Семейство архитектуры ARM
![]() | |
Дизайнер | |
---|---|
Биты | 32-bit , 64-bit |
Представлено | 1985 год |
Дизайн | РИСК |
Тип | Загрузка-сохранение |
Ветвление | Код состояния , сравнение и ветвление |
Открыть | Собственный |
Introduced | 2011 |
---|---|
Version | ARMv8-R, ARMv8-A, ARMv8.1-A, ARMv8.2-A, ARMv8.3-A, ARMv8.4-A, ARMv8.5-A, ARMv8.6-A, ARMv8.7-A, ARMv8.8-A, ARMv8.9-A, ARMv9.0-A, ARMv9.1-A, ARMv9.2-A, ARMv9.3-A, ARMv9.4-A |
Encoding | AArch64/A64 and AArch32/A32 use 32-bit instructions, AArch32/T32 (Thumb-2) uses mixed 16- and 32-bit instructions[1] |
Endianness | Bi (little as default) |
Extensions | SVE, SVE2, SME, AES, SHA, TME; All mandatory: Thumb-2, Neon, VFPv4-D16, VFPv4; obsolete: Jazelle |
Registers | |
General-purpose | 31 × 64-bit integer registers[1] |
Floating point | 32 × 128-bit registers[1] for scalar 32- and 64-bit FP or SIMD FP or integer; or cryptography |
Version | ARMv9-R, ARMv9-M, ARMv8-R, ARMv8-M, ARMv7-A, ARMv7-R, ARMv7E-M, ARMv7-M, ARMv6-M |
---|---|
Encoding | 32-bit, except Thumb-2 extensions use mixed 16- and 32-bit instructions. |
Endianness | Bi (little as default) |
Extensions | Thumb-2, Neon, Jazelle, AES, SHA, DSP, Saturated, FPv4-SP, FPv5, Helium |
Registers | |
General-purpose | 15 × 32-bit integer registers, including R14 (link register), but not R15 (PC) |
Floating point | Up to 32 × 64-bit registers,[2] SIMD/floating-point (optional) |
Version | ARMv6, ARMv5, ARMv4T, ARMv3, ARMv2 |
---|---|
Encoding | 32-bit, except Thumb extension uses mixed 16- and 32-bit instructions. |
Endianness | Bi (little as default) in ARMv3 and above |
Extensions | Thumb, Jazelle |
Registers | |
General-purpose | 15 × 32-bit integer registers, including R14 (link register), but not R15 (PC, 26-bit addressing in older) |
Floating point | None |
ARM (стилизованное в нижнем регистре как «arm» , ранее аббревиатура от Advanced RISC Machines и первоначально Acorn RISC Machine ) — это семейство RISC архитектур набора команд (ISA) для компьютерных процессоров . Arm Ltd. разрабатывает ISA и лицензирует их другим компаниям, которые создают физические устройства, использующие этот набор команд. Он также разрабатывает и лицензирует ядра , реализующие эти ISA.
Благодаря своей низкой стоимости, низкому энергопотреблению и низкому выделению тепла процессоры ARM полезны для легких портативных устройств с батарейным питанием, включая смартфоны , ноутбуки и планшетные компьютеры , а также во встроенных системах . [3] [4] [5] Однако процессоры ARM также используются для настольных компьютеров и серверов , включая самый быстрый в мире суперкомпьютер ( Fugaku ) 2020 года. [6] до 2022 года. Произведено более 230 миллиардов чипов ARM, [7] [8] [9] по состоянию на 2022 год [update]ARM — наиболее широко используемое семейство архитектур набора команд. [10] [4] [11] [12] [13]
There have been several generations of the ARM design. The original ARM1 used a 32-bit internal structure but had a 26-bit address space that limited it to 64 MB of main memory. This limitation was removed in the ARMv3 series, which has a 32-bit address space, and several additional generations up to ARMv7 remained 32-bit. Released in 2011, the ARMv8-A architecture added support for a 64-bit address space and 64-bit arithmetic with its new 32-bit fixed-length instruction set.[14] Arm Ltd. has also released a series of additional instruction sets for different rules; the "Thumb" extension adds both 32- and 16-bit instructions for improved code density, while Jazelle added instructions for directly handling Java bytecode. More recent changes include the addition of simultaneous multithreading (SMT) for improved performance or fault tolerance.[15]
History
[edit]BBC Micro
[edit]Acorn Computers' first widely successful design was the BBC Micro, introduced in December 1981. This was a relatively conventional machine based on the MOS Technology 6502 CPU but ran at roughly double the performance of competing designs like the Apple II due to its use of faster dynamic random-access memory (DRAM). Typical DRAM of the era ran at about 2 MHz; Acorn arranged a deal with Hitachi for a supply of faster 4 MHz parts.[16]
Machines of the era generally shared memory between the processor and the framebuffer, which allowed the processor to quickly update the contents of the screen without having to perform separate input/output (I/O). As the timing of the video display is exacting, the video hardware had to have priority access to that memory. Due to a quirk of the 6502's design, the CPU left the memory untouched for half of the time. Thus by running the CPU at 1 MHz, the video system could read data during those down times, taking up the total 2 MHz bandwidth of the RAM. In the BBC Micro, the use of 4 MHz RAM allowed the same technique to be used, but running at twice the speed. This allowed it to outperform any similar machine on the market.[17]
Acorn Business Computer
[edit]1981 was also the year that the IBM Personal Computer was introduced. Using the recently introduced Intel 8088, a 16-bit CPU compared to the 6502's 8-bit design, it offered higher overall performance. Its introduction changed the desktop computer market radically: what had been largely a hobby and gaming market emerging over the prior five years began to change to a must-have business tool where the earlier 8-bit designs simply could not compete. Even newer 32-bit designs were also coming to market, such as the Motorola 68000[18] and National Semiconductor NS32016.[19]
Acorn began considering how to compete in this market and produced a new paper design named the Acorn Business Computer. They set themselves the goal of producing a machine with ten times the performance of the BBC Micro, but at the same price.[20] This would outperform and underprice the PC. At the same time, the recent introduction of the Apple Lisa brought the graphical user interface (GUI) concept to a wider audience and suggested the future belonged to machines with a GUI.[21] The Lisa, however, cost $9,995, as it was packed with support chips, large amounts of memory, and a hard disk drive, all very expensive then.[22]
The engineers then began studying all of the CPU designs available. Their conclusion about the existing 16-bit designs was that they were a lot more expensive and were still "a bit crap",[23] offering only slightly higher performance than their BBC Micro design. They also almost always demanded a large number of support chips to operate even at that level, which drove up the cost of the computer as a whole. These systems would simply not hit the design goal.[23] They also considered the new 32-bit designs, but these cost even more and had the same issues with support chips.[24] According to Sophie Wilson, all the processors tested at that time performed about the same, with about a 4 Mbit/s bandwidth.[25][a]
Two key events led Acorn down the path to ARM. One was the publication of a series of reports from the University of California, Berkeley, which suggested that a simple chip design could nevertheless have extremely high performance, much higher than the latest 32-bit designs on the market.[26] The second was a visit by Steve Furber and Sophie Wilson to the Western Design Center, a company run by Bill Mensch and his sister, which had become the logical successor to the MOS team and was offering new versions like the WDC 65C02. The Acorn team saw high school students producing chip layouts on Apple II machines, which suggested that anyone could do it.[27][28] In contrast, a visit to another design firm working on modern 32-bit CPU revealed a team with over a dozen members who were already on revision H of their design and yet it still contained bugs.[b] This cemented their late 1983 decision to begin their own CPU design, the Acorn RISC Machine.[29]
Design concepts
[edit]The original Berkeley RISC designs were in some sense teaching systems, not designed specifically for outright performance. To the RISC's basic register-heavy and load/store concepts, ARM added a number of the well-received design notes of the 6502. Primary among them was the ability to quickly serve interrupts, which allowed the machines to offer reasonable input/output performance with no added external hardware. To offer interrupts with similar performance as the 6502, the ARM design limited its physical address space to 64 MB of total addressable space, requiring 26 bits of address. As instructions were 4 bytes (32 bits) long, and required to be aligned on 4-byte boundaries, the lower 2 bits of an instruction address were always zero. This meant the program counter (PC) only needed to be 24 bits, allowing it to be stored along with the eight bit processor flags in a single 32-bit register. That meant that upon receiving an interrupt, the entire machine state could be saved in a single operation, whereas had the PC been a full 32-bit value, it would require separate operations to store the PC and the status flags. This decision halved the interrupt overhead.[30]
Another change, and among the most important in terms of practical real-world performance, was the modification of the instruction set to take advantage of page mode DRAM. Recently introduced, page mode allowed subsequent accesses of memory to run twice as fast if they were roughly in the same location, or "page", in the DRAM chip. Berkeley's design did not consider page mode and treated all memory equally. The ARM design added special vector-like memory access instructions, the "S-cycles", that could be used to fill or save multiple registers in a single page using page mode. This doubled memory performance when they could be used, and was especially important for graphics performance.[31]
The Berkeley RISC designs used register windows to reduce the number of register saves and restores performed in procedure calls; the ARM design did not adopt this.
Wilson developed the instruction set, writing a simulation of the processor in BBC BASIC that ran on a BBC Micro with a second 6502 processor.[32][33] This convinced Acorn engineers they were on the right track. Wilson approached Acorn's CEO, Hermann Hauser, and requested more resources. Hauser gave his approval and assembled a small team to design the actual processor based on Wilson's ISA.[34] The official Acorn RISC Machine project started in October 1983.
ARM1
[edit]
Acorn chose VLSI Technology as the "silicon partner", as they were a source of ROMs and custom chips for Acorn. Acorn provided the design and VLSI provided the layout and production. The first samples of ARM silicon worked properly when first received and tested on 26 April 1985.[3] Known as ARM1, these versions ran at 6 MHz.[35]
The first ARM application was as a second processor for the BBC Micro, where it helped in developing simulation software to finish development of the support chips (VIDC, IOC, MEMC), and sped up the CAD software used in ARM2 development. Wilson subsequently rewrote BBC BASIC in ARM assembly language. The in-depth knowledge gained from designing the instruction set enabled the code to be very dense, making ARM BBC BASIC an extremely good test for any ARM emulator.
ARM2
[edit]The result of the simulations on the ARM1 boards led to the late 1986 introduction of the ARM2 design running at 8 MHz, and the early 1987 speed-bumped version at 10 to 12 MHz.[c] A significant change in the underlying architecture was the addition of a Booth multiplier, whereas formerly multiplication had to be carried out in software.[37] Further, a new Fast Interrupt reQuest mode, FIQ for short, allowed registers 8 through 14 to be replaced as part of the interrupt itself. This meant FIQ requests did not have to save out their registers, further speeding interrupts.[38]
The first use of the ARM2 was the Acorn Archimedes personal computer models A305, A310, and A440 launched in 1987.
