of baryonic matter started to condense within cold dark matter halos.[182][183] These primordial structures allowed gasses to condense in to protogalaxies, large scale gas clouds that were precursors to the first galaxies.[184]: 6
As gas falls in to the gravity of the dark matter halos, its pressure and temperature rise. To condense further, the gas must radiate energy. This process was slow in the early universe dominated by hydrogen atoms and molecules which are inefficient radiators compared to heavier elements. As clumps of gas aggregate forming rotating disks, temperatures and pressures continue to increase. Some places within the disk reach high enough density to form stars.

Once protogalaxies began to form and contract, the first halo stars, called Population III stars, appeared within them.[185] These were composed of primordial gas, almost entirely of hydrogen and helium. Emission from the first stars heats the remaining gas helping to trigger additional star formation; the ultraviolet light emission from the first generation of stars re-ionized the surrounding neutral hydrogen in expanding spheres eventually reaching the entire universe, an event called reionization.[186] The most massive stars collapse in violent supernova explosions releasing heavy elements ("metals") into the interstellar medium.[187][184]: 14 This metal content is incorporated into population II stars.
Theoretical models for early galaxy formation have been verified and informed by a large number and variety of sophisticated astronomical observations.[184]: 43 The photometric observations generally need spectroscopic confirmation due the large number mechanisms that can introduce systematic errors. For example, a high redshift (z ~ 16) photometric observation by James Webb Space Telescope (JWST) was later corrected to be closer to z ~ 5.[188]Nevertheless, confirmed observations from the JWST and other observatories are accumulating, allowing systematic comparison of early galaxies to predictions of theory.[189]
Evidence for individual Population III stars in early galaxies is even more challenging. Even seemingly confirmed spectroscopic evidence may turn out to have other origins. For example, astronomers reported HeII emission evidence for Population III stars in the Cosmos Redshift 7 galaxy, with a redshift value of 6.60.[190] Subsequent observations[191] found metallic emission lines, OIII, inconsistent with an early-galaxy star.[185]: 108
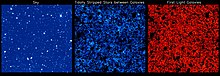
Evolution
Once stars begin to form, emit radiation, and in some cases explode, the process of galaxy formation becomes very complex, involving interactions between the forces of gravity, radiation, and thermal energy. Many details are still poorly understood.[193]
Within a billion years of a galaxy's formation, key structures begin to appear.[194] Globular clusters, the central supermassive black hole, and a galactic bulge of metal-poor Population II stars form. The creation of a supermassive black hole appears to play a key role in actively regulating the growth of galaxies by limiting the total amount of additional matter added.[195] During this early epoch, galaxies undergo a major burst of star formation.[196]
During the following two billion years, the accumulated matter settles into a galactic disc.[197] A galaxy will continue to absorb infalling material from high-velocity clouds and dwarf galaxies throughout its life.[198] This matter is mostly hydrogen and helium. The cycle of stellar birth and death slowly increases the abundance of heavy elements, eventually allowing the formation of planets.[199]
Star formation rates in galaxies depend upon their local environment. Isolated 'void' galaxies have highest rate per stellar mass, with 'field' galaxies associated with spiral galaxies having lower rates and galaxies in dense cluster having the lowest rates.[201]
На эволюцию галактик могут существенно влиять взаимодействия и столкновения. Слияния галактик были обычным явлением в раннюю эпоху, и большинство галактик имели своеобразную морфологию. [202] Учитывая расстояния между звездами, подавляющее большинство звездных систем в сталкивающихся галактиках не пострадают. Однако гравитационное удаление межзвездного газа и пыли, составляющих спиральные рукава, приводит к образованию длинной цепочки звезд, известной как приливные хвосты. Примеры этих образований можно увидеть в NGC 4676. [203] или Антенны Галактик . [204]
Галактика Млечный Путь и близлежащая Галактика Андромеды движутся навстречу друг другу со скоростью около 130 км/с , и — в зависимости от бокового движения — они могут столкнуться примерно через пять-шесть миллиардов лет. Хотя Млечный Путь никогда раньше не сталкивался с такой большой галактикой, как Андромеда, в прошлом он сталкивался и сливался с другими галактиками. [205] Космологическое моделирование показывает, что 11 миллиардов лет назад она слилась с особенно большой галактикой, получившей название Кракен . [206] [207]
Столь масштабные взаимодействия редки. С течением времени слияния двух систем одинакового размера становятся менее частыми. Большинство ярких галактик практически не изменились за последние несколько миллиардов лет, а чистая скорость звездообразования, вероятно, также достигла своего пика около десяти миллиардов лет назад. [208]
Будущие тенденции
Спиральные галактики, такие как Млечный Путь , производят новые поколения звезд, пока в их спиральных рукавах есть плотные молекулярные облака межзвездного водорода. [209] Эллиптические галактики в основном лишены этого газа и поэтому образуют мало новых звезд. [210] Запасы звездообразующего материала конечны; как только звезды превратят имеющийся запас водорода в более тяжелые элементы, новое звездообразование прекратится. [211] [212]
Ожидается, что нынешняя эра звездообразования продлится до ста миллиардов лет, а затем «звездный век» закончится примерно через десять-сто триллионов лет (10 13 –10 14 лет), когда самые маленькие и долгоживущие звезды видимой Вселенной, крошечные красные карлики , начинают тускнеть. В конце звездной эпохи галактики будут состоять из компактных объектов : коричневых карликов , белых карликов остывающих или холодных (« черные карлики »), нейтронных звезд и черных дыр . В конце концов, в результате гравитационной релаксации все звезды либо упадут в центральные сверхмассивные черные дыры, либо будут выброшены в межгалактическое пространство в результате столкновений. [211] [213]
Галерея
- Галактики (слева/вверху, справа/внизу): NGC 7541 , 3021 , NGC NGC 5643 , , NGC NGC 3254 3147 , NGC 105 , NGC 2608 , NGC 3583 , NGC 3147 , MRK 1337 , NGC 5861 , NGC 2525 , 1015 , UGC 9391 , NGC 691 , NGC 7678 , NGC 2442 , NGC 5468 , NGC 5917 , NGC 4639 , NGC 3972 , Усиковые галактики , NGC 5584 , M106 , NGC 7250 , NGC 3370 , NGC 5728 , НГК 4424 , НГК 1559 , НГК 3982 , NGC 1448 , NGC 4680 , M101 , NGC 1365 , NGC 7329 , NGC 3447
- 19 спиральных галактик, обращенных лицом друг к другу, полученные космическим телескопом Джеймса Уэбба в ближнем и среднем инфракрасном диапазоне. Старые звезды здесь кажутся голубыми и скоплены в ядрах галактик. Светящаяся пыль, показывающая, где она находится вокруг звезд и между звездами, окрашенная в оттенки красного и оранжевого. Звезды, которые еще не полностью сформировались и окружены газом и пылью, кажутся ярко-красными. [214]
См. также
- Яркие ранние галактики
- Темная галактика
- Галактическая ориентация
- Формирование и эволюция галактик
- Прославленный проект
- Список галактик
- Список самых далеких астрономических объектов
- Список ближайших галактик
- Список крупнейших галактик
- Галактика с низкой поверхностной яркостью
- Очертание галактик
- Хронология знаний о галактиках, скоплениях галактик и крупномасштабной структуре
Примечания
- ^ Это диаметр, измеренный по D 25 стандарту . Исследование 2018 года показало, что за пределами этого диаметра существуют звезды диска, хотя неясно, насколько это влияет на профиль поверхностной яркости. [10] [11]
- ↑ Райт назвал Млечный Путь Великим Вихрем (Великим Водоворотом) и оценил его диаметр в 8,64 × 10. 12 миль (13,9×10 12 км). [32]
Ссылки
- ^ Спарк и Галлахер 2000 , стр. i.
- ^ Хапп, Эрика; Рой, Стив; Вацке, Меган (12 августа 2006 г.). «НАСА находит прямое доказательство существования темной материи» . НАСА . Архивировано из оригинала 28 марта 2020 года . Проверено 17 апреля 2007 г.
- ^ Хауэлл, Элизабет; Харви, Эйлса (11 февраля 2022 г.). «Сколько звезд во Вселенной? - Можем ли мы оценить общее количество звезд?» . Space.com . Проверено 16 июля 2022 г.
- ^ Стригари, Луи Э.; Буллок, Джеймс С.; Каплингхат, Манодж; Саймон, Джошуа Д.; Геха, Марла ; Уиллман, Бет; Уокер, Мэтью Г. (2008). «Общая шкала масс галактик-спутников Млечного Пути» . Природа . 454 (7208): 1096–1097. arXiv : 0808.3772 . Бибкод : 2008Natur.454.1096S . дои : 10.1038/nature07222 . ISSN 0028-0836 . ПМИД 18756252 .
- ^ Гувер, Аарон (16 июня 2003 г.). «Астрономы УФ: Вселенная немного проще, чем ожидалось» (пресс-релиз). Университет Флориды . Архивировано из оригинала 20 июля 2011 года . Проверено 4 марта 2011 г.
- На основе: Грэм, Алистер В.; Гусман, Рафаэль (2003). «HST-фотометрия карликовых эллиптических галактик в коме и объяснение предполагаемой структурной дихотомии между карликовыми и яркими эллиптическими галактиками». Астрономический журнал . 125 (6): 2936–2950. arXiv : astro-ph/0303391 . Бибкод : 2003AJ....125.2936G . дои : 10.1086/374992 . S2CID 13284968 .