According to the Dhrystone benchmark, the ARM2 was roughly seven times the performance of a typical 7 MHz 68000-based system like the Amiga or Macintosh SE. It was twice as fast as an Intel 80386 running at 16 MHz, and about the same speed as a multi-processor VAX-11/784 superminicomputer. The only systems that beat it were the Sun SPARC and MIPS R2000 RISC-based workstations.[39] Further, as the CPU was designed for high-speed I/O, it dispensed with many of the support chips seen in these machines; notably, it lacked any dedicated direct memory access (DMA) controller which was often found on workstations. The graphics system was also simplified based on the same set of underlying assumptions about memory and timing. The result was a dramatically simplified design, offering performance on par with expensive workstations but at a price point similar to contemporary desktops.[39]
The ARM2 featured a 32-bit data bus, 26-bit address space and 27 32-bit registers, of which 16 are accessible at any one time (including the PC).[40] The ARM2 had a transistor count of just 30,000,[41] compared to Motorola's six-year-older 68000 model with around 68,000. Much of this simplicity came from the lack of microcode, which represents about one-quarter to one-third of the 68000's transistors, and the lack of (like most CPUs of the day) a cache. This simplicity enabled the ARM2 to have a low power consumption and simpler thermal packaging, through having fewer powered transistors, yet offering better performance than the contemporary, 1987, the IBM PS/2 Model 50, which initially utilised an Intel 80286, offering 1.8 MIPS @ 10 MHz, and later in 1987, the 2 MIPS of the PS/2 70, with its Intel 386 DX @ 16 MHz.[42][43]
A successor, ARM3, was produced with a 4 KB cache, which further improved performance.[44] The address bus was extended to 32 bits in the ARM6, but program code still had to lie within the first 64 MB of memory in 26-bit compatibility mode, due to the reserved bits for the status flags.[45]
Advanced RISC Machines Ltd. – ARM6
[edit]
In the late 1980s, Apple Computer and VLSI Technology started working with Acorn on newer versions of the ARM core. In 1990, Acorn spun off the design team into a new company named Advanced RISC Machines Ltd.,[46][47][48] which became ARM Ltd. when its parent company, Arm Holdings plc, floated on the London Stock Exchange and Nasdaq in 1998.[49] The new Apple–ARM work would eventually evolve into the ARM6, first released in early 1992. Apple used the ARM6-based ARM610 as the basis for their Apple Newton PDA.
Early licensees
[edit]In 1994, Acorn used the ARM610 as the main central processing unit (CPU) in their RiscPC computers. DEC licensed the ARMv4 architecture and produced the StrongARM.[50] At 233 MHz, this CPU drew only one watt (newer versions draw far less). This work was later passed to Intel as part of a lawsuit settlement, and Intel took the opportunity to supplement their i960 line with the StrongARM. Intel later developed its own high performance implementation named XScale, which it has since sold to Marvell. Transistor count of the ARM core remained essentially the same throughout these changes; ARM2 had 30,000 transistors,[51] while ARM6 grew only to 35,000.[52]
Market share
[edit]In 2005, about 98% of all mobile phones sold used at least one ARM processor.[53] In 2010, producers of chips based on ARM architectures reported shipments of 6.1 billion ARM-based processors, representing 95% of smartphones, 35% of digital televisions and set-top boxes, and 10% of mobile computers. In 2011, the 32-bit ARM architecture was the most widely used architecture in mobile devices and the most popular 32-bit one in embedded systems.[54] In 2013, 10 billion were produced[55] and "ARM-based chips are found in nearly 60 percent of the world's mobile devices".[56]
Licensing
[edit]
Core licence
[edit]Arm Ltd.'s primary business is selling IP cores, which licensees use to create microcontrollers (MCUs), CPUs, and systems-on-chips based on those cores. The original design manufacturer combines the ARM core with other parts to produce a complete device, typically one that can be built in existing semiconductor fabrication plants (fabs) at low cost and still deliver substantial performance. The most successful implementation has been the ARM7TDMI with hundreds of millions sold. Atmel has been a precursor design center in the ARM7TDMI-based embedded system.
The ARM architectures used in smartphones, PDAs and other mobile devices range from ARMv5 to ARMv8-A.
In 2009, some manufacturers introduced netbooks based on ARM architecture CPUs, in direct competition with netbooks based on Intel Atom.[57]
Arm Ltd. offers a variety of licensing terms, varying in cost and deliverables. Arm Ltd. provides to all licensees an integratable hardware description of the ARM core as well as complete software development toolset (compiler, debugger, software development kit), and the right to sell manufactured silicon containing the ARM CPU.
SoC packages integrating ARM's core designs include Nvidia Tegra's first three generations, CSR plc's Quatro family, ST-Ericsson's Nova and NovaThor, Silicon Labs's Precision32 MCU, Texas Instruments's OMAP products, Samsung's Hummingbird and Exynos products, Apple's A4, A5, and A5X, and NXP's i.MX.
Fabless licensees, who wish to integrate an ARM core into their own chip design, are usually only interested in acquiring a ready-to-manufacture verified semiconductor intellectual property core. For these customers, Arm Ltd. delivers a gate netlist description of the chosen ARM core, along with an abstracted simulation model and test programs to aid design integration and verification. More ambitious customers, including integrated device manufacturers (IDM) and foundry operators, choose to acquire the processor IP in synthesizable RTL (Verilog) form. With the synthesizable RTL, the customer has the ability to perform architectural level optimisations and extensions. This allows the designer to achieve exotic design goals not otherwise possible with an unmodified netlist (high clock speed, very low power consumption, instruction set extensions, etc.). While Arm Ltd. does not grant the licensee the right to resell the ARM architecture itself, licensees may freely sell manufactured products such as chip devices, evaluation boards and complete systems. Merchant foundries can be a special case; not only are they allowed to sell finished silicon containing ARM cores, they generally hold the right to re-manufacture ARM cores for other customers.
Arm Ltd. prices its IP based on perceived value. Lower performing ARM cores typically have lower licence costs than higher performing cores. In implementation terms, a synthesisable core costs more than a hard macro (blackbox) core. Complicating price matters, a merchant foundry that holds an ARM licence, such as Samsung or Fujitsu, can offer fab customers reduced licensing costs. In exchange for acquiring the ARM core through the foundry's in-house design services, the customer can reduce or eliminate payment of ARM's upfront licence fee.
Compared to dedicated semiconductor foundries (such as TSMC and UMC) without in-house design services, Fujitsu/Samsung charge two- to three-times more per manufactured wafer.[citation needed] For low to mid volume applications, a design service foundry offers lower overall pricing (through subsidisation of the licence fee). For high volume mass-produced parts, the long term cost reduction achievable through lower wafer pricing reduces the impact of ARM's NRE (non-recurring engineering) costs, making the dedicated foundry a better choice.
Companies that have developed chips with cores designed by Arm include Amazon.com's Annapurna Labs subsidiary,[58] Analog Devices, Apple, AppliedMicro (now: MACOM Technology Solutions[59]), Atmel, Broadcom, Cavium, Cypress Semiconductor, Freescale Semiconductor (now NXP Semiconductors), Huawei, Intel,[dubious – discuss] Maxim Integrated, Nvidia, NXP, Qualcomm, Renesas, Samsung Electronics, ST Microelectronics, Texas Instruments, and Xilinx.
Built on ARM Cortex Technology licence
[edit]In February 2016, ARM announced the Built on ARM Cortex Technology licence, often shortened to Built on Cortex (BoC) licence. This licence allows companies to partner with ARM and make modifications to ARM Cortex designs. These design modifications will not be shared with other companies. These semi-custom core designs also have brand freedom, for example Kryo 280.
Companies that are current licensees of Built on ARM Cortex Technology include Qualcomm.[60]
Architectural licence
[edit]Companies can also obtain an ARM architectural licence for designing their own CPU cores using the ARM instruction sets. These cores must comply fully with the ARM architecture. Companies that have designed cores that implement an ARM architecture include Apple, AppliedMicro (now: Ampere Computing), Broadcom, Cavium (now: Marvell), Digital Equipment Corporation, Intel, Nvidia, Qualcomm, Samsung Electronics, Fujitsu, and NUVIA Inc. (acquired by Qualcomm in 2021).
ARM Flexible Access
[edit]On 16 July 2019, ARM announced ARM Flexible Access. ARM Flexible Access provides unlimited access to included ARM intellectual property (IP) for development. Per product licence fees are required once a customer reaches foundry tapeout or prototyping.[61][62]
75% of ARM's most recent IP over the last two years are included in ARM Flexible Access. As of October 2019:
- CPUs: Cortex-A5, Cortex-A7, Cortex-A32, Cortex-A34, Cortex-A35, Cortex-A53, Cortex-R5, Cortex-R8, Cortex-R52, Cortex-M0, Cortex-M0+, Cortex-M3, Cortex-M4, Cortex-M7, Cortex-M23, Cortex-M33
- GPUs: Mali-G52, Mali-G31. Includes Mali Driver Development Kits (DDK).
- Interconnect: CoreLink NIC-400, CoreLink NIC-450, CoreLink CCI-400, CoreLink CCI-500, CoreLink CCI-550, ADB-400 AMBA, XHB-400 AXI-AHB
- System Controllers: CoreLink GIC-400, CoreLink GIC-500, PL192 VIC, BP141 TrustZone Memory Wrapper, CoreLink TZC-400, CoreLink L2C-310, CoreLink MMU-500, BP140 Memory Interface
- Security IP: CryptoCell-312, CryptoCell-712, TrustZone True Random Number Generator
- Peripheral Controllers: PL011 UART, PL022 SPI, PL031 RTC
- Debug & Trace: CoreSight SoC-400, CoreSight SDC-600, CoreSight STM-500, CoreSight System Trace Macrocell, CoreSight Trace Memory Controller
- Design Kits: Corstone-101, Corstone-201
- Physical IP: Artisan PIK for Cortex-M33 TSMC 22ULL including memory compilers, logic libraries, GPIOs and documentation
- Tools & Materials: Socrates IP ToolingARM Design Studio, Virtual System Models
- Support: Standard ARM Technical support, ARM online training, maintenance updates, credits toward onsite training and design reviews
Cores
[edit]Architecture | Core bit-width |
Cores | Profile | Refe- rences | |
---|---|---|---|---|---|
Arm Ltd. | Third-party | ||||
ARMv1 |
ARM1 | Classic |
|||
ARMv2 |
32 |
ARM2, ARM250, ARM3 | Amber, STORM Open Soft Core[63] | Classic |
|
ARMv3 |
32 |
ARM6, ARM7 | Classic |
||
ARMv4 |
32 |
ARM8 | StrongARM, FA526, ZAP Open Source Processor Core | Classic |
|
ARMv4T |
32 |
ARM7TDMI, ARM9TDMI, SecurCore SC100 | Classic |
||
ARMv5TE |
32 |
ARM7EJ, ARM9E, ARM10E | XScale, FA626TE, Feroceon, PJ1/Mohawk | Classic |
|
ARMv6 |
32 |
ARM11 | Classic |
||
ARMv6-M |
32 |
ARM Cortex-M0, ARM Cortex-M0+, ARM Cortex-M1, SecurCore SC000 | |||
ARMv7-M |
32 |
ARM Cortex-M3, SecurCore SC300 | Apple M7 motion coprocessor | Microcontroller |
|
ARMv7E-M |
32 |
ARM Cortex-M4, ARM Cortex-M7 | Microcontroller |
||
ARMv8-M |
32 |
ARM Cortex-M23,[65] ARM Cortex-M33[66] | Microcontroller |
||
ARMv8.1-M
|
32
|
ARM Cortex-M55, ARM Cortex-M85 | Microcontroller
|
||
ARMv7-R |
32 |
ARM Cortex-R4, ARM Cortex-R5, ARM Cortex-R7, ARM Cortex-R8 | |||
ARMv8-R |
32 |
ARM Cortex-R52 | Real-time |
||
64
|
ARM Cortex-R82 | Real-time
|
|||
ARMv7-A |
32 |
ARM Cortex-A5, ARM Cortex-A7, ARM Cortex-A8, ARM Cortex-A9, ARM Cortex-A12, ARM Cortex-A15, ARM Cortex-A17 | Qualcomm Scorpion/Krait, PJ4/Sheeva, Apple Swift (A6, A6X) | ||
ARMv8-A |
32 |
ARM Cortex-A32[72] | Application |
||
64/32 |
ARM Cortex-A35,[73] ARM Cortex-A53, ARM Cortex-A57,[74] ARM Cortex-A72,[75] ARM Cortex-A73[76] | X-Gene, Nvidia Denver 1/2, Cavium ThunderX, AMD K12, Apple Cyclone (A7)/Typhoon (A8, A8X)/Twister (A9, A9X)/Hurricane+Zephyr (A10, A10X), Qualcomm Kryo, Samsung M1/M2 ("Mongoose") /M3 ("Meerkat") | Application |
||
ARM Cortex-A34[82] | Application
|
||||
ARMv8.1-A |
64/32 |
TBA | Cavium ThunderX2 | Application |
|
ARMv8.2-A |
64/32 |
ARM Cortex-A55,[84] ARM Cortex-A75,[85] ARM Cortex-A76,[86] ARM Cortex-A77, ARM Cortex-A78, ARM Cortex-X1, ARM Neoverse N1 | Nvidia Carmel, Samsung M4 ("Cheetah"), Fujitsu A64FX (ARMv8 SVE 512-bit) | Application |
|
64 |
ARM Cortex-A65, ARM Neoverse E1 with simultaneous multithreading (SMT), ARM Cortex-A65AE[90] (also having e.g. ARMv8.4 Dot Product; made for safety critical tasks such as advanced driver-assistance systems (ADAS)) | Apple Monsoon+Mistral (A11) (September 2017) | Application |
||
ARMv8.3-A
|
64/32 |
TBA | Application
|
||
64 |
TBA | Apple Vortex+Tempest (A12, A12X, A12Z), Marvell ThunderX3 (v8.3+)[91] | Application |
||
ARMv8.4-A |
64/32 |
TBA | Application |
||
64 |
ARM Neoverse V1 | Apple Lightning+Thunder (A13), Apple Firestorm+Icestorm (A14, M1) | Application |
||
ARMv8.5-A
|
64/32 |
TBA | Application
|
||
64 |
TBA | Application
|
|||
ARMv8.6-A
|
64 |
TBA | Apple Avalanche+Blizzard (A15, M2), Apple Everest+Sawtooth (A16)[92] | Application
|
|
ARMv8.7-A
|
64 |
TBA | Application
|
||
ARMv8.8-A
|
64
|
TBA | Application
|
||
ARMv8.9-A
|
64
|
TBA | Application
|
||
ARMv9.0-A
|
64
|
ARM Cortex-A510, ARM Cortex-A710, ARM Cortex-A715, ARM Cortex-X2, ARM Cortex-X3, ARM Neoverse E2, ARM Neoverse N2, ARM Neoverse V2 | Application
|
||
ARMv9.1-A
|
64
|
TBA | Application
|
||
ARMv9.2-A
|
64
|
ARM Cortex-A520, ARM Cortex-A720, ARM Cortex-X4 | Apple M4[96] | Application
|
|
ARMv9.3-A
|
64
|
TBA | Application
|
||
ARMv9.4-A
|
64
|
TBA | Application
|
- ^ Jump up to: a b Although most datapaths and CPU registers in the early ARM processors were 32-bit, addressable memory was limited to 26 bits; with upper bits, then, used for status flags in the program counter register.
- ^ Jump up to: a b c ARMv3 included a compatibility mode to support the 26-bit addresses of earlier versions of the architecture. This compatibility mode optional in ARMv4, and removed entirely in ARMv5.
Arm provides a list of vendors who implement ARM cores in their design (application specific standard products (ASSP), microprocessor and microcontrollers).[99]
Example applications of ARM cores
[edit]
ARM cores are used in a number of products, particularly PDAs and smartphones. Some computing examples are Microsoft's first generation Surface, Surface 2 and Pocket PC devices (following 2002), Apple's iPads, and Asus's Eee Pad Transformer tablet computers, and several Chromebook laptops. Others include Apple's iPhone smartphones and iPod portable media players, Canon PowerShot digital cameras, Nintendo Switch hybrid, the Wii security processor and 3DS handheld game consoles, and TomTom turn-by-turn navigation systems.
In 2005, Arm took part in the development of Manchester University's computer SpiNNaker, which used ARM cores to simulate the human brain.[100]
ARM chips are also used in Raspberry Pi, BeagleBoard, BeagleBone, PandaBoard, and other single-board computers, because they are very small, inexpensive, and consume very little power.
32-bit architecture
[edit]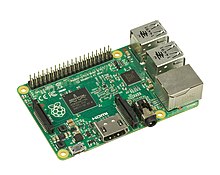

The 32-bit ARM architecture (ARM32), such as ARMv7-A (implementing AArch32; see section on Armv8-A for more on it), was the most widely used architecture in mobile devices as of 2011[update].[54]
Since 1995, various versions of the ARM Architecture Reference Manual (see § External links) have been the primary source of documentation on the ARM processor architecture and instruction set, distinguishing interfaces that all ARM processors are required to support (such as instruction semantics) from implementation details that may vary. The architecture has evolved over time, and version seven of the architecture, ARMv7, defines three architecture "profiles":
- A-profile, the "Application" profile, implemented by 32-bit cores in the Cortex-A series and by some non-ARM cores
- R-profile, the "Real-time" profile, implemented by cores in the Cortex-R series
- M-profile, the "Microcontroller" profile, implemented by most cores in the Cortex-M series
Although the architecture profiles were first defined for ARMv7, ARM subsequently defined the ARMv6-M architecture (used by the Cortex M0/M0+/M1) as a subset of the ARMv7-M profile with fewer instructions.
CPU modes
[edit]Except in the M-profile, the 32-bit ARM architecture specifies several CPU modes, depending on the implemented architecture features. At any moment in time, the CPU can be in only one mode, but it can switch modes due to external events (interrupts) or programmatically.[101]
- User mode: The only non-privileged mode.
- FIQ mode: A privileged mode that is entered whenever the processor accepts a fast interrupt request.
- IRQ mode: A privileged mode that is entered whenever the processor accepts an interrupt.
- Supervisor (svc) mode: A privileged mode entered whenever the CPU is reset or when an SVC instruction is executed.
- Abort mode: A privileged mode that is entered whenever a prefetch abort or data abort exception occurs.
- Undefined mode: A privileged mode that is entered whenever an undefined instruction exception occurs.
- System mode (ARMv4 and above): The only privileged mode that is not entered by an exception. It can only be entered by executing an instruction that explicitly writes to the mode bits of the Current Program Status Register (CPSR) from another privileged mode (not from user mode).
- Monitor mode (ARMv6 and ARMv7 Security Extensions, ARMv8 EL3): A monitor mode is introduced to support TrustZone extension in ARM cores.
- Hyp mode (ARMv7 Virtualization Extensions, ARMv8 EL2): A hypervisor mode that supports Popek and Goldberg virtualization requirements for the non-secure operation of the CPU.[102][103]
- Thread mode (ARMv6-M, ARMv7-M, ARMv8-M): A mode which can be specified as either privileged or unprivileged. Whether the Main Stack Pointer (MSP) or Process Stack Pointer (PSP) is used can also be specified in CONTROL register with privileged access. This mode is designed for user tasks in RTOS environment but it is typically used in bare-metal for super-loop.
- Handler mode (ARMv6-M, ARMv7-M, ARMv8-M): A mode dedicated for exception handling (except the RESET which are handled in Thread mode). Handler mode always uses MSP and works in privileged level.
Instruction set
[edit]The original (and subsequent) ARM implementation was hardwired without microcode, like the much simpler 8-bit 6502 processor used in prior Acorn microcomputers.
The 32-bit ARM architecture (and the 64-bit architecture for the most part) includes the following RISC features:
- Load–store architecture.
- No support for unaligned memory accesses in the original version of the architecture. ARMv6 and later, except some microcontroller versions, support unaligned accesses for half-word and single-word load/store instructions with some limitations, such as no guaranteed atomicity.[104][105]
- Uniform 16 × 32-bit register file (including the program counter, stack pointer and the link register).
- Fixed instruction width of 32 bits to ease decoding and pipelining, at the cost of decreased code density. Later, the Thumb instruction set added 16-bit instructions and increased code density.
- Mostly single clock-cycle execution.
To compensate for the simpler design, compared with processors like the Intel 80286 and Motorola 68020, some additional design features were used:
- Conditional execution of most instructions reduces branch overhead and compensates for the lack of a branch predictor in early chips.
- Arithmetic instructions alter condition codes only when desired.
- 32-bit barrel shifter can be used without performance penalty with most arithmetic instructions and address calculations.
- Has powerful indexed addressing modes.
- A link register supports fast leaf function calls.
- A simple, but fast, 2-priority-level interrupt subsystem has switched register banks.
Arithmetic instructions
[edit]ARM includes integer arithmetic operations for add, subtract, and multiply; some versions of the architecture also support divide operations.
ARM supports 32-bit × 32-bit multiplies with either a 32-bit result or 64-bit result, though Cortex-M0 / M0+ / M1 cores do not support 64-bit results.[106] Some ARM cores also support 16-bit × 16-bit and 32-bit × 16-bit multiplies.