- ↑ Перейти обратно: Перейти обратно: а б Джарретт, TH «Атлас морфологии галактик в ближнем инфракрасном диапазоне» . Центр инфракрасной обработки и анализа . Калифорнийский технологический институт . Архивировано из оригинала 20 ноября 2023 года . Проверено 9 января 2007 г.
- ^ Чемла, Сара (14 января 2021 г.). «Астрономы ошибались относительно количества галактик во Вселенной» . «Джерузалем Пост» . Архивировано из оригинала 14 января 2021 года . Проверено 14 января 2021 г.
- ^ Сондерс, Тоби (25 июля 2023 г.). «Сколько галактик во Вселенной? Гораздо больше, чем вы думаете» . Научный фокус BBC . Проверено 9 января 2024 г.
- ↑ Перейти обратно: Перейти обратно: а б Гудвин, Саймон П.; Гриббин, Джон ; Хендри, Мартин А. (1998). «Относительный размер Млечного Пути» (PDF) . Обсерватория . 118 : 201–208. Бибкод : 1998Obs...118..201G .
- ^ Лопес-Корредойра, М.; Альенде Прието, К.; Гарсон, Ф.; Ван, Х.; Лю, К.; Дэн, Л. (9 апреля 2018 г.). «Дисковые звезды Млечного Пути обнаружены на расстоянии более 25 кпк от его центра» . Астрономия и астрофизика . 612 : Л8. arXiv : 1804.03064 . Бибкод : 2018A&A...612L...8L . дои : 10.1051/0004-6361/201832880 . S2CID 59933365 .
- ^ Фриман, Дэвид (25 мая 2018 г.). «Галактика Млечный Путь может быть намного больше, чем мы думали» (Пресс-релиз). CNBC . Архивировано из оригинала 13 августа 2018 года . Проверено 13 августа 2018 г.
- ^ Рисс, Адам Г .; Флири, Юрген; Вальс-Габо, Дэвид (2012). «Зависимость периода цефеид от светимости в ближнем инфракрасном диапазоне и расстояние до M31 по данным широкоугольной камеры 3 космического телескопа Хаббл». Астрофизический журнал . 745 (2): 156. arXiv : 1110.3769 . Бибкод : 2012ApJ...745..156R . дои : 10.1088/0004-637X/745/2/156 . S2CID 119113794 .
- ^ «Скопления галактик и крупномасштабная структура» . Кембриджский университет . Архивировано из оригинала 26 апреля 2012 года . Проверено 15 января 2007 г.
- ^ Гибни, Элизабет (2014). «Новый адрес Земли: «Солнечная система, Млечный Путь, Ланиакеа» . Природа . дои : 10.1038/nature.2014.15819 . S2CID 124323774 .
- ^ Лук, Чарльз Т .; Фридрихсен, GWS; Берчфилд, Роберт В. , ред. (1966). Оксфордский словарь английской этимологии . Оксфорд: Кларендон Пресс . п. 385.
- ↑ Перейти обратно: Перейти обратно: а б Харпер, Дуглас. «галактика» . Интернет-словарь этимологии . Архивировано из оригинала 17 ноября 2011 года . Проверено 11 ноября 2011 г.
- ^ Уоллер и Ходж 2003 , с. 91
- ^ Конечный, Любомир. «Эмблематика, сельское хозяйство и мифография в происхождении Млечного Пути» (PDF) . Академия наук Чехии . Архивировано из оригинала (PDF) 20 июля 2006 г. Проверено 5 января 2007 г.
- ^ Рао, Джо (2 сентября 2005 г.). «Исследуй царство лучников» . Space.com . Архивировано из оригинала 31 октября 2010 года . Проверено 3 января 2007 г.
- ^ «Результаты по объекту МЕСЬЕ 109 (М109)» . Внегалактическая база данных НАСА/IPAC . Калифорнийский технологический институт . Проверено 13 января 2023 г.
- ^ «Что такое Слоановский цифровой обзор неба?» . Слоановский цифровой обзор неба . Архивировано из оригинала 12 января 2024 года . Проверено 3 марта 2024 г.
- ^ Плутарх (2006). Полное собрание сочинений, том 3: Очерки и сборники . Библиотека Эхо. п. 66. ИСБН 978-1-4068-3224-2 . Архивировано из оригинала 24 марта 2021 года . Проверено 25 июля 2018 г.
- ↑ Перейти обратно: Перейти обратно: а б с Монтада, Хосеп Пуч (28 сентября 2007 г.). «Ибн Баджа» . Стэнфордская энциклопедия философии . Архивировано из оригинала 16 марта 2020 года . Проверено 11 июля 2008 г.
- ^ Гейдарзаде 2008 , стр. 23–25
- ^ Мохамед 2000 , стр. 49–50.
- ^ Буали, Хамид-Эддин; Згал, Мурад; Лахдар, Зохра Б. (2005). «Популяризация оптических явлений: создание первой мастерской Ибн Аль-Хайсама по фотографии» (PDF) . Во Флори, Франсуа (ред.). Девятое международное тематическое совещание по образованию и подготовке кадров в области оптики и фотоники . Труды SPIE . Том. 9664, Девятое международное тематическое совещание по образованию и обучению в области оптики и фотоники. Бибкод : 2005SPIE.9664E..22B . дои : 10.1117/12.2207764 .
- ^ О'Коннор, Джон Дж.; Робертсон, Эдмунд Ф. , «Абу Аррайхан Мухаммад ибн Ахмад аль-Бируни» , Архив истории математики MacTutor , Университет Сент-Эндрюс
- ^ Гейдарзаде 2008 , с. 25, таблица 2.1
- ^ Ливингстон, Джон В. (1971). «Ибн Кайим аль-Джавзия: защита четырнадцатого века от астрологических предсказаний и алхимической трансмутации». Журнал Американского восточного общества . 91 (1): 96–103. дои : 10.2307/600445 . JSTOR 600445 .
- ^ Галилей, Галилей (1610). Сидерей Нунций . Венеция : Томас Бальони. стр. 15–16 . Проверено 10 января 2024 г.
- Английский перевод: Галилей, Галилей ; Кеплер, Иоганнес (1880). «Сидерический вестник Галилео Галилея»: и часть предисловия к «Диоптрике» Кеплера, содержащая оригинальный отчет об астрономических открытиях Галилея . Перевод Карлоса, Эдварда Стаффорда. Лондон: Ривингтонс. стр. 42–43 . Проверено 10 января 2024 г.
- ^ О'Коннор, Джон Дж.; Робертсон, Эдмунд Ф. (ноябрь 2002 г.). «Галилео Галилей» . MacTutor Архив истории математики . Университет Сент-Эндрюс . Архивировано из оригинала 3 марта 2016 года . Проверено 8 января 2007 г.
- ^ Райт 1750 , с. 73.
- ^ Райт 1750 , стр. 48–.
- ↑ Перейти обратно: Перейти обратно: а б с д Эванс, Джей Си (24 ноября 1998 г.). «Наша Галактика» . Университет Джорджа Мейсона . Архивировано из оригинала 24 сентября 2003 года . Проверено 4 января 2007 г.
- ↑ Перейти обратно: Перейти обратно: а б Кант, Иммануил (20 ноября 2016 г.) [1755]. история и небес Универсальная естественная теория Кенигсберг и Лейпциг : Иоганн Фридрих Петерсен. ISBN 978-3-89131-002-1 . Архивировано из оригинала 20 ноября 2016 г. Английский перевод Яна Джонстона доступен на Wayback Machine (архивировано 29 августа 2014 г.).
- ^ Гершель, Уильям (1785). «XII. О построении небес» . Некоторые отчеты о нынешних предприятиях, исследованиях и трудах гениальных людей во многих значительных частях мира . Философские труды Лондонского королевского общества. Том. 75. Лондон. стр. 213–266. дои : 10.1098/rstl.1785.0012 . ISSN 0261-0523 . S2CID 186213203 . Архивировано из оригинала 20 ноября 2016 года . Проверено 27 января 2016 г. Схема галактики Гершеля появляется сразу после с. 266.
- ^ Пол 1993 , стр. 16–18.
- ^ Тримбл, В. (1999). «Роберт Трамплер и (не)прозрачность космоса». Бюллетень Американского астрономического общества . 31 (31): 1479. Бибкод : 1999AAS...195.7409T .
- ↑ Перейти обратно: Перейти обратно: а б Кеппл и Саннер 1998 , стр. 18.
- ↑ Перейти обратно: Перейти обратно: а б Фроммерт, Хартмут; Кронберг, Кристина (11 марта 2004 г.). «Большое Магелланово Облако, БМО» . Парижская обсерватория . Архивировано из оригинала 22 июня 2017 года.
- ^ Фроммерт, Хартмут; Кронберг, Кристина. «Абд-ар-Рахман Аль Суфи (7 декабря 903 г. - 25 мая 986 г. н.э.)» . Парижская обсерватория . Архивировано из оригинала 16 апреля 2007 года . Проверено 19 апреля 2007 г.
- ^ Гордон, Куртисс Дж. (1969). «История нашего понимания спиральной галактики: Мессье 33» (PDF) . Ежеквартальный журнал Королевского астрономического общества . 10 : 293–307. Архивировано (PDF) из оригинала 26 апреля 2021 г.
- ↑ См. текст, цитируемый из книги Райта «Оригинальная теория или новая гипотеза Вселенной» в Дайсон, Фриман Дж. (1979). Возмущение Вселенной . Панские книги . п. 245. ИСБН 978-0-330-26324-5 . Архивировано из оригинала 24 марта 2021 года . Проверено 25 июля 2018 г.