The divide instructions are only included in the following ARM architectures:
- Armv7-M and Armv7E-M architectures always include divide instructions.[107]
- Armv7-R architecture always includes divide instructions in the Thumb instruction set, but optionally in its 32-bit instruction set.[108]
- Armv7-A architecture optionally includes the divide instructions. The instructions might not be implemented, or implemented only in the Thumb instruction set, or implemented in both the Thumb and ARM instruction sets, or implemented if the Virtualization Extensions are included.[108]
Registers
[edit]usr | sys | svc | abt | und | irq | fiq |
---|---|---|---|---|---|---|
R0 | ||||||
R1 | ||||||
R2 | ||||||
R3 | ||||||
R4 | ||||||
R5 | ||||||
R6 | ||||||
R7 | ||||||
R8 | R8_fiq | |||||
R9 | R9_fiq | |||||
R10 | R10_fiq | |||||
R11 | R11_fiq | |||||
R12 | R12_fiq | |||||
R13 | R13_svc | R13_abt | R13_und | R13_irq | R13_fiq | |
R14 | R14_svc | R14_abt | R14_und | R14_irq | R14_fiq | |
R15 | ||||||
CPSR | ||||||
SPSR_svc | SPSR_abt | SPSR_und | SPSR_irq | SPSR_fiq |
Registers R0 through R7 are the same across all CPU modes; they are never banked.
Registers R8 through R12 are the same across all CPU modes except FIQ mode. FIQ mode has its own distinct R8 through R12 registers.
R13 and R14 are banked across all privileged CPU modes except system mode. That is, each mode that can be entered because of an exception has its own R13 and R14. These registers generally contain the stack pointer and the return address from function calls, respectively.
Aliases:
- R13 is also referred to as SP, the stack pointer.
- R14 is also referred to as LR, the link register.
- R15 is also referred to as PC, the program counter.
The Current Program Status Register (CPSR) has the following 32 bits.[109]
- M (bits 0–4) is the processor mode bits.
- T (bit 5) is the Thumb state bit.
- F (bit 6) is the FIQ disable bit.
- I (bit 7) is the IRQ disable bit.
- A (bit 8) is the imprecise data abort disable bit.
- E (bit 9) is the data endianness bit.
- IT (bits 10–15 and 25–26) is the if-then state bits.
- GE (bits 16–19) is the greater-than-or-equal-to bits.
- DNM (bits 20–23) is the do not modify bits.
- J (bit 24) is the Java state bit.
- Q (bit 27) is the sticky overflow bit.
- V (bit 28) is the overflow bit.
- C (bit 29) is the carry/borrow/extend bit.
- Z (bit 30) is the zero bit.
- N (bit 31) is the negative/less than bit.
Conditional execution
[edit]Almost every ARM instruction has a conditional execution feature called predication, which is implemented with a 4-bit condition code selector (the predicate). To allow for unconditional execution, one of the four-bit codes causes the instruction to be always executed. Most other CPU architectures only have condition codes on branch instructions.[110]
Though the predicate takes up four of the 32 bits in an instruction code, and thus cuts down significantly on the encoding bits available for displacements in memory access instructions, it avoids branch instructions when generating code for small if
statements. Apart from eliminating the branch instructions themselves, this preserves the fetch/decode/execute pipeline at the cost of only one cycle per skipped instruction.
An algorithm that provides a good example of conditional execution is the subtraction-based Euclidean algorithm for computing the greatest common divisor. In the C programming language, the algorithm can be written as:
int gcd(int a, int b) {
while (a != b) // We enter the loop when a < b or a > b, but not when a == b
if (a > b) // When a > b we do this
a -= b;
else // When a < b we do that (no "if (a < b)" needed since a != b is checked in while condition)
b -= a;
return a;
}
The same algorithm can be rewritten in a way closer to target ARM instructions as:
loop:
// Compare a and b
GT = a > b;
LT = a < b;
NE = a != b;
// Perform operations based on flag results
if (GT) a -= b; // Subtract *only* if greater-than
if (LT) b -= a; // Subtract *only* if less-than
if (NE) goto loop; // Loop *only* if compared values were not equal
return a;
and coded in assembly language as:
; assign a to register r0, b to r1
loop: CMP r0, r1 ; set condition "NE" if (a ≠ b),
; "GT" if (a > b),
; or "LT" if (a < b)
SUBGT r0, r0, r1 ; if "GT" (Greater Than), then a = a − b
SUBLT r1, r1, r0 ; if "LT" (Less Than), then b = b − a
BNE loop ; if "NE" (Not Equal), then loop
B lr ; return
which avoids the branches around the then
and else
clauses. If r0
and r1
are equal then neither of the SUB
instructions will be executed, eliminating the need for a conditional branch to implement the while
check at the top of the loop, for example had SUBLE
(less than or equal) been used.
One of the ways that Thumb code provides a more dense encoding is to remove the four-bit selector from non-branch instructions.
Other features
[edit]Another feature of the instruction set is the ability to fold shifts and rotates into the data processing (arithmetic, logical, and register-register move) instructions, so that, for example, the statement in C language:
a += (j << 2);
could be rendered as a one-word, one-cycle instruction:[111]
ADD Ra, Ra, Rj, LSL #2
This results in the typical ARM program being denser than expected with fewer memory accesses; thus the pipeline is used more efficiently.
The ARM processor also has features rarely seen in other RISC architectures, such as PC-relative addressing (indeed, on the 32-bit[1] ARM the PC is one of its 16 registers) and pre- and post-increment addressing modes.
The ARM instruction set has increased over time. Some early ARM processors (before ARM7TDMI), for example, have no instruction to store a two-byte quantity.
Pipelines and other implementation issues
[edit]The ARM7 and earlier implementations have a three-stage pipeline; the stages being fetch, decode, and execute. Higher-performance designs, such as the ARM9, have deeper pipelines: Cortex-A8 has thirteen stages. Additional implementation changes for higher performance include a faster adder and more extensive branch prediction logic. The difference between the ARM7DI and ARM7DMI cores, for example, was an improved multiplier; hence the added "M".
Coprocessors
[edit]The ARM architecture (pre-Armv8) provides a non-intrusive way of extending the instruction set using "coprocessors" that can be addressed using MCR, MRC, MRRC, MCRR, and similar instructions. The coprocessor space is divided logically into 16 coprocessors with numbers from 0 to 15, coprocessor 15 (cp15) being reserved for some typical control functions like managing the caches and MMU operation on processors that have one.
In ARM-based machines, peripheral devices are usually attached to the processor by mapping their physical registers into ARM memory space, into the coprocessor space, or by connecting to another device (a bus) that in turn attaches to the processor. Coprocessor accesses have lower latency, so some peripherals—for example, an XScale interrupt controller—are accessible in both ways: through memory and through coprocessors.
In other cases, chip designers only integrate hardware using the coprocessor mechanism. For example, an image processing engine might be a small ARM7TDMI core combined with a coprocessor that has specialised operations to support a specific set of HDTV transcoding primitives.
Debugging
[edit]This section needs additional citations for verification. (March 2011) |
All modern ARM processors include hardware debugging facilities, allowing software debuggers to perform operations such as halting, stepping, and breakpointing of code starting from reset. These facilities are built using JTAG support, though some newer cores optionally support ARM's own two-wire "SWD" protocol. In ARM7TDMI cores, the "D" represented JTAG debug support, and the "I" represented presence of an "EmbeddedICE" debug module. For ARM7 and ARM9 core generations, EmbeddedICE over JTAG was a de facto debug standard, though not architecturally guaranteed.
The ARMv7 architecture defines basic debug facilities at an architectural level. These include breakpoints, watchpoints and instruction execution in a "Debug Mode"; similar facilities were also available with EmbeddedICE. Both "halt mode" and "monitor" mode debugging are supported. The actual transport mechanism used to access the debug facilities is not architecturally specified, but implementations generally include JTAG support.
There is a separate ARM "CoreSight" debug architecture, which is not architecturally required by ARMv7 processors.
Debug Access Port
[edit]The Debug Access Port (DAP) is an implementation of an ARM Debug Interface.[112] There are two different supported implementations, the Serial Wire JTAG Debug Port (SWJ-DP) and the Serial Wire Debug Port (SW-DP).[113] CMSIS-DAP is a standard interface that describes how various debugging software on a host PC can communicate over USB to firmware running on a hardware debugger, which in turn talks over SWD or JTAG to a CoreSight-enabled ARM Cortex CPU.[114][115][116]
DSP enhancement instructions
[edit]To improve the ARM architecture for digital signal processing and multimedia applications, DSP instructions were added to the instruction set.[117] These are signified by an "E" in the name of the ARMv5TE and ARMv5TEJ architectures. E-variants also imply T, D, M, and I.
The new instructions are common in digital signal processor (DSP) architectures. They include variations on signed multiply–accumulate, saturated add and subtract, and count leading zeros.
First introduced in 1999, this extension of the core instruction set contrasted with ARM's earlier DSP coprocessor known as Piccolo, which employed a distinct, incompatible instruction set whose execution involved a separate program counter.[118] Piccolo instructions employed a distinct register file of sixteen 32-bit registers, with some instructions combining registers for use as 48-bit accumulators and other instructions addressing 16-bit half-registers. Some instructions were able to operate on two such 16-bit values in parallel. Communication with the Piccolo register file involved load to Piccolo and store from Piccolo coprocessor instructions via two buffers of eight 32-bit entries. Described as reminiscent of other approaches, notably Hitachi's SH-DSP and Motorola's 68356, Piccolo did not employ dedicated local memory and relied on the bandwidth of the ARM core for DSP operand retrieval, impacting concurrent performance.[119] Piccolo's distinct instruction set also proved not to be a "good compiler target".[118]
SIMD extensions for multimedia
[edit]Introduced in the ARMv6 architecture, this was a precursor to Advanced SIMD, also named Neon.[120]
Jazelle
[edit]Jazelle DBX (Direct Bytecode eXecution) is a technique that allows Java bytecode to be executed directly in the ARM architecture as a third execution state (and instruction set) alongside the existing ARM and Thumb-mode. Support for this state is signified by the "J" in the ARMv5TEJ architecture, and in ARM9EJ-S and ARM7EJ-S core names. Support for this state is required starting in ARMv6 (except for the ARMv7-M profile), though newer cores only include a trivial implementation that provides no hardware acceleration.
Thumb
[edit]To improve compiled code density, processors since the ARM7TDMI (released in 1994[121]) have featured the Thumb compressed instruction set, which have their own state. (The "T" in "TDMI" indicates the Thumb feature.) When in this state, the processor executes the Thumb instruction set, a compact 16-bit encoding for a subset of the ARM instruction set.[122] Most of the Thumb instructions are directly mapped to normal ARM instructions. The space saving comes from making some of the instruction operands implicit and limiting the number of possibilities compared to the ARM instructions executed in the ARM instruction set state.