- ^ Пейн-Гапошкин, Сесилия Х. (1953). «Почему галактики имеют спиральную форму?» . Научный американец . Том. 189, нет. 3. С. 89–99. ISSN 0036-8733 . JSTOR 24944338 .
- ^ Стейнике, Вольфганг (2012). «Тайна M51: Лорд Росс, Робинсон, Саут и открытие спиральной структуры в 1845 году» . Журнал астрономической истории и наследия . 15 (1): 19–29. Бибкод : 2012JAHH...15...19S . дои : 10.3724/SP.J.1440-2807.2012.01.03 .
- ^ Слайфер, Весто. М. (1913). «Лучевая скорость туманности Андромеды». Бюллетень обсерватории Лоуэлл . 1 (8): 56–57. Бибкод : 1913LowOB...2...56S .
- ^ Слайфер, Весто М. (1915). «Спектрографические наблюдения туманностей». Популярная астрономия . Том. 23. С. 21–24. Бибкод : 1915PA.....23...21S .
- ^ Кертис, Хибер Д. (1988). «Новые звезды в спиральных туманностях и теория островной Вселенной» . Публикации Тихоокеанского астрономического общества . 100 : 6. Бибкод : 1988PASP..100....6C . дои : 10.1086/132128 .
- ^ Уивер, Гарольд. Ф. «Роберт Джулиус Трамплер» . Национальная академия наук США . Архивировано из оригинала 24 декабря 2013 года . Проверено 5 января 2007 г.
- ^ Эпик, Эрнст (1922). «Оценка расстояния до туманности Андромеды». Астрофизический журнал . 55 : 406. Бибкод : 1922ApJ....55..406O . дои : 10.1086/142680 .
- ^ Хаббл, Эдвин П. (1929). «Спиральная туманность как звездная система Мессье 31» . Астрофизический журнал . 69 : 103–158. Бибкод : 1929ApJ....69..103H . дои : 10.1086/143167 .
- ^ Хаббл, Эдвин П. (1926). «№ 324. Внегалактические туманности». Материалы обсерватории Маунт-Вилсон . 324 . Институт Карнеги в Вашингтоне: 1–49. Бибкод : 1926CMWCI.324....1H .
- ^ Сэндидж, Аллан Р. (1989). «Эдвин Хаббл, 1889–1953» . Журнал Королевского астрономического общества Канады . 83 (6): 351–362. Бибкод : 1989JRASC..83..351S . Архивировано из оригинала 5 февраля 2024 года . Проверено 21 апреля 2024 г.
- ^ Рике, Джордж Генри (2012). Измерение Вселенной: многоволновая перспектива . Издательство Кембриджского университета . п. xi. ISBN 978-0-521-76229-8 .
- ^ «Ближний, средний и дальний инфракрасный диапазон» . Центр инфракрасной обработки и анализа . Калифорнийский технологический институт . Архивировано из оригинала 30 декабря 2006 года . Проверено 2 января 2007 г.
- ^ Фракной и др. 2023 , стр. 144 , 202 .
- ^ «Влияние верхних слоев атмосферы Земли на радиосигналы» . НАСА . Архивировано из оригинала 7 мая 2012 года . Проверено 10 августа 2006 г.
- ^ «Телескоп НАСА увидел черную дыру Мунка на звезде» . Исследователь эволюции галактики . НАСА . 5 декабря 2006. Архивировано из оригинала 25 апреля 2010 года . Проверено 2 января 2007 г.
- ^ Данн, Роберт (9 августа 2005 г.). «Введение в рентгеновскую астрономию» . Института астрономии Рентгеновская группа . Архивировано из оригинала 1 января 2007 года . Проверено 2 января 2007 г.
- ^ Корбелли, Э.; Салуччи, П. (2000). «Расширенная кривая вращения и гало темной материи М33» . Ежемесячные уведомления Королевского астрономического общества . 311 (2): 441–447. arXiv : astro-ph/9909252 . Бибкод : 2000MNRAS.311..441C . дои : 10.1046/j.1365-8711.2000.03075.x .
- ^ Тенн, Джо. «Хендрик Кристоффель ван де Хюльст» . Медалисты Брюса . Государственный университет Сономы . Архивировано из оригинала 14 января 2012 года . Проверено 5 января 2007 г.
- ^ Лопес-Корредойра, М.; и др. (2001). «В поисках галактического бара и кольца в ДЕНИСе». Астрономия и астрофизика . 373 (1): 139–152. arXiv : astro-ph/0104307 . Бибкод : 2001A&A...373..139L . дои : 10.1051/0004-6361:20010560 . S2CID 18399375 .
- ^ Рубин, Вера К. (1983). «Темная материя в спиральных галактиках». Научный американец . Том. 248, нет. 6. С. 96–106. Бибкод : 1983SciAm.248f..96R . doi : 10.1038/scientificamerican0683-96 .
- ^ Рубин, Вера К. (2000). «Сто лет вращающихся галактик» . Публикации Тихоокеанского астрономического общества . 112 (772): 747–750. Бибкод : 2000PASP..112..747R . дои : 10.1086/316573 . S2CID 122927800 .
- ^ «Хаббл исключает ведущее объяснение темной материи» . Служба новостей Хаббла (пресс-релиз). НАСА . 17 октября 1994 года. Архивировано из оригинала 27 ноября 2012 года . Проверено 8 января 2007 г.
- ^ Мэттсон, Барбара (27 ноября 2002 г.). Гибб, Мередит (ред.). «Сколько здесь галактик?» . Представьте себе Вселенную! . НАСА . Архивировано из оригинала 28 июля 2012 года . Проверено 8 января 2007 г.
- ^ Краан-Кортевег, RC; Юрашек, С. (2000). «Картирование скрытой Вселенной: распределение галактик в зоне избегания». Публикации Астрономического общества Австралии . 17 (1): 6–12. arXiv : astro-ph/9910572 . Бибкод : 2000PASA...17....6K . дои : 10.1071/AS00006 . S2CID 17900483 .
- ^ Конселиче, Кристофер Дж.; и др. (2016). «Эволюция плотности числа галактик при z < 8 и ее последствия» . Астрофизический журнал . 830 (2): 83. arXiv : 1607.03909 . Бибкод : 2016ApJ...830...83C . дои : 10.3847/0004-637X/830/2/83 . S2CID 17424588 .
- ^ Фонтан Генри (17 октября 2016 г.). «Как минимум два триллиона галактик» . Нью-Йорк Таймс . Архивировано из оригинала 31 декабря 2019 года . Проверено 17 октября 2016 г.
- ^ Лауэр, Тод Р.; и др. (11 января 2021 г.). «Новые горизонты наблюдений космического оптического фона» . Астрофизический журнал . 906 (2): 77. arXiv : 2011.03052 . Бибкод : 2021ApJ...906...77L . дои : 10.3847/1538-4357/abc881 . hdl : 1721.1/133770 . ISSN 1538-4357 . S2CID 226277978 .
- ^ «Космический корабль «Новые горизонты» отвечает на вопрос: насколько темен космос?» . Физика.орг . Архивировано из оригинала 15 января 2021 года . Проверено 15 января 2021 г.
- ^ Фракной и др. 2023 , стр. 851–856 , 907–915 .
- ^ Барстоу, Массачусетс (2005). «Эллиптические галактики» . Физический факультет Лестерского университета . Архивировано из оригинала 29 июля 2012 года . Проверено 8 июня 2006 г.
- ^ Мэтьюз, Томас А.; Морган, Уильям В.; Шмидт, Мартен (1964). «Обсуждение галактик, идентифицированных [так в оригинале] с помощью радиоисточников» . Астрофизический журнал . 140 : 35. Бибкод : 1964ApJ...140...35M . дои : 10.1086/147890 .
- ^ «Отслеживание роста галактик» . НАСА . 6 февраля 2017 г. Проверено 20 апреля 2024 г.
- ^ Тонри, Джон Л. (1987). «Свойства CD-галактик» . Структура и динамика эллиптических галактик . Том. 127. с. 89. Бибкод : 1987IAUS..127...89T . дои : 10.1007/978-94-009-3971-4_7 . ISBN 978-90-277-2586-8 . S2CID 117980521 .
- ^ Товмассян Грант М.; Андернах, Хайнц (11 декабря 2012 г.). «Об образовании CD-галактик и их родительских скоплений: Формирование CD-галактик и родительских скоплений» . Ежемесячные уведомления Королевского астрономического общества . 427 (3): 2047–2056. arXiv : 1212.0238 . дои : 10.1111/j.1365-2966.2012.22044.x .
- ^ Бендер, Ральф; Корменди, Джон; Корнелл, Марк Э.; Фишер, Дэвид Б. (30 июня 2015 г.). «Структура и формирование CD-галактик: NGC 6166 в Abell 2199» . Астрофизический журнал . 807 (1): 56. arXiv : 1411.2598 . Бибкод : 2015ApJ...807...56B . дои : 10.1088/0004-637X/807/1/56 . ISSN 1538-4357 .
Эта идея возникла в период расцвета проблемы охлаждающего потока, когда мы наблюдали большие количества испускающего рентгеновские лучи горячего газа в кластерах, но не могли измерить температурные профили. [...] Эта возможность теперь рассматривается как неудачная.