In Thumb, the 16-bit opcodes have less functionality. For example, only branches can be conditional, and many opcodes are restricted to accessing only half of all of the CPU's general-purpose registers. The shorter opcodes give improved code density overall, even though some operations require extra instructions. In situations where the memory port or bus width is constrained to less than 32 bits, the shorter Thumb opcodes allow increased performance compared with 32-bit ARM code, as less program code may need to be loaded into the processor over the constrained memory bandwidth.
Unlike processor architectures with variable length (16- or 32-bit) instructions, such as the Cray-1 and Hitachi SuperH, the ARM and Thumb instruction sets exist independently of each other. Embedded hardware, such as the Game Boy Advance, typically have a small amount of RAM accessible with a full 32-bit datapath; the majority is accessed via a 16-bit or narrower secondary datapath. In this situation, it usually makes sense to compile Thumb code and hand-optimise a few of the most CPU-intensive sections using full 32-bit ARM instructions, placing these wider instructions into the 32-bit bus accessible memory.
The first processor with a Thumb instruction decoder was the ARM7TDMI. All processors supporting 32-bit instruction sets, starting with ARM9, and including XScale, have included a Thumb instruction decoder. It includes instructions adopted from the Hitachi SuperH (1992), which was licensed by ARM.[123] ARM's smallest processor families (Cortex M0 and M1) implement only the 16-bit Thumb instruction set for maximum performance in lowest cost applications. ARM processors that don't support 32-bit addressing also omit Thumb.
Thumb-2
[edit]Thumb-2 technology was introduced in the ARM1156 core, announced in 2003. Thumb-2 extends the limited 16-bit instruction set of Thumb with additional 32-bit instructions to give the instruction set more breadth, thus producing a variable-length instruction set. A stated aim for Thumb-2 was to achieve code density similar to Thumb with performance similar to the ARM instruction set on 32-bit memory.
Thumb-2 extends the Thumb instruction set with bit-field manipulation, table branches and conditional execution. At the same time, the ARM instruction set was extended to maintain equivalent functionality in both instruction sets. A new "Unified Assembly Language" (UAL) supports generation of either Thumb or ARM instructions from the same source code; versions of Thumb seen on ARMv7 processors are essentially as capable as ARM code (including the ability to write interrupt handlers). This requires a bit of care, and use of a new "IT" (if-then) instruction, which permits up to four successive instructions to execute based on a tested condition, or on its inverse. When compiling into ARM code, this is ignored, but when compiling into Thumb it generates an actual instruction. For example:
; if (r0 == r1)
CMP r0, r1
ITE EQ ; ARM: no code ... Thumb: IT instruction
; then r0 = r2;
MOVEQ r0, r2 ; ARM: conditional; Thumb: condition via ITE 'T' (then)
; else r0 = r3;
MOVNE r0, r3 ; ARM: conditional; Thumb: condition via ITE 'E' (else)
; recall that the Thumb MOV instruction has no bits to encode "EQ" or "NE".
All ARMv7 chips support the Thumb instruction set. All chips in the Cortex-A series that support ARMv7, all Cortex-R series, and all ARM11 series support both "ARM instruction set state" and "Thumb instruction set state", while chips in the Cortex-M series support only the Thumb instruction set.[124][125][126]
Thumb Execution Environment (ThumbEE)
[edit]ThumbEE (erroneously called Thumb-2EE in some ARM documentation), which was marketed as Jazelle RCT[127] (Runtime Compilation Target), was announced in 2005 and deprecated in 2011. It first appeared in the Cortex-A8 processor. ThumbEE is a fourth instruction set state, making small changes to the Thumb-2 extended instruction set. These changes make the instruction set particularly suited to code generated at runtime (e.g. by JIT compilation) in managed Execution Environments. ThumbEE is a target for languages such as Java, C#, Perl, and Python, and allows JIT compilers to output smaller compiled code without reducing performance.[citation needed]
New features provided by ThumbEE include automatic null pointer checks on every load and store instruction, an instruction to perform an array bounds check, and special instructions that call a handler. In addition, because it utilises Thumb-2 technology, ThumbEE provides access to registers r8–r15 (where the Jazelle/DBX Java VM state is held).[128] Handlers are small sections of frequently called code, commonly used to implement high level languages, such as allocating memory for a new object. These changes come from repurposing a handful of opcodes, and knowing the core is in the new ThumbEE state.
On 23 November 2011, Arm deprecated any use of the ThumbEE instruction set,[129] and Armv8 removes support for ThumbEE.
Floating-point (VFP)
[edit]VFP (Vector Floating Point) technology is a floating-point unit (FPU) coprocessor extension to the ARM architecture[130] (implemented differently in Armv8 – coprocessors not defined there). It provides low-cost single-precision and double-precision floating-point computation fully compliant with the ANSI/IEEE Std 754-1985 Standard for Binary Floating-Point Arithmetic. VFP provides floating-point computation suitable for a wide spectrum of applications such as PDAs, smartphones, voice compression and decompression, three-dimensional graphics and digital audio, printers, set-top boxes, and automotive applications. The VFP architecture was intended to support execution of short "vector mode" instructions but these operated on each vector element sequentially and thus did not offer the performance of true single instruction, multiple data (SIMD) vector parallelism. This vector mode was therefore removed shortly after its introduction,[131] to be replaced with the much more powerful Advanced SIMD, also named Neon.
Some devices such as the ARM Cortex-A8 have a cut-down VFPLite module instead of a full VFP module, and require roughly ten times more clock cycles per float operation.[132] Pre-Armv8 architecture implemented floating-point/SIMD with the coprocessor interface. Other floating-point and/or SIMD units found in ARM-based processors using the coprocessor interface include FPA, FPE, iwMMXt, some of which were implemented in software by trapping but could have been implemented in hardware. They provide some of the same functionality as VFP but are not opcode-compatible with it. FPA10 also provides extended precision, but implements correct rounding (required by IEEE 754) only in single precision.[133]
- VFPv1
- Obsolete
- VFPv2
- An optional extension to the ARM instruction set in the ARMv5TE, ARMv5TEJ and ARMv6 architectures. VFPv2 has 16 64-bit FPU registers.
- VFPv3 or VFPv3-D32
- Implemented on most Cortex-A8 and A9 ARMv7 processors. It is backward-compatible with VFPv2, except that it cannot trap floating-point exceptions. VFPv3 has 32 64-bit FPU registers as standard, adds VCVT instructions to convert between scalar, float and double, adds immediate mode to VMOV such that constants can be loaded into FPU registers.
- VFPv3-D16
- As above, but with only 16 64-bit FPU registers. Implemented on Cortex-R4 and R5 processors and the Tegra 2 (Cortex-A9).
- VFPv3-F16
- Uncommon; it supports IEEE754-2008 half-precision (16-bit) floating point as a storage format.
- VFPv4 or VFPv4-D32
- Implemented on Cortex-A12 and A15 ARMv7 processors, Cortex-A7 optionally has VFPv4-D32 in the case of an FPU with Neon.[134] VFPv4 has 32 64-bit FPU registers as standard, adds both half-precision support as a storage format and fused multiply-accumulate instructions to the features of VFPv3.
- VFPv4-D16
- As above, but it has only 16 64-bit FPU registers. Implemented on Cortex-A5 and A7 processors in the case of an FPU without Neon.[134]
- VFPv5-D16-M
- Implemented on Cortex-M7 when single and double-precision floating-point core option exists.
In Debian Linux and derivatives such as Ubuntu and Linux Mint, armhf (ARM hard float) refers to the ARMv7 architecture including the additional VFP3-D16 floating-point hardware extension (and Thumb-2) above. Software packages and cross-compiler tools use the armhf vs. arm/armel suffixes to differentiate.[135]
Advanced SIMD (Neon)
[edit]The Advanced SIMD extension (also known as Neon or "MPE" Media Processing Engine) is a combined 64- and 128-bit SIMD instruction set that provides standardised acceleration for media and signal processing applications. Neon is included in all Cortex-A8 devices, but is optional in Cortex-A9 devices.[136] Neon can execute MP3 audio decoding on CPUs running at 10 MHz, and can run the GSM adaptive multi-rate (AMR) speech codec at 13 MHz. It features a comprehensive instruction set, separate register files, and independent execution hardware.[137] Neon supports 8-, 16-, 32-, and 64-bit integer and single-precision (32-bit) floating-point data and SIMD operations for handling audio and video processing as well as graphics and gaming processing. In Neon, the SIMD supports up to 16 operations at the same time. The Neon hardware shares the same floating-point registers as used in VFP. Devices such as the ARM Cortex-A8 and Cortex-A9 support 128-bit vectors, but will execute with 64 bits at a time,[132] whereas newer Cortex-A15 devices can execute 128 bits at a time.[138][139]
A quirk of Neon in Armv7 devices is that it flushes all subnormal numbers to zero, and as a result the GCC compiler will not use it unless -funsafe-math-optimizations
, which allows losing denormals, is turned on. "Enhanced" Neon defined since Armv8 does not have this quirk, but as of GCC 8.2 the same flag is still required to enable Neon instructions.[140] On the other hand, GCC does consider Neon safe on AArch64 for Armv8.
ProjectNe10 is ARM's first open-source project (from its inception; while they acquired an older project, now named Mbed TLS). The Ne10 library is a set of common, useful functions written in both Neon and C (for compatibility). The library was created to allow developers to use Neon optimisations without learning Neon, but it also serves as a set of highly optimised Neon intrinsic and assembly code examples for common DSP, arithmetic, and image processing routines. The source code is available on GitHub.[141]
ARM Helium technology
[edit]Helium is the M-Profile Vector Extension (MVE). It adds more than 150 scalar and vector instructions.[142]
Security extensions
[edit]TrustZone (for Cortex-A profile)
[edit]The Security Extensions, marketed as TrustZone Technology, is in ARMv6KZ and later application profile architectures. It provides a low-cost alternative to adding another dedicated security core to an SoC, by providing two virtual processors backed by hardware based access control. This lets the application core switch between two states, referred to as worlds (to reduce confusion with other names for capability domains), to prevent information leaking from the more trusted world to the less trusted world. This world switch is generally orthogonal to all other capabilities of the processor, thus each world can operate independently of the other while using the same core. Memory and peripherals are then made aware of the operating world of the core and may use this to provide access control to secrets and code on the device.[143]
Typically, a rich operating system is run in the less trusted world, with smaller security-specialised code in the more trusted world, aiming to reduce the attack surface. Typical applications include DRM functionality for controlling the use of media on ARM-based devices,[144] and preventing any unapproved use of the device.