- ^ Фабиан, AC; Нульсен, PEJ (1977). «Дозвуковая аккреция остывающего газа в скоплениях галактик» . Ежемесячные уведомления Королевского астрономического общества . 180 (3): 479. Бибкод : 1977MNRAS.180..479F . дои : 10.1093/mnras/180.3.479 .
- ^ Макдональд, М.; Макнамара, БР; Войт, генеральный директор; Бэйлисс, М.; Бенсон, бакалавр; Бродуин, М.; Каннинг, REA; Флориан, МК; Гармир, врач общей практики; Гаспари, М.; Глэддерс, доктор медицины; Главачек-Ларрондо, Дж.; Кара, Э.; Райхардт, CL; Рассел, HR (1 ноября 2019 г.). «Анатомия охлаждающего потока: реакция обратной связи на чистое охлаждение в ядре скопления Феникс» . Астрофизический журнал . 885 (1): 63. arXiv : 1904.08942 . Бибкод : 2019ApJ...885...63M . дои : 10.3847/1538-4357/ab464c . ISSN 0004-637X .
- ^ «Галактический лук» . Европейское космическое агентство . Архивировано из оригинала 6 августа 2020 года . Проверено 11 мая 2015 г.
- ^ Уильямс, MJ; Бюро, М.; Каппеллари, М. (2010). «Кинематические ограничения на содержание звезд и темной материи в спиральных галактиках и галактиках S0». Ежемесячные уведомления Королевского астрономического общества . 400 (4): 1665–1689. arXiv : 0909.0680 . Бибкод : 2009MNRAS.400.1665W . дои : 10.1111/j.1365-2966.2009.15582.x . S2CID 17940107 .
- ^ Смит, Джин (6 марта 2000 г.). «Галактики — Спиральные туманности» . Калифорнийский университет, Центр астрофизики и космических наук Сан-Диего. Архивировано из оригинала 27 марта 2023 года . Проверено 30 ноября 2006 г.
- ^ Ван ден Берг 1998 , с. 17
- ^ Обрешков, Д.; Глейзбрук, К. (28 февраля 2014 г.). «Фундаментальная связь массы, спина и морфологии спиральных галактик» . Астрофизический журнал . 784 (1): 26. arXiv : 1312.4543 . Бибкод : 2014ApJ...784...26O . дои : 10.1088/0004-637X/784/1/26 . ISSN 0004-637X .
- ^ «Толстый или плоский: придание галактикам формы» . Физика.орг . 27 февраля 2014 г. Архивировано из оригинала 24 марта 2021 г. 4
- ^ Бертин и Лин 1996 , стр. 65–85.
- ^ Белкора 2003 , с. 355
- ^ Эскридж, ПБ; Фрогель, Дж. А. (1999). «Какова истинная доля спиральных галактик с перемычкой?». Астрофизика и космическая наука . 269/270: 427–430. Бибкод : 1999Ap&SS.269..427E . дои : 10.1023/А:1017025820201 . S2CID 189840251 .
- ^ Бурно, Ф.; Комбс, Ф. (2002). «Аккреция газа на спиральных галактиках: образование и обновление баров». Астрономия и астрофизика . 392 (1): 83–102. arXiv : astro-ph/0206273 . Бибкод : 2002A&A...392...83B . дои : 10.1051/0004-6361:20020920 . S2CID 17562844 .
- ^ Кнапен, Йохан Х.; Перес-Рамирес, Даниэль; Лайне, Сеппо (2002). «Околоядерные области в спиральных галактиках с перемычкой — II. Отношения с родительскими галактиками». Ежемесячные уведомления Королевского астрономического общества . 337 (3): 808–828. arXiv : astro-ph/0207258 . Бибкод : 2002MNRAS.337..808K . дои : 10.1046/j.1365-8711.2002.05840.x . S2CID 10845683 .
- ^ Алар, Кристоф (2001). «Еще один бар в Арденнах». Письма по астрономии и астрофизике . 379 (2): L44–L47. arXiv : astro-ph/0110491 . Бибкод : 2001A&A...379L..44A . дои : 10.1051/0004-6361:20011487 . S2CID 18018228 .
- ^ Сандерс, Роберт (9 января 2006 г.). «Галактика Млечный Путь искривлена и вибрирует, как барабан» (Пресс-релиз). Калифорнийский университет в Беркли . Архивировано из оригинала 18 января 2014 года . Проверено 24 мая 2006 г.
- ^ Белл, Греция; Левин, SE (1997). «Масса Млечного Пути и принадлежность к карликовому сфероидальному потоку». Бюллетень Американского астрономического общества . 29 (2): 1384. Бибкод : 1997AAS...19110806B .
- ^ Сантос, Мигель (21 марта 2016 г.). «Мы только что открыли новый тип колоссальной галактики» . Футуризм . Архивировано из оригинала 24 марта 2021 года . Проверено 21 марта 2016 г.
- ^ Огл, Патрик М.; Ланц, Лоранн; Надер, Кирилл; Хелу, Джордж (1 января 2016 г.). «Сверхсветящиеся спиральные галактики» . Астрофизический журнал . 817 (2): 109. arXiv : 1511.00659 . Бибкод : 2016ApJ...817..109O . дои : 10.3847/0004-637X/817/2/109 . ISSN 0004-637X . S2CID 35287348 .
- ^ Гербер, РА; Лэмб, ЮАР; Бальсара, Д.С. (1994). «Эволюция кольцевой галактики как функция массы «нарушителя». Бюллетень Американского астрономического общества . 26 : 911. Бибкод : 1994AAS...184.3204G .
- ^ «ISO раскрывает скрытые кольца Андромеды» (Пресс-релиз). Европейское космическое агентство . 14 октября 1998 года. Архивировано из оригинала 5 мая 2006 года . Проверено 24 мая 2006 г.
- ^ «Спитцер раскрывает то, что упустил Эдвин Хаббл» (пресс-релиз). Гарвард-Смитсоновский центр астрофизики . 31 мая 2004 года. Архивировано из оригинала 7 сентября 2006 года . Проверено 6 декабря 2006 г.
- ^ Барстоу, Массачусетс (2005). «Неправильные галактики» . Университет Лестера . Архивировано из оригинала 27 февраля 2012 года . Проверено 5 декабря 2006 г.
- ^ Фракной и др. 2023 , стр. 879 .
- ^ Пандья, Вирадж; Романовский, Аарон Дж.; Лайне, Сеппо; Броди, Джин П.; Джонсон, Бенджамин Д.; Глаккум, Уильям; Вийом, Алекса; Куйландр, Жан-Шарль; Гвин, Стивен; Крик, Джессика; Ласкер, Рональд; Мартин-Наварро, Игнасио; Мартинес-Дельгадо, Дэвид; ван Доккум, Питер (1 мая 2018 г.). «Звездное население двух ультрадиффузных галактик по данным оптической и ближней инфракрасной фотометрии» . Астрофизический журнал . 858 (1): 29. arXiv : 1711.05272 . Бибкод : 2018ApJ...858...29P . дои : 10.3847/1538-4357/aab498 . ISSN 0004-637X .
- ^ Прощай, Деннис (26 января 2024 г.). «Как вы называете галактику без звезд?» . Нью-Йорк Таймс . Архивировано из оригинала 2 февраля 2024 года.
- ^ ван Доккум, Питер Г.; Авраам, Роберто ; Мерритт, Эллисон; Чжан, Цзелай; Геха, Марла ; Конрой, Чарли (январь 2015 г.). «Сорок семь чрезвычайно рассеянных галактик размером с Млечный Путь в скоплении Комы». Письма астрофизического журнала . 798 (2): L45. arXiv : 1410.8141 . Бибкод : 2015ApJ...798L..45V . дои : 10.1088/2041-8205/798/2/L45 . ISSN 2041-8205 .
- ↑ Перейти обратно: Перейти обратно: а б Матео, Марио (1998). «Карликовые галактики Местной группы» . Ежегодный обзор астрономии и астрофизики . 36 (1): 435–506. arXiv : astro-ph/9810070 . Бибкод : 1998ARA&A..36..435M . дои : 10.1146/annurev.astro.36.1.435 . ISSN 0066-4146 . S2CID 119333888 .
- ^ Филлипс, С.; Дринкуотер, MJ; Грегг, доктор медицины; Джонс, Дж. Б. (2001). «Сверхкомпактные карликовые галактики в скоплении Печи». Астрофизический журнал . 560 (1): 201–206. arXiv : astro-ph/0106377 . Бибкод : 2001ApJ...560..201P . дои : 10.1086/322517 . S2CID 18297376 .
- ^ Грошонг, Кимм (24 апреля 2006 г.). «Вокруг Млечного Пути обнаружены странные галактики-спутники» . Новый учёный . Архивировано из оригинала 2 июля 2015 года . Проверено 10 января 2007 г.
- ^ Ширбер, М. (27 августа 2008 г.). «Нет похудению карликовых галактик» . Наука СЕЙЧАС . Архивировано из оригинала 30 мая 2020 года . Проверено 27 августа 2008 г.
- ↑ Перейти обратно: Перейти обратно: а б с «Взаимодействие галактик» . Университета Мэриленда Кафедра астрономии . Архивировано из оригинала 9 мая 2006 года . Проверено 19 декабря 2006 г.
- ↑ Перейти обратно: Перейти обратно: а б с «Взаимодействующие галактики» . Суинбернский университет . Архивировано из оригинала 18 апреля 2023 года . Проверено 19 декабря 2006 г.