In practice, since the specific implementation details of proprietary TrustZone implementations have not been publicly disclosed for review, it is unclear what level of assurance is provided for a given threat model, but they are not immune from attack.[145][146]
Open Virtualization[147] is an open source implementation of the trusted world architecture for TrustZone.
AMD has licensed and incorporated TrustZone technology into its Secure Processor Technology.[148] Enabled in some but not all products, AMD's APUs include a Cortex-A5 processor for handling secure processing.[149][150][151] In fact, the Cortex-A5 TrustZone core had been included in earlier AMD products, but was not enabled due to time constraints.[150]
Samsung Knox uses TrustZone for purposes such as detecting modifications to the kernel, storing certificates and attestating keys.[152]
TrustZone for Armv8-M (for Cortex-M profile)
[edit]The Security Extension, marketed as TrustZone for Armv8-M Technology, was introduced in the Armv8-M architecture. While containing similar concepts to TrustZone for Armv8-A, it has a different architectural design, as world switching is performed using branch instructions instead of using exceptions. It also supports safe interleaved interrupt handling from either world regardless of the current security state. Together these features provide low latency calls to the secure world and responsive interrupt handling. ARM provides a reference stack of secure world code in the form of Trusted Firmware for M and PSA Certified.
No-execute page protection
[edit]As of ARMv6, the ARM architecture supports no-execute page protection, which is referred to as XN, for eXecute Never.[153]
Large Physical Address Extension (LPAE)
[edit]The Large Physical Address Extension (LPAE), which extends the physical address size from 32 bits to 40 bits, was added to the Armv7-A architecture in 2011.[154]
The physical address size may be even larger in processors based on the 64-bit (Armv8-A) architecture. For example, it is 44 bits in Cortex-A75 and Cortex-A65AE.[155]
Армв8-Р и Армв8-М
[ редактировать ]Архитектуры Armv8-R и Armv8-M , анонсированные после архитектуры Armv8-A, имеют некоторые общие функции с Armv8-A. Однако Armv8-M не включает никаких 64-битных инструкций AArch64, а Armv8-R изначально не включал никаких инструкций AArch64; эти инструкции были добавлены в Armv8-R позже.
Армв8.1-М
[ редактировать ]Архитектура Armv8.1-M, анонсированная в феврале 2019 года, представляет собой усовершенствование архитектуры Armv8-M. Он приносит новые функции, в том числе:
- Новое расширение набора векторных команд. Векторное расширение M-профиля (MVE), или Helium, предназначено для приложений обработки сигналов и машинного обучения.
- Дополнительные улучшения набора команд для циклов и ветвей (расширение ветвей с низкими издержками).
- Инструкции для поддержки чисел с плавающей запятой половинной точности .
- Улучшен набор инструкций для управления TrustZone для модулей с плавающей запятой (FPU).
- Новый атрибут памяти в модуле защиты памяти (MPU).
- Улучшения в отладке, включая блок мониторинга производительности (PMU), непривилегированное расширение отладки и дополнительную поддержку отладки, ориентированы на разработку приложений для обработки сигналов.
- Расширение надежности, доступности и удобства обслуживания (RAS).
64/32-битная архитектура
[ редактировать ]
Armv8
[ редактировать ]Армв8-А
[ редактировать ]Объявлено в октябре 2011 г. [14] Armv8-A (часто называемый ARMv8, хотя также доступен Armv8-R) представляет собой фундаментальное изменение в архитектуре ARM. Он поддерживает два состояния выполнения : 64-битное состояние с именем AArch64 и 32-битное состояние с именем AArch32 . В состоянии AArch64 A64 поддерживается новый 64-битный набор инструкций ; в состоянии AArch32 поддерживаются два набора инструкций: исходный 32-битный набор команд с именем A32 и 32-битный набор инструкций Thumb-2 с именем T32 . AArch32 обеспечивает совместимость пользовательского пространства с Armv7-A. Состояние процессора может измениться при изменении уровня исключения; это позволяет 32-битным приложениям выполняться в состоянии AArch32 в 64-битной ОС, ядро которой выполняется в состоянии AArch64, а также позволяет 32-битной ОС работать в состоянии AArch32 под управлением 64-битного гипервизора, работающего в состоянии AArch64. . [1] ARM анонсировала свои ядра Cortex-A53 и Cortex-A57 30 октября 2012 года. [74] Apple была первой, кто выпустил ядро, совместимое с Armv8-A, в потребительском продукте ( Apple A7 в iPhone 5S ). AppliedMicro , используя FPGA , первым продемонстрировал Armv8-A. [156] Первой SoC Armv8-A от Samsung является Exynos 5433, используемый в Galaxy Note 4 , который имеет два кластера по четыре ядра Cortex-A57 и Cortex-A53 в конфигурации big.LITTLE ; но он будет работать только в режиме AArch32. [157]
Как для AArch32, так и для AArch64 Armv8-A поддерживает стандарт VFPv3/v4 и расширенный SIMD (Neon). Он также добавляет инструкции криптографии, поддерживающие AES , SHA-1 / SHA-256 и арифметику с конечными полями . [158] AArch64 был представлен в Armv8-A и его последующей версии. AArch64 не включен в 32-битные архитектуры Armv8-R и Armv8-M.
Процессор ARMv8-A может поддерживать один или оба AArch32 и AArch64; он может поддерживать AArch32 и AArch64 на более низких уровнях исключений и только AArch64 на более высоких уровнях исключений. [159] Например, ARM Cortex-A32 поддерживает только AArch32. [160] ARM Cortex-A34 поддерживает только AArch64, [161] а ARM Cortex-A72 поддерживает как AArch64, так и AArch32. [162] Процессор ARMv9-A должен поддерживать AArch64 на всех уровнях исключений и может поддерживать AArch32 на уровне EL0. [159]
Армв8-Р
[ редактировать ]В профиль Armv8-R была добавлена дополнительная поддержка AArch64, причем первым ядром ARM, реализующим ее, стал Cortex-R82. [163] Он добавляет набор инструкций A64.
Армв9
[ редактировать ]Армв9-А
[ редактировать ]В обновленной архитектуре, анонсированной в марте 2021 года, особое внимание уделяется безопасному выполнению и разделениям . [164] [165]
Система вооружения готова
[ редактировать ]Arm SystemReady, ранее называвшаяся Arm ServerReady, — это программа сертификации, которая помогает внедрить стандартные готовые операционные системы и гипервизоры в системы на базе Arm, от серверов центров обработки данных до промышленных периферийных устройств и устройств Интернета вещей. Ключевыми строительными блоками программы являются спецификации минимальных требований к оборудованию и встроенному ПО, на которые могут опираться операционные системы и гипервизоры. Эти характеристики: [166]
- Базовая системная архитектура (BSA) [167] и дополнения для конкретного сегмента рынка (например, дополнение Server BSA) [168]
- Базовые требования к загрузке (BBR) [169] и базовые требования безопасности загрузки (BBR) [170]
Эти спецификации разработаны совместно компанией Arm и ее партнерами из Консультативного комитета по системной архитектуре (SystemArchAC).
Architecture Compliance Suite (ACS) — это инструменты тестирования, которые помогают проверить соответствие этим спецификациям. Спецификация требований Arm SystemReady документирует требования сертификации. [171]
представила Эту программу компания Arm в 2020 году на первом мероприятии DevSummit . Его предшественник Arm ServerReady был представлен в 2018 году на мероприятии Arm TechCon. В настоящее время в эту программу входят четыре группы:
- SystemReady SR: этот диапазон предназначен для серверов и рабочих станций, поддерживающих операционные системы и гипервизоры, требующие интерфейсов UEFI , ACPI и SMBIOS . Windows, Red Hat Enterprise Linux и VMware ESXi-Arm требуют этих интерфейсов, хотя другие дистрибутивы Linux и BSD также могут их поддерживать. [ нужны разъяснения ]
- SystemReady LS (LinuxBoot System): этот диапазон предназначен для серверов, которые гиперскейлеры используют для поддержки операционных систем Linux, которым требуется прошивка LinuxBoot вместе с интерфейсами ACPI и SMBIOS.
- SystemReady ES (встроенная система): этот диапазон предназначен для промышленных периферийных устройств и устройств Интернета вещей, которые поддерживают операционные системы и гипервизоры, требующие интерфейсов UEFI, ACPI и SMBIOS. Windows IoT Enterprise, Red Hat Enterprise Linux и VMware ESXi-Arm требуют этих интерфейсов, хотя другие дистрибутивы Linux и BSD также могут их поддерживать. [ нужны разъяснения ]
- SystemReady IR (IoT Ready): эта полоса предназначена для промышленных периферийных устройств и устройств IoT, которые поддерживают операционные системы, требующие интерфейсы UEFI и дерева устройств . Также могут поддерживаться встроенный Linux (например, Yocto ) и некоторые дистрибутивы Linux/BSD (например, Fedora, Ubuntu, Debian и OpenSUSE). [ нужны разъяснения ]
Сертифицированный PSA
[ редактировать ]Сертифицированный PSA , ранее называвшийся Архитектурой безопасности платформы, представляет собой независимую от архитектуры структуру безопасности и схему оценки. Он предназначен для обеспечения безопасности устройств Интернета вещей (IoT), построенных на процессорах «система на кристалле» (SoC). [172] Он был введен для повышения безопасности в тех случаях, когда полностью доверенная среда выполнения слишком велика или сложна. [173]
Архитектура была представлена компанией Arm в 2017 году на ежегодном мероприятии TechCon . [173] [174] Хотя схема не зависит от архитектуры, она впервые была реализована на процессорных ядрах Arm Cortex-M, предназначенных для использования в микроконтроллерах. Сертификат PSA включает в себя свободно доступные модели угроз и анализы безопасности, демонстрирующие процесс принятия решения о функциях безопасности в распространенных продуктах Интернета вещей. [175] Он также предоставляет бесплатно загружаемые пакеты интерфейса прикладного программирования (API), архитектурные спецификации, реализации встроенного ПО с открытым исходным кодом и соответствующие наборы тестов. [176]
После разработки структуры безопасности архитектуры в 2017 году схема обеспечения сертификации PSA была запущена два года спустя в Embedded World в 2019 году. [177] PSA Certified предлагает многоуровневую схему оценки безопасности для поставщиков чипов, поставщиков ОС и производителей устройств Интернета вещей. [178] Презентация Embedded World познакомила поставщиков чипов с сертификацией уровня 1. Тогда же был представлен проект защиты второго уровня. [179] Сертификация уровня 2 стала применимым стандартом в феврале 2020 года. [180]
Сертификация была создана совместными заинтересованными сторонами PSA, чтобы обеспечить индивидуальный подход к безопасности для разнообразного набора продуктов Интернета вещей. Спецификации, сертифицированные PSA, не зависят от реализации и архитектуры, поэтому их можно применять к любому чипу, программному обеспечению или устройству. [181] [179] Сертификация также устраняет фрагментацию отрасли среди производителей и разработчиков продуктов Интернета вещей . [182]
Поддержка операционной системы
[ редактировать ]32-битные операционные системы
[ редактировать ]Исторические операционные системы
[ редактировать ]Первый 32-битный персональный компьютер на базе ARM, Acorn Archimedes , изначально предназначался для работы под управлением амбициозной операционной системы под названием ARX . Машины поставлялись с ОС RISC , которая также использовалась в более поздних системах на базе ARM от Acorn и других производителей. Некоторые ранние машины Acorn также могли использовать порт Unix под названием RISC iX . (Не следует путать с RISC/os , современным вариантом Unix архитектуры MIPS.)