- ^ «С шестнадцатилетием, телескоп Хаббл!» . Служба новостей Хаббла (пресс-релиз). НАСА . 24 апреля 2006 года. Архивировано из оригинала 27 августа 2016 года . Проверено 10 августа 2006 г.
- ↑ Перейти обратно: Перейти обратно: а б «Звездные галактики» . Гарвард-Смитсоновский центр астрофизики . 29 августа 2006 г. Архивировано из оригинала 16 марта 2019 г. Проверено 10 августа 2006 г.
- ^ Кенникатт, Роберт С. младший; и др. (2005). «Демография и галактики-хозяева звездообразования». В Де Грийсе, Ричард; Гонсалес Дельгадо, Роза М. (ред.). Звездообразования: от 30 галактик Дораду до галактик Лаймана . Библиотека астрофизики и космических наук. Том. 329. Дордрехт: Спрингер . стр. 187–194. Бибкод : 2005ASSL..329..187K . дои : 10.1007/1-4020-3539-X_33 . ISBN 978-1-4020-3538-8 .
- ^ Смит, Джин (13 июля 2006 г.). «Звездные вспышки и сталкивающиеся галактики» . Калифорнийский университет, Центр астрофизики и космических наук Сан-Диего. Архивировано из оригинала 17 декабря 2023 года . Проверено 10 августа 2006 г.
- ^ Кил, Уильям К. (сентябрь 2006 г.). «Звездные галактики» . Университет Алабамы . Архивировано из оригинала 31 августа 2023 года . Проверено 11 декабря 2006 г.
- ^ Адамс, Дэвид Дж.; и др. (2004). Джонс, Марк Х.; Ламбурн, Роберт Дж.А. (ред.). Введение в галактики и космологию . Издательство Кембриджского университета . стр. 142–144. ISBN 978-0-521-54623-2 .
- ^ Кембхави, Аджит К.; Нарликар, Джаянт В. (2012) [1999]. «Свойства радио» . Квазары и активные ядра галактик: введение . Издательство Кембриджского университета . стр. 214–272. ISBN 978-1-139-17440-4 .
- ^ «Вводная астрономия: двухлопастные радиогалактики» . Кафедра астрономии Мэрилендского университета . Архивировано из оригинала 1 сентября 2022 года . Проверено 1 сентября 2022 г.
- ^ Оэй, Мартин SSL; ван Верен, Рейнаут Дж.; Хардкасл, Мартин Дж.; Боттеон, Андреа; Шимвелл, Тим В.; Дабхаде, Пратик; Гаст, Айвин РДЖГИБ; Ретгеринг, Хууб Дж.А.; Брюгген, Маркус; Тассе, Кирилл; Уильямс, Венди Л.; Шулевский, Александр (1 апреля 2022 г.). «Открытие радиогалактики размером не менее 5 Мпк» . Астрономия и астрофизика . 660 : А2. arXiv : 2202.05427 . Бибкод : 2022A&A...660A...2O . дои : 10.1051/0004-6361/202142778 . ISSN 0004-6361 .
- ^ Кондон, Джей-Джей (сентябрь 1992 г.). «Радиоизлучение нормальных галактик» . Ежегодный обзор астрономии и астрофизики . 30 (1): 575–611. Бибкод : 1992ARA&A..30..575C . дои : 10.1146/annurev.aa.30.090192.003043 . ISSN 0066-4146 .
- ^ Фракной и др. 2023 , с. 906 .
- ^ Закамская, Надя Л.; Штраус, Майкл А.; Кролик, Джулиан Х.; Коллиндж, Мэтью Дж.; Холл, Патрик Б.; Хао, Лей; Хекман, Тимоти М.; Ивези, элько; Ричардс, Гордон Т.; Шлегель, Дэвид Дж.; Шнайдер, Дональд П.; Стратева, «Искра»; Ванден Берк, Дэниел Э.; Андерсон, Скотт Ф.; Бринкманн, Джон (ноябрь 2003 г.). «Кандидаты в квазары типа II из Слоановского цифрового обзора неба. I. Выбор и оптические свойства выборки при 0,3 < Z» . Астрономический журнал . 126 (5): 2125–2144. arXiv : astro-ph/0309551 . дои : 10.1086/378610 . ISSN 0004-6256 .
- ^ Фракной и др. 2023 , стр. 907–915 .
- ↑ Перейти обратно: Перейти обратно: а б Кил, Уильям К. (2000). «Знакомство с активными ядрами галактик» . Университет Алабамы . Архивировано из оригинала 27 апреля 2012 года . Проверено 6 декабря 2006 г.
- ↑ Перейти обратно: Перейти обратно: а б Лохнер, Джим. Гибб, Мередит (ред.). «Монстр посередине» . Представьте себе Вселенную! . НАСА . Архивировано из оригинала 26 марта 2009 года . Проверено 20 декабря 2006 г.
- ^ Петерсон, Брэдли М. (1997). Введение в активные ядра галактик . Издательство Кембриджского университета . ISBN 978-0-521-47911-0 .
- ^ Петерсон, Брэдли М. (1 августа 2008 г.). «Центральная черная дыра и отношения с родительской галактикой» (PDF) . Новые обзоры астрономии . Активные ядра галактик при высочайшем угловом разрешении: теория и наблюдения. 52 (6): 240–252. Бибкод : 2008NewAR..52..240P . дои : 10.1016/j.newar.2008.06.005 . ISSN 1387-6473 . S2CID 121460317 .
- ^ Фракной и др. 2023 , стр. 899–906 .
- ^ Ковачевич, Анджелка Б; Йи, Тигфэн; Дай, Синьюй; Ян, Син; Чворович-Хайдиняк, Ива; Попович, Лука Ч (21 мая 2020 г.). «Подтверждена короткая периодическая переменность кандидата в сверхмассивную двойную черную дыру субпарсека Mrk 231» . Ежемесячные уведомления Королевского астрономического общества . 494 (3): 4069–4076. arXiv : 2003.06359 . дои : 10.1093/mnras/staa737 . ISSN 0035-8711 .
- ^ Немиров, Роберт; Боннелл, Джерри, ред. (10 ноября 2023 г.). «UHZ1: Далёкая галактика и чёрная дыра» . Астрономическая картина дня . НАСА , Мичиганский технологический университет . Проверено 18 февраля 2024 г.
- ^ Богдан, Акос; Гулдинг, Энди Д.; Натараджан, Приямвада; Ковач, Орсоля Э.; Трембле, Грант Р.; Чадаяммури, Урмила; Волонтери, Марта; Крафт, Ральф П.; Форман, Уильям Р.; Джонс, Кристина; Чуразов Евгений; Журавлева, Ирина (январь 2024 г.). «Доказательства тяжелого происхождения ранних сверхмассивных черных дыр из рентгеновского квазара az ≈ 10» . Природная астрономия . 8 (1): 126–133. arXiv : 2305.15458 . Бибкод : 2024NatAs...8..126B . дои : 10.1038/s41550-023-02111-9 . ISSN 2397-3366 . S2CID 258887541 .
- ^ Блэндфорд, Р.Д.; Нараян, Р. (сентябрь 1992 г.). «Космологические применения гравитационного линзирования» . Ежегодный обзор астрономии и астрофизики . 30 (1): 311–358. дои : 10.1146/annurev.aa.30.090192.001523 . ISSN 0066-4146 .
- ↑ Перейти обратно: Перейти обратно: а б Хекман, Тимоти М. (1980). «Оптический и радиообзор ядер ярких галактик» (PDF) . Астрономия и астрофизика . 87 (1–2): 152–164. Бибкод : 1980A&A....87..152H .
- ^ Хо, Луис К.; Филиппенко Алексей Владимирович; Сарджент, Уоллес LW (1997). «Поиски «карликовых» сейфертовских ядер. V. Демография ядерной активности в соседних галактиках». Астрофизический журнал . 487 (2): 568–578. arXiv : astro-ph/9704108 . Бибкод : 1997ApJ...487..568H . дои : 10.1086/304638 . S2CID 16742031 .
- ^ Перес-Торрес, Мигель; Маттила, Сеппо; Алонсо-Эрреро, Альмудена; Аалто, Сюзанна; Эфстатиу, Андреас (декабрь 2021 г.). «Звездообразование и ядерная активность в светящихся инфракрасных галактиках: инфракрасный обзор через радио» . Обзор астрономии и астрофизики . 29 (1): 2. arXiv : 2010.05072 . Бибкод : 2021A&ARv..29....2P . дои : 10.1007/s00159-020-00128-x . ISSN 0935-4956 . S2CID 253687419 .
- ↑ Перейти обратно: Перейти обратно: а б Беллокки, Э.; Перейра-Сантаэлла, М.; Колина, Л.; Лабиано, А.; Санчес-Гарсия, М.; Алонсо-Эрреро, А.; Аррибас, С.; Гарсиа-Бурильо, С.; Вильяр-Мартин, М.; Ригопулу, Д.; Валентино, Ф.; Пуглиси, А.; Диас-Сантос, Т.; Каццоли, С.; Усеро, А. (август 2022 г.). «Выбросы компактного молекулярного газа в локальных LIRG среди галактик с низким и высоким z» . Астрономия и астрофизика . 664 : А60. arXiv : 2204.02055 . Бибкод : 2022A&A...664A..60B . дои : 10.1051/0004-6361/202142802 . ISSN 0004-6361 .
- ^ Сандерс, Дэвид Б.; Мирабель, IF (сентябрь 1996 г.). «Светящиеся инфракрасные галактики» (PDF) . Ежегодный обзор астрономии и астрофизики . 34 (1): 749–792 [772–773]. Бибкод : 1996ARA&A..34..749S . дои : 10.1146/annurev.astro.34.1.749 . ISSN 0066-4146 .
- ^ Сэндедж, Крон и Лонгэр 1995 , стр. 43.
- ^ Сэндедж, Крон и Лонгэр 1995 , стр. 73.
- ↑ Перейти обратно: Перейти обратно: а б Чамба, Нушкия (2020). «Исторический взгляд на концепцию размера галактики» . Исследовательские заметки Американского астрономического общества . 4 (7): 117. arXiv : 2010.07946 . Бибкод : 2020RNAAS...4..117C . дои : 10.3847/2515-5172/aba951 . S2CID 223953458 .
- ^ Мушоцкий, Ричард. «Измерение светимости галактик» (PDF) . ASTR620: Галактики . Мэрилендского университета Астрономический факультет . Проверено 14 февраля 2024 г.
- ^ Спарк и Галлахер 2000 , 1.3.1
- ^ Фуке, П.; Патурел, Г. (сентябрь 1985 г.). «Стандартные фотометрические диаметры галактик. II — Редукция каталога ESO, UGC, MCG» . Астрономия и астрофизика . 150 (2): 192–204. Бибкод : 1985A&A...150..192F .
- ^ «Результаты по объекту Большое Магелланово Облако» . Внегалактическая база данных НАСА/IPAC . Калифорнийский технологический институт . Проверено 8 марта 2024 г.
- ^ «Результаты по объекту MESSIER 087 (NGC 4486)» . Внегалактическая база данных НАСА/IPAC . Калифорнийский технологический институт . Проверено 8 марта 2024 г.
- ^ «Результаты для объекта MESSIER 031 (Галактика Андромеды)» . Внегалактическая база данных НАСА/IPAC . Калифорнийский технологический институт . Проверено 8 марта 2024 г.
- ^ Де Вокулёр, Жерар (1948). «Исследование внегалактических туманностей» . Анналы астрофизики . 11 : 247. Бибкод : 1948АнАп...11..247Д .
- ^ Фиш, Роберт А. (1963). «Значение закона концентрации светимости в эллиптических галактиках» . Астрономический журнал . 68 : 72. Бибкод : 1963AJ.....68R..72F . дои : 10.1086/109075 .
- ^ Серсич, Хосе Луис (1968). «Об образовании галактик путем фрагментации» . Вестник астрономических институтов Чехословакии . 19 : 105. Бибкод : 1968BAICz..19..105S .
- ^ Конселиче, Кристофер Дж.; Бершади, Мэтью А.; Янгрен, Анна (2000). «Асимметрия галактик: физическая морфология близких галактик и галактик с большим красным смещением» . Астрофизический журнал . 529 (2): 886–910. arXiv : astro-ph/9907399 . Бибкод : 2000ApJ...529..886C . дои : 10.1086/308300 . S2CID 118962524 .
- ^ Бургарелла, Д.; Буат, В.; Донас, Дж.; Миллиард, Б.; Чапелон, С. (2001). «Ультрафиолетовая видимость и количественная морфология галактических дисков при низком и высоком красном смещении». Астрономия и астрофизика . 369 (2): 421–431. arXiv : astro-ph/0101344 . Бибкод : 2001A&A...369..421B . дои : 10.1051/0004-6361:20010107 . S2CID 858029 .
- ^ Лаубертс, Андрис; Валентин, Эдвин А. (1989). Каталог поверхностной фотометрии галактик ESO-Упсала . Бибкод : 1989spce.book.....L .
- ^ Петросян, Ваге (1976). «Поверхностная яркость и эволюция галактик» . Астрофизический журнал . 210 : Л53. Бибкод : 1976ApJ...209L...1P . дои : 10.1086/182301 .
- ^ «Петросянская величина» . Слоановский цифровой обзор неба . Архивировано из оригинала 2 августа 2023 года . Проверено 20 апреля 2024 г.
- ↑ Перейти обратно: Перейти обратно: а б Грэм, Алистер В.; Водитель, Саймон П.; Петросян, Ваге; Конселиче, Кристофер Дж.; Бершади, Мэтью А.; Кроуфорд, Стивен М.; Гото, Томоцугу (2005). «Общие величины галактик и эффективные радиусы по величинам и радиусам Петросяна». Астрономический журнал . 130 (4): 1535–1544. arXiv : astro-ph/0504287 . Бибкод : 2005AJ....130.1535G . дои : 10.1086/444475 . S2CID 11517686 .
- ^ Джаррет, Том; Розенберг, Джессика (6 февраля 1997 г.). «Круглые и фиксированные эллиптические апертуры: Петросиан и изофотальная фотометрия» . Сравнение фотометрии GALWORKS и STSDAS IRAF в полярном поле, Центр научных данных WISE . Калифорнийский технологический институт . Проверено 20 апреля 2024 г.
- ^ «Меры потока, величины и диаметра SDSS» . Внегалактическая база данных НАСА/IPAC . 19 июля 2017 г. Проверено 20 апреля 2024 г.
- ↑ Перейти обратно: Перейти обратно: а б Джарретт, TH; Честер, Т.; Кутри, Р.; Шнайдер, SE; Хухра, JP (2003). «Атлас больших галактик 2MASS» . Астрономический журнал . 125 (2): 525–554. Бибкод : 2003AJ....125..525J . дои : 10.1086/345794 . S2CID 117784410 .
- ^ Аргудо-Фернандес, М.; Верли, С.; Бергонд, Г.; Дуарте Пуэртас, С.; Рамос Кармона, Э.; Сабатер, Дж.; Фернандес Лоренцо, М.; Эспада, Д.; Сулентич, Дж.; Руис, Дж. Э.; Леон, С. (июнь 2015 г.). «Каталоги изолированных галактик, изолированных пар и изолированных тройек в локальной Вселенной» . Астрономия и астрофизика . 578 : А110. arXiv : 1504.00117 . Бибкод : 2015A&A...578A.110A . дои : 10.1051/0004-6361/201526016 . ISSN 0004-6361 .
- ^ Караченцев И.Д.; Макаров Д.И.; Караченцева В.Е.; Мельник О.В. (январь 2011 г.). «Каталог близких изолированных галактик в объёме z < 0,01» . Астрофизический вестник . 66 (1): 1–27. arXiv : 1103.3990 . Бибкод : 2011AstBu..66....1K . дои : 10.1134/S1990341311010019 . ISSN 1990-3413 .
- ^ Мельник О.; Караченцева В.; Караченцев И. (1 августа 2015 г.). «Скорость звездообразования в изолированных галактиках, выбранных из двухмикронного обзора всего неба» . Ежемесячные уведомления Королевского астрономического общества . 451 (2): 1482–1495. arXiv : 1504.07990 . дои : 10.1093/mnras/stv950 . ISSN 1365-2966 .
- ^ Хиршманн, Микаэла; Де Люсия, Габриэлла; Иовино, Анжела; Куччиати, Ольга (1 августа 2013 г.). «Изолированные галактики в иерархических моделях формирования галактик – современные свойства и история окружающей среды» . Ежемесячные уведомления Королевского астрономического общества . 433 (2): 1479–1491. arXiv : 1302.3616 . Бибкод : 2013MNRAS.433.1479H . дои : 10.1093/mnras/stt827 . ISSN 1365-2966 .
- ^ Ван, Вентинг; Уайт, Саймон Д.М. (21 августа 2012 г.). «Обилие спутников вокруг ярких изолированных галактик: Обилие спутников» . Ежемесячные уведомления Королевского астрономического общества . 424 (4): 2574–2598. arXiv : 1203.0009 . Бибкод : 2012MNRAS.424.2574W . дои : 10.1111/j.1365-2966.2012.21256.x .
- ^ «Группы и скопления галактик» . Рентгеновская обсерватория Чандра . Гарвард-Смитсоновский центр астрофизики . Архивировано из оригинала 22 февраля 2024 года . Проверено 15 января 2007 г.
- ^ Рикер, Пол. «Когда сталкиваются скопления галактик» . Суперкомпьютерный центр Сан-Диего . Калифорнийский университет, Сан-Диего . Архивировано из оригинала 2 августа 2023 года . Проверено 21 апреля 2024 г.
- ^ Помпеи, Эмануэла; Далем, Майкл; Иовино, Анжела (24 ноября 2006 г.). Далем, Майкл (ред.). «Оптический и радиообзор южных компактных групп галактик» . Бирмингемского университета Группа астрофизики и космических исследований . Архивировано из оригинала 13 июня 2007 года . Проверено 15 января 2007 г.
- ^ Понман, Тревор (25 февраля 2005 г.). «Галактические системы: Группы» . Бирмингемского университета Группа астрофизики и космических исследований . Архивировано из оригинала 15 февраля 2009 года . Проверено 15 января 2007 г.
- ^ Жирарди, Мариса; Джурицин, Г. (2000). «Наблюдательная функция масс свободных групп галактик». Астрофизический журнал . 540 (1): 45–56. arXiv : astro-ph/0004149 . Бибкод : 2000ApJ...540...45G . дои : 10.1086/309314 . S2CID 14059401 .
- ^ «Хаббл обнаружил самый дальний из когда-либо виденных протоскоплений галактик» (пресс-релиз). НАСА , Европейское космическое агентство . Архивировано из оригинала 12 июня 2018 года . Проверено 22 января 2015 г.