Встроенные операционные системы
[ редактировать ]32-битная архитектура ARM поддерживается большим количеством встроенных операционных систем и операционных систем реального времени , в том числе:
- А2
- Андроид
- ЧибиОС/РТ
- боги
- ДРИС
- ЭКоС
- embOS
- FreeBSD
- FreeRTOS
- ЧЕСТНОСТЬ
- Линукс
- Операционные системы микроконтроллеров
- Мбед
- МИНИКС 3
- MQX
- Нуклеус ПЛЮС
- NutX
- ОКЛ4
- Встроенная операционная система (OSE)
- ОС-9 [183]
- Фарос [184]
- План 9
- PikeOS [185]
- QNX
- БУНТ
- РТЭМС
- RTXC-кадры
- СКИПТА [186]
- ThreadX
- ТизенРТ
- Т-Ядро
- Вксворкс
- Windows встроенный компактный
- Windows 10 IoT ядро
- Зефир
Операционные системы мобильных устройств
[ редактировать ]По состоянию на март 2024 года 32-битная архитектура ARM была основной аппаратной средой для большинства операционных систем мобильных устройств, таких как следующие, но многие из этих платформ, таких как Android и Apple iOS, перешли на 64-битную архитектуру ARM:
Раньше, но сейчас снято с производства:
- Если есть
- ОС BlackBerry / BlackBerry 10
- ОС Firefox
- МиГо
- Ньютон ОС
- iOS 10 и более ранние версии
- Симбиан
- Windows 10 Мобильная
- Windows РТ
- Windows Телефон
- Windows Мобайл
Настольные и серверные операционные системы
[ редактировать ]32-битная архитектура ARM поддерживается ОС RISC и несколькими Unix-подобными операционными системами, включая:
- FreeBSD
- NetBSD
- OpenBSD
- OpenSolaris [187]
- несколько дистрибутивов Linux , например:
64-битные операционные системы
[ редактировать ]Встроенные операционные системы
[ редактировать ]- ЧЕСТНОСТЬ [188]
- ИЛИ [189]
- СКИПТА [186]
- продатьL4 [190]
- Фарос [184]
- FreeRTOS
- QNX [191]
- Вксворкс [192]
- Зефир
Операционные системы мобильных устройств
[ редактировать ]- Android поддерживает Armv8-A в Android Lollipop (5.0) и более поздних версиях.
- iOS поддерживает Armv8-A в iOS 7 и более поздних версиях на 64-битных процессорах Apple SoC . iOS 11 и более поздних версий, а также iPadOS поддерживают только 64-разрядные процессоры и приложения ARM.
- Мобиан
- ПостмаркетОС
- Арка Linux ARM
- Манджаро [193]
Настольные и серверные операционные системы
[ редактировать ]- Поддержка Armv8-A была включена в ядро Linux версии 3.7 в конце 2012 года. [194] Armv8-A поддерживается рядом дистрибутивов Linux , таких как:
- Поддержка Armv8-A была включена во FreeBSD в конце 2014 года. [202]
- OpenBSD имеет поддержку Armv8 с 2023 года. [update]. [203]
- NetBSD поддерживает Armv8 с начала 2018 года. [204]
- Windows — Windows 10 запускает 32-разрядные « x86- и 32-разрядные приложения ARM». [205] а также собственные настольные приложения ARM64; [206] [207] Windows 11 запускает собственные приложения ARM64, а также может запускать приложения x86 и x86-64 посредством эмуляции. Поддержка 64-битных приложений ARM в Microsoft Store доступна с ноября 2018 года. [208]
- macOS поддерживает ARM с конца 2020 года; Первый выпуск с поддержкой ARM — macOS Big Sur . [209] Rosetta 2 добавляет поддержку приложений x86-64 , но не виртуализацию компьютерных платформ x86-64. [210]
Портирование на 32- или 64-битные операционные системы ARM.
[ редактировать ]Приложения Windows, перекомпилированные для ARM и связанные с Winelib из проекта Wine , могут работать на 32-битной или 64-битной ARM в Linux, FreeBSD или других совместимых операционных системах. [211] [212] Двоичные файлы x86, например, если они не были специально скомпилированы для ARM, были продемонстрированы на ARM с использованием QEMU с Wine (в Linux и других системах), [ нужна ссылка ] но не работает на полной скорости или с теми же возможностями, что и Winelib.
Примечания
[ редактировать ]- ^ При использовании 32-битных слов скорость 4 Мбит/с соответствует 1 MIPS .
- ^ В доступных ссылках не упоминается, какая это была группа разработчиков, но, учитывая время и известную историю проектов той эпохи, вполне вероятно, что это была команда National Semiconductor, чей NS32016 страдал от большого количества ошибок.
- ^ Мэтт Эванс отмечает, что, похоже, более быстрые версии были просто отнесены к более высоким уровням и, похоже, не имели никаких основных изменений. [36]
См. также
[ редактировать ]- Янтарный — ядро процессора с открытым исходным кодом, совместимое с ARM.
- AMULET – асинхронная реализация архитектуры ARM.
- Яблочный кремний
- Аккредитованный инженер ARM – программа сертификации
- ARM big.LITTLE — гетерогенная вычислительная архитектура ARM.
- ARMulator - симулятор набора команд.
- Сравнение процессоров ARM
- Крах (уязвимость безопасности) [213]
- Компьютер с сокращенным набором команд (RISC)
- РИСК-V
- Призрак (уязвимость безопасности)
- Unicore — 32-регистровая архитектура, основанная на 32-битном ARM.
Ссылки
[ редактировать ]Цитаты
[ редактировать ]- ^ Перейти обратно: а б с д и ж Гризентуэйт, Ричард (2011). «Обзор технологии ARMv8-A» (PDF) . Архивировано из оригинала (PDF) 11 ноября 2011 года . Проверено 31 октября 2011 г.
- ^ «Стандарт вызова процедур для архитектуры ARM» (PDF) . Арм Холдингс . 30 ноября 2013 года . Проверено 27 мая 2013 г.
- ^ Перейти обратно: а б Уилсон, Роджер (2 ноября 1988 г.). «Некоторые факты о машине Acorn RISC» . Группа новостей : comp.arch . Проверено 25 мая 2007 г.
- ^ Перейти обратно: а б Хачман, Марк (14 октября 2002 г.). «Ядра ARM проникают на территорию 3G» . ЭкстримТех . Проверено 24 мая 2018 г.
- ^ Терли, Джим (18 декабря 2002 г.). «Двухпроцентное решение» . Встроенный . Проверено 14 февраля 2023 г.
- ^ Катресс, Ян (22 июня 2020 г.). «Новый суперкомпьютер №1: Fujitsu Fugaku и A64FX выводят Arm на вершину с производительностью 415 петафлопс» . anandtech.com . Проверено 25 января 2021 г.
- ^ «Arm Partners поставили 200 миллиардов чипов» . Оружие (Пресс-релиз) . Проверено 3 ноября 2021 г.
- ^ «Создание умного мира и использование искусственного интеллекта: ARM» . Обзор кремния . 2019 . Проверено 8 апреля 2020 г.
- ^ «Возможность массового подключения к Интернету вещей, поскольку партнеры ARM поставляют 100 миллиардов чипов» . сообщество.arm.com . 27 февраля 2017 г. Проверено 8 апреля 2020 г.
совокупное внедрение 100 миллиардов чипов, половина из которых была поставлена за последние четыре года. [..] почему не триллион или больше? Это наша цель: в течение следующих двух десятилетий будет развернуто триллион подключенных устройств.
- ^ «Рынок MCU на пути перехода к 32-битным устройствам и устройствам на базе ARM: 32-битные вершины продаж; 16-битные лидеры по поставкам единиц» . IC-инсайты. 25 апреля 2013 года . Проверено 1 июля 2014 г.
- ^ Терли, Джим (2002). «Двухпроцентное решение» . Embedded.com.
- ^ «Arm Holdings стремится к расширению ПК и серверов» . Регистр . 1 февраля 2011 г.
- ^ Макгуайр-Баланза, Керри (11 мая 2010 г.). «ARM от нуля до миллиардов за 25 коротких лет» . Арм Холдингс . Проверено 8 ноября 2012 г.
- ^ Перейти обратно: а б «ARM раскрывает технические подробности следующей версии архитектуры ARM» (пресс-релиз). Арм Холдингс . 27 октября 2011 г. Архивировано из оригинала 1 января 2019 г. . Проверено 20 сентября 2013 г.
- ^ «Анонс платформы ARM Neoverse N1» . сообщество.arm.com . 20 февраля 2019 г. Проверено 8 апреля 2020 г.
- ^ Фэйрберн, Дуглас (31 января 2012 г.). «Устная история Софи Уилсон» (PDF) . Архивировано (PDF) из оригинала 3 марта 2016 года . Проверено 2 февраля 2016 г.
- ^ Смит, Тони (30 ноября 2011 г.). «Би-би-си Micro исполняется 30 лет» . Регистровое оборудование . Архивировано из оригинала 12 декабря 2011 года . Проверено 12 декабря 2011 г.
- ^ Полссон, Кен. «Хронология микропроцессоров» . Processortimeline.info . Архивировано из оригинала 9 августа 2018 года . Проверено 27 сентября 2013 г.
- ^ Лиди, Гленн (апрель 1983 г.). «Семейство микропроцессоров National Semiconductor NS16000» . Байт . стр. 53–66 . Проверено 22 августа 2020 г.
- ^ Эванс 2019 , 6:00.
- ^ Маннерс, Дэвид (29 апреля 1998 г.). «Путь ARM» . Еженедельник электроники . Архивировано из оригинала 29 июля 2012 года . Проверено 26 октября 2012 г.
- ^ Эванс 2019 , 5:30.
- ^ Перейти обратно: а б Эванс 2019 , 7:45.
- ^ Эванс 2019 , 8:30.
- ^ Софи Уилсон на Alt Party 2009 (Часть 3/8) . Архивировано из оригинала 11 декабря 2021 года.