- ^ Дубински, Джон (1998). «Происхождение ярчайших скоплений галактик» . Астрофизический журнал . 502 (2): 141–149. arXiv : astro-ph/9709102 . Бибкод : 1998ApJ...502..141D . дои : 10.1086/305901 . S2CID 3137328 . Архивировано из оригинала 14 мая 2011 года.
- ^ «АТЛАСГАЛ Исследование Млечного Пути завершено» . Европейская южная обсерватория . Архивировано из оригинала 24 марта 2021 года . Проверено 7 марта 2016 г.
- ^ Бахколл, Нета А. (1988). «Крупномасштабная структура Вселенной, обозначенная скоплениями галактик». Ежегодный обзор астрономии и астрофизики . 26 (1): 631–686. Бибкод : 1988ARA&A..26..631B . дои : 10.1146/annurev.aa.26.090188.003215 .
- ^ Мандолези, Назарено; и др. (1986). «Крупномасштабная однородность Вселенной, измеренная по микроволновому фону». Письма к природе . 319 (6056): 751–753. Бибкод : 1986Natur.319..751M . дои : 10.1038/319751a0 . S2CID 4349689 .
- ^ Хорват, Иштван; Сова, Жолт; Хаккила, Джон; Тот, Л. Виктор (2015). «Новые данные подтверждают существование Великой стены Геркулеса – Северной Короны». Астрономия и астрофизика . 584 : А48. arXiv : 1510.01933 . Бибкод : 2015A&A...584A..48H . дои : 10.1051/0004-6361/201424829 . S2CID 56073380 .
- ^ Хорват, Иштван; Сова, Жолт; Хаккила, Джон; Тот, Виктор Л. (2014). «Аномалии пространственного распределения гамма-всплесков» . Proceedings of Science : 78. arXiv : 1507.05528 . Бибкод : 2014styd.confE..78H . дои : 10.22323/1.233.0078 .
- ^ ван ден Берг, Сидней (2000). «Обновленная информация о локальной группе». Публикации Тихоокеанского астрономического общества . 112 (770): 529–536. arXiv : astro-ph/0001040 . Бибкод : 2000PASP..112..529В . дои : 10.1086/316548 . S2CID 1805423 .
- ^ Талли, Ричард Брент (1982). «Локальное сверхскопление» . Астрофизический журнал . 257 : 389–422. Бибкод : 1982ApJ...257..389T . дои : 10.1086/159999 .
- ^ Темпель, Эльмо (1 сентября 2014 г.). «Космология: Знакомьтесь со сверхскоплением Ланиакея» . Природа . 513 (7516): 41–42. Бибкод : 2014Natur.513...41T . дои : 10.1038/513041a . ПМИД 25186896 .
- ↑ Перейти обратно: Перейти обратно: а б Бек, Райнер (2007). «Галактические магнитные поля» . Схоларпедия . 2 (8): 2411. Бибкод : 2007SchpJ...2.2411B . doi : 10.4249/scholarpedia.2411 .
- ^ «Тайны строительства галактического мегаполиса» (Пресс-релиз). Европейская южная обсерватория . Архивировано из оригинала 24 марта 2021 года . Проверено 15 октября 2014 г.
- ^ «Протогалактики» . Гарвард-Смитсоновский центр астрофизики . 18 ноября 1999 года. Архивировано из оригинала 25 марта 2008 года . Проверено 10 января 2007 г.
- ^ Фирмани, Дж.; Авила-Риз, Владимир (2003). «Физические процессы, лежащие в основе морфологической последовательности Хаббла». Мексиканский журнал астрономии и астрофизики . 17 : 107–120. arXiv : astro-ph/0303543 . Бибкод : 2003RMxAC..17..107F .
- ↑ Перейти обратно: Перейти обратно: а б с Даял, Пратика; Феррара, Андреа (декабрь 2018 г.). «Раннее формирование галактик и его крупномасштабные последствия» . Отчеты по физике . 780–782: 1–64. arXiv : 1809.09136 . Бибкод : 2018PhR...780....1D . дои : 10.1016/j.physrep.2018.10.002 .
- ↑ Перейти обратно: Перейти обратно: а б Клессен, Ральф С.; Гловер, Саймон, Колорадо (18 августа 2023 г.). «Первые звезды: образование, свойства и влияние» . Ежегодный обзор астрономии и астрофизики . 61 (1): 65–130. arXiv : 2303.12500 . Бибкод : 2023ARA&A..61...65K . doi : 10.1146/annurev-astro-071221-053453 . ISSN 0066-4146 .
- ^ Баркана, Реннан; Леб, Авраам (2001). «В начале: первые источники света и реионизация Вселенной» (PDF) . Отчеты по физике . 349 (2): 125–238. arXiv : astro-ph/0010468 . Бибкод : 2001PhR...349..125B . дои : 10.1016/S0370-1573(01)00019-9 . S2CID 119094218 . Архивировано из оригинала (PDF) 14 марта 2021 г.
- ^ Прощай, Деннис (17 июня 2015 г.). «Найдены следы древнейших звезд, обогативших космос» . Нью-Йорк Таймс . Архивировано из оригинала 29 июня 2019 года . Проверено 17 июня 2015 г.
- ^ Плуг Харо, Пол; Дикинсон, Марк; Финкельштейн, Стивен Л.; Открытка, Джейхан С.; Доннан, Каллум Т.; Бургарелла, Денис; Карналл, Адам С.; Каллен, Фергюс; Данлоп, Джеймс С.; Фернандес, Виталь; Фудзимото, Сэйдзи; Юнг, Интаэ; Криппс, Мелани; Ларсон, Ребекка Л.; Папович, Кейси (26 октября 2023 г.). «Подтверждение и опровержение очень ярких галактик в ранней Вселенной» . Природа 622 (7984): 707–711. arXiv : 2303.15431 . Бибкод : 2023Nature.622..707A . дои : 10.1038/ s41586-023-06521-7 ISSN 0028-0836 . ПМИД 37579792 .
- ^ Бойлан-Колчин, Майкл (13 апреля 2023 г.). «Стресс-тестирование ΛCDM с кандидатами в галактики с большим красным смещением» . Природная астрономия . 7 (6): 731–735. arXiv : 2208.01611 . Бибкод : 2023НатАс...7..731Б . дои : 10.1038/s41550-023-01937-7 . ISSN 2397-3366 . ПМЦ 10281863 . ПМИД 37351007 .
- ^ Собрал, Дэвид; и др. (4 июня 2015 г.). «Доказательства наличия POPIII-подобных звездных популяций в наиболее ярких излучателях LYMAN-α в эпоху реионизации: спектроскопическое подтверждение» . Астрофизический журнал . 808 (2): 139. arXiv : 1504.01734 . Бибкод : 2015ApJ...808..139S . дои : 10.1088/0004-637x/808/2/139 . S2CID 18471887 .
- ^ Боулер, RAA; Маклюр, Р.Дж.; Данлоп, Дж. С.; Маклеод, диджей; Стэнвей, скорая помощь; Элдридж, Джей-Джей; Джарвис, MJ (5 апреля 2017 г.). «Нет доказательств существования звезд населения III или черной дыры прямого коллапса в альфа-излучателе Лаймана 'CR7' с z = 6,6 » . Ежемесячные уведомления Королевского астрономического общества . 469 (1): 448–458. arXiv : 1609.00727 . дои : 10.1093/mnras/stx839 . ISSN 0035-8711 .
- ^ «Признаки самых ранних галактик» . Европейское космическое агентство . Архивировано из оригинала 6 августа 2020 года . Проверено 15 сентября 2015 г.
- ^ Longair 2008 , с. 583.
- ^ О'Каллаган, Джонатан (6 декабря 2022 г.). «Астрономы борются с открытием JWST ранних галактик» . Научный американец . Проверено 6 декабря 2022 г.
- ^ «Моделирование показывает, как растущие черные дыры регулируют формирование галактик» (пресс-релиз). Университет Карнеги-Меллон . 9 февраля 2005 года. Архивировано из оригинала 31 марта 2012 года . Проверено 7 января 2007 г.
- ^ Мэсси, Роберт; Хьюард, Анита (21 апреля 2007 г.). «Пойманы с поличным; формирующиеся галактики захвачены в молодой Вселенной» . Королевское астрономическое общество . Архивировано из оригинала 15 ноября 2013 года . Проверено 20 апреля 2007 г.
- ^ Ногучи, Масафуми (1999). «Ранняя эволюция дисковых галактик: образование выпуклостей в комковатых молодых галактических дисках». Астрофизический журнал . 514 (1): 77–95. arXiv : astro-ph/9806355 . Бибкод : 1999ApJ...514...77N . дои : 10.1086/306932 . S2CID 17963236 .
- ^ Боуг, Карлтон; Френк, Карлос (май 1999 г.). «Как устроены галактики?» . Институт физики . Архивировано из оригинала 26 апреля 2007 года . Проверено 16 января 2007 г.
- ^ Гонсалес, Гильермо (1998). Реболо, Рафаэль; Мартин, Эдуардо Л.; Осорио, Мария Роза Сапатеро (ред.). Звездная металличность — связь планет (PDF) . Коричневые карлики и внесолнечные планеты . Серия конференций ASP. Том. 134. стр. 431–437. Бибкод : 1998ASPC..134..431G .
- ^ Московиц, Клара (25 сентября 2012 г.). «Телескоп Хаббл открывает самый дальний вид во Вселенную» . Space.com . Архивировано из оригинала 5 мая 2020 года . Проверено 26 сентября 2012 г.
- ^ Рохас, Рэндалл Р.; Вогели, Майкл С.; Хойл, Фиона; Бринкманн, Джон (10 мая 2005 г.). «Спектроскопические свойства галактик войда в Слоанском цифровом обзоре неба» . Астрофизический журнал . 624 (2): 571–585. arXiv : astro-ph/0409074 . Бибкод : 2005ApJ...624..571R . дои : 10.1086/428476 . ISSN 0004-637X .
- ^ Конселиче, Кристофер Дж. (февраль 2007 г.). «Невидимая рука Вселенной» . Научный американец . Том. 296, нет. 2. С. 35–41. Бибкод : 2007SciAm.296b..34C . doi : 10.1038/scientificamerican0207-34 .
- ^ Форд, Х.; и др. (30 апреля 2002 г.). «Мыши (NGC 4676): столкновение галактик с хвостами звезд и газа» . Служба новостей Хаббла (пресс-релиз). НАСА . Архивировано из оригинала 7 сентября 2016 года . Проверено 8 мая 2007 г.
- ^ Удар, Кертис (1999). «Столкновения галактик». Отчеты по физике . 321 (1–3): 1–137. arXiv : astro-ph/9908269 . Бибкод : 1999PhR...321....1S . дои : 10.1016/S0370-1573(99)00030-7 . S2CID 119369136 .
- ^ Бузер, Роланд (2000). «Формирование и ранняя эволюция галактики Млечный Путь». Наука . 287 (5450): 69–74. Бибкод : 2000Sci...287...69B . дои : 10.1126/science.287.5450.69 . ПМИД 10615051 .
- ^ Круйссен, Дж. М. Дидерик; Пфеффер, Джоэл Л; Шеванс, Мелани; Бонака, Ана; Трухильо-Гомес, Себастьян; Бастиан, Нейт; Рейна-Кампос, Марта; Крэйн, Роберт А; Хьюз, Меган Э. (октябрь 2020 г.). «Кракен раскрывает себя — история слияния Млечного Пути, реконструированная с помощью моделирования E-MOSAICS» . Ежемесячные уведомления Королевского астрономического общества . 498 (2): 2472–2491. arXiv : 2003.01119 . дои : 10.1093/mnras/staa2452 .
- ^ Янг, Моника (13 ноября 2020 г.). «Звездные скопления открывают «Кракена» в прошлом Млечного Пути» . Небо и телескоп . Архивировано из оригинала 15 ноября 2020 года . Проверено 15 ноября 2020 г.
- ^ Пантер, Б.; Хименес, Р.; Небеса, А.Ф.; Шарло, С. (2007). «История звездообразования галактик в Слоанском цифровом обзоре неба». Ежемесячные уведомления Королевского астрономического общества . 378 (4): 1550–1564. arXiv : astro-ph/0608531 . Бибкод : 2007MNRAS.378.1550P . дои : 10.1111/j.1365-2966.2007.11909.x . S2CID 15174718 .
- ^ Кенникатт, Роберт С. младший; Тэмблин, Питер; Конгдон, Чарльз Э. (1994). «Прошлое и будущее звездообразование в дисковых галактиках». Астрофизический журнал . 435 (1): 22–36. Бибкод : 1994ApJ...435...22K . дои : 10.1086/174790 .
- ^ Кнапп, Джиллиан Р. (1999). Звездообразование в галактиках ранних типов . Том. 163. Тихоокеанское астрономическое общество . п. 119. arXiv : astro-ph/9808266 . Бибкод : 1999ASPC..163..119K . ISBN 978-1-886733-84-8 . OCLC 41302839 .
- ↑ Перейти обратно: Перейти обратно: а б Адамс, Фред; Лафлин, Грег (13 июля 2006 г.). «Великая космическая битва» . Астрономическое общество Тихого океана . Архивировано из оригинала 13 мая 2012 года . Проверено 16 января 2007 г.
- ^ Чой, Чарльз К. (13 мая 2015 г.). «Разгадана космическая «тайна убийства»: галактики «задушены» » . Space.com . Архивировано из оригинала 24 марта 2021 года . Проверено 14 мая 2015 г.
- ^ Побоевски, Салли (21 января 1997 г.). «Физика предлагает заглянуть на темную сторону Вселенной» . Мичиганский университет . Архивировано из оригинала 21 января 2005 года . Проверено 13 января 2007 г.
- ^ «Уэбб раскрывает структуру 19 спиральных галактик» . ЕКА . 29 января 2024 г. . Проверено 30 января 2024 г.
Библиография
- Белкора, Лейла (2003). Заботясь о небесах: история открытия Млечного Пути . ЦРК Пресс . ISBN 978-0-7503-0730-7 . Архивировано из оригинала 24 марта 2021 года . Проверено 25 июля 2018 г.
- Бертен, Джузеппе ; Линь, Цзя-Чяо (1996). Спиральная структура в галактиках: теория волн плотности . МТИ Пресс . ISBN 978-0-262-02396-2 . Архивировано из оригинала 24 марта 2021 года . Проверено 25 июля 2018 г.
- Бинни, Джеймс ; Меррифилд, Майкл (1998). Галактическая астрономия . Издательство Принстонского университета. ISBN 978-0-691-00402-0 . ОСЛК 39108765 . Архивировано из оригинала 24 марта 2021 года . Проверено 25 июля 2018 г.
- Дикинсон, Теренс (2004). Вселенная и за ее пределами (4-е изд.). Книги Светлячка. ISBN 978-1-55297-901-3 . OCLC 55596414 .
- Фракной, Эндрю ; и др. (2023). Астрономия 2е . ОпенСтакс . ISBN 978-1-951693-50-3 .
- Гейдарзаде, Тофиг (2008). История физических теорий комет от Аристотеля до Уиппла . Спрингер. ISBN 978-1-4020-8322-8 . Архивировано из оригинала 24 марта 2021 года . Проверено 25 июля 2018 г.
- Мо, Ходжун; ван ден Бош, Фрэнк; Уайт, Саймон (2010). Формирование и эволюция галактик . Издательство Кембриджского университета . ISBN 978-0-521-85793-2 . Архивировано из оригинала 24 марта 2021 года . Проверено 25 июля 2018 г.
- Кеппл, Джордж Р.; Саннер, Глен В. (1998). Путеводитель наблюдателя за ночным небом, Том 1 . Вильманн-Белл . ISBN 978-0-943396-58-3 . Архивировано из оригинала 24 марта 2021 года . Проверено 25 июля 2018 г.
- Лонгэйр, Малкольм С. (2008). Формирование галактик . Библиотека астрономии и астрофизики (2-е изд.). Берлин: Шпрингер . дои : 10.1007/978-3-540-73478-9 . ISBN 978-3-540-73477-2 .
- Мерритт, Дэвид Р. (2013). Динамика и эволюция галактических ядер . Издательство Принстонского университета . ISBN 978-1-4008-4612-2 . Архивировано из оригинала 24 марта 2021 года . Проверено 25 июля 2018 г.
- Мохамед, Мохайни (2000). Великие мусульманские математики . Издательство УТМ . ISBN 978-983-52-0157-8 . OCLC 48759017 . Архивировано из оригинала 24 марта 2021 года . Проверено 25 июля 2018 г.
- Пол, Эрих Р. (1993). Галактика Млечный Путь и статистическая космология, 1890–1924 гг . Издательство Кембриджского университета . ISBN 978-0-521-35363-2 . Архивировано из оригинала 24 марта 2021 года . Проверено 25 июля 2018 г.
- Сэндидж, Аллан Р .; Крон, Ричард Г.; Лонгэр, Малкольм С. (1995). Глубокая Вселенная . Курс повышения квалификации Саас-Фе, Швейцарское общество астрофизики и астрономии. Том. 23. Шпрингер-Верлаг . ISBN 978-3-54-058913-6 .
- Спарк, Линда С .; Галлахер, Джон С. (2000). Галактики во Вселенной: Введение . Издательство Кембриджского университета . ISBN 978-0-521-59740-1 . Архивировано из оригинала 24 марта 2021 года . Проверено 25 июля 2018 г.
- Ван ден Берг, Сидней (1998). Морфология и классификация галактик . Издательство Кембриджского университета. ISBN 978-0-521-62335-3 . Архивировано из оригинала 24 марта 2021 года . Проверено 25 июля 2018 г.
- Уоллер, Уильям Х.; Ходж, Пол В. (2003). Галактики и космический рубеж . Издательство Гарвардского университета . ISBN 978-0-674-01079-6 .
- Райт, Томас (1750). Оригинальная теория или новая гипотеза Вселенной . Лондон: Чапель. Архивировано из оригинала 30 апреля 2021 года . Проверено 21 апреля 2024 г.
... звезды не рассеяны бесконечно и не распределены беспорядочным образом по всему земному пространству, без порядка или замысла, ... это явление [является] не чем иным, как определенным эффектом, возникающим из положения наблюдателя, ... Чтобы зритель, помещенный в неопределенное пространство, ... это [т. е. Млечный Путь ( Via Lactea )] [представляет собой] обширное кольцо звезд ...
Внешние ссылки
- Внегалактическая база данных НАСА/IPAC (NED)
- Расстояния, независимые от красного смещения NED
- Галактики в программе «В наше время » на BBC
- Атлас Вселенной
- Галактики – Информация и любительские наблюдения
- Galaxy Zoo - проект гражданской науки по классификации галактик
- «Полет через Вселенную, проведенный Sloan Digital Sky Survey» - анимационное видео из лаборатории Беркли.