- ^ Чисналл, Дэвид (23 августа 2010 г.). Понимание архитектуры ARM . Проверено 26 мая 2013 г.
- ^ Эванс 2019 , 9:00.
- ^ Фербер, Стивен Б. (2000). Архитектура системы на кристалле ARM . Бостон: Аддисон-Уэсли. ISBN 0-201-67519-6 .
- ^ Эванс 2019 , 9:50.
- ^ Эванс 2019 , 23:30.
- ^ Эванс 2019 , 26:00.
- ^ «История разработки набора инструкций ARM с Софи Уилсон (Часть 3)» . 10 мая 2015 г. Архивировано из оригинала 11 декабря 2021 г. Проверено 25 мая 2020 г. - через YouTube.
- ^ «Устная история Софи Уилсон - сотрудник Музея компьютерной истории, 2012 г.» (PDF) . Музей истории компьютеров . 31 января 2012 года . Проверено 25 мая 2020 г.
- ^ Харкер, Т. (лето 2009 г.). «ARM серьезно относится к IP (вторая часть серии, состоящей из двух частей [Мнение ассоциированной редакции]») . IEEE Solid-State Circuits Magazine . 1 (3): 8–69. doi : 10.1109/MSSC.2009.933674 . ISSN 1943-0590. .S2CID 36567166 .
- ^ Эванс 2019 , 20:30.
- ^ Эванс 2019 , 22:00.
- ^ Эванс 2019 , 21:30.
- ^ Эванс 2019 , 22:0030.
- ^ Перейти обратно: а б Эванс 2019 , 14:00.
- ^ «От одного Arm к другому! Процессоры и архитектуры ARM» . Проверено 31 мая 2022 г.
- ^ Леви, Маркус. «История архитектуры ARM: от создания до IPO» (PDF) . Проверено 18 июля 2022 г.
- ^ Представляем Commodore Amiga 3000 (PDF) . Коммодор-Амига, Инк. 1991.
- ^ «Заявления о скорости компьютеров MIPS и MFLOPS с 1980 по 1996 год» . www.roylongbottom.org.uk . Проверено 17 июня 2023 г.
- ^ Сантану Чаттопадхьяй (2010). Проектирование встроенной системы . PHI Learning Pvt. ООО с. 9. ISBN 978-81-203-4024-4 .
- ^ Ричард Мюррей. «32-битная операция» .
- ^ «Вехи компании АРМ» . РУКА . Архивировано из оригинала 20 апреля 2015 года . Проверено 8 апреля 2015 г.
- ^ Эндрюс, Джейсон (2005). «3 темы проверки SoC для архитектуры ARM». Совместная проверка аппаратного и программного обеспечения для проектирования ARM SoC . Оксфорд, Великобритания: Elsevier . стр. 69 . ISBN 0-7506-7730-9 .
ARM начинала свою деятельность как филиал Acorn Computer в Кембридже, Англия, с созданием совместного предприятия между Acorn, Apple и VLSI Technology. Команда из двенадцати сотрудников разработала первый микропроцессор ARM в период с 1983 по 1985 год.
- ^ Вебер, Джонатан (28 ноября 1990 г.). «Apple присоединится к Acorn, VLSI в предприятии по производству микросхем» . Лос-Анджелес Таймс . Лос-Анджелес . Проверено 6 февраля 2012 года .
Apple инвестировала около 3 миллионов долларов (примерно 1,5 миллиона фунтов) в 30% акций компании, получившей название Advanced Risc Machines Ltd. (ARM) [...]
- ^ «Корпоративный справочник ARM» (PDF) . РУКА . Архивировано из оригинала (PDF) 4 октября 2006 года.
- ^ Монтанаро, Джеймс; и др. (1997). «КМОП RISC-микропроцессор 160 МГц, 32 бита, 0,5 Вт» (PDF) . Цифровой технический журнал . 9 (1): 49–62.
- ^ ДеМоун, Пол (9 ноября 2000 г.). «Гонка ARM за встраиваемое мировое господство» . Реальные мировые технологии . Проверено 6 октября 2015 г.
- ^ «Марш машин» . Technologyreview.com . Обзор технологий Массачусетского технологического института . 20 апреля 2010 г. Архивировано из оригинала 16 октября 2015 г. Проверено 6 октября 2015 г.
- ^ Кразит, Том (3 апреля 2006 г.). «вооружен для гостиной» . CNET.
- ^ Перейти обратно: а б Фитцпатрик, Дж. (2011). «Интервью со Стивом Фербером» . Коммуникации АКМ . 54 (5): 34–39. дои : 10.1145/1941487.1941501 .
- ^ Трейси Робинсон (12 февраля 2014 г.). «Празднование 50 миллиардов проданных чипов на базе ARM» .
- ^ Сара Марри (3 марта 2014 г.). «Охват ARM: рубеж в 50 миллиардов чипов» . Архивировано из оригинала 16 сентября 2015 года.
- ^ Браун, Эрик (2009). «Нетбук ARM поставляется со съемным планшетом» . Архивировано из оригинала 3 января 2013 года . Проверено 19 августа 2009 г.
- ^ Питер Кларк (7 января 2016 г.). «Amazon теперь продает собственные чипы ARM» .
- ^ «MACOM успешно завершила приобретение AppliedMicro» (пресс-релиз). 26 января 2017 г.
- ^ Фрумусану, Андрей. «Детали ARM, созданные на основе лицензии на технологию ARM Cortex» . АнандТех . Проверено 26 мая 2019 г.
- ^ Катресс, Ян. «Гибкий доступ ARM: спроектируйте SoC, прежде чем тратить деньги» . АнандТех . Проверено 9 октября 2019 г.
- ^ «Часто задаваемые вопросы о гибком доступе ARM» . РУКА . Проверено 9 октября 2019 г.
- ^ Нолтинг, Стефан. «Процессорная система STORM CORE» (PDF) . Открытые ядра . Проверено 1 апреля 2014 г.
- ^ ZAP на GitHub
- ^ «Процессор Кортекс-М23» . РУКА . Проверено 27 октября 2016 г.
- ^ «Процессор Кортекс-М33» . РУКА . Проверено 27 октября 2016 г.
- ^ «Архитектура ARMv8-M упрощает безопасность для встраиваемых систем Smart» . РУКА . Проверено 10 ноября 2015 г.
- ^ ООО, Арм. «Архитектура М-профиля» . Рука | Архитектура цифрового мира . Проверено 29 августа 2023 г.
- ^ «Архитектура ARMv8-R» . Проверено 10 июля 2015 г.
- ^ Краск, Саймон (октябрь 2013 г.). «Архитектура ARM Cortex-R» (PDF) . Арм Холдингс. Архивировано из оригинала (PDF) 6 апреля 2014 года . Проверено 1 февраля 2014 г.
- ^ Смит, Райан (20 сентября 2016 г.). «ARM анонсирует процессор Cortex-R52: детерминированный и безопасный для ADAS и многого другого» . АнандТех . Проверено 20 сентября 2016 г.
- ^ «Процессор Cortex-A32» . РУКА . Проверено 10 октября 2019 г.
- ^ «Процессор Cortex-A35» . РУКА . Проверено 10 ноября 2015 г.
- ^ Перейти обратно: а б «ARM выпускает серию Cortex-A50, самые энергоэффективные 64-битные процессоры в мире» (пресс-релиз). Арм Холдингс . Проверено 31 октября 2012 г.
- ^ «Процессор Cortex-A72» . РУКА . Проверено 10 июля 2015 г.
- ^ «Процессор Cortex-A73» . РУКА . Проверено 2 июня 2016 г.
- ^ «Архитектура ARMv8-A» . Проверено 10 июля 2015 г.
- ^ «Cavium Thunder X увеличивает количество ядер ARM на одном чипе до 48» . Полуточный . 3 июня 2014 г.
- ^ «Cavium на Supercomputing 2014» . Яху Финанс . 17 ноября 2014 года. Архивировано из оригинала 16 октября 2015 года . Проверено 15 января 2017 г.
- ^ Берт, Джефф (17 ноября 2014 г.). «Cray оценит чипы ARM в своих суперкомпьютерах» . электронная неделя .
- ^ «Samsung анонсирует Exynos 8890 с модемом Cat.12/13 и специальным процессором» . АнандТех .
- ^ «Процессор Cortex-A34» . РУКА . Проверено 10 октября 2019 г.
- ^ «D21500 [AARCH64] Добавить поддержку Broadcom Vulcan» . Reviews.llvm.org .
- ^ «Процессор Cortex-A55» . РУКА . Проверено 29 мая 2017 г.
- ^ «Процессор Cortex-A75» . РУКА . Проверено 29 мая 2017 г.
- ^ «Процессор Cortex-A76» . РУКА . Проверено 11 октября 2018 г.
- ^ Беренис Манн (апрель 2017 г.). «Архитектура ARM — эволюция и доставка ARMv8.2-A» . сообщество.ARM.com .
- ^ Фрумусану, Андрей. «Samsung анонсирует Exynos 9825 SoC: первый 7-нм кремниевый чип EUV» . АнандТех . Проверено 11 октября 2019 г.
- ^ «Fujitsu начала производить в Японии миллиарды супервычислений с помощью самого мощного ARM-процессора A64FX» . Новости информационных технологий Китая . Архивировано из оригинала 20 июня 2019 года . Проверено 17 августа 2019 г.
Чип ARMv8 SVE (масштабируемое векторное расширение), использующий 512-битную плавающую запятую.
- ^ «Кортекс-А65АЕ – АРМ» . РУКА . Проверено 8 апреля 2020 г.
может выполнять два потока параллельно в каждом цикле. Каждый поток может находиться на разных уровнях исключений и запускать разные операционные системы.
- ^ Фрумусану, Андрей. «Marvell анонсирует ThunderX3: 96 ядер и 384 потока, серверный процессор ARM третьего поколения» . АнандТех . Проверено 26 мая 2020 г.
- ^ «AArch64: добавить поддержку новых процессоров Apple · apple/llvm-project@677da09» . Гитхаб . Проверено 23 сентября 2022 г.
- ^ «Новые возможности архитектуры Armv8-A — Блог «Архитектуры и процессоры» — Блоги сообщества Arm — Сообщество Arm» . сообщество.arm.com . 21 сентября 2020 г. Проверено 28 декабря 2021 г.
- ^ «Решение Arm для будущих потребностей искусственного интеллекта, безопасности и специализированных вычислений — это v9» . Рука . Проверено 16 августа 2021 г.
- ^ «Первые процессоры Armv9 Cortex для потребительских вычислений» . сообщество.arm.com . 25 мая 2021 г. Проверено 16 августа 2021 г.
- ^ «В компилятор LLVM добавлена поддержка Apple M4, что подтверждает его возможности ISA» . www.phoronix.com . Проверено 15 июня 2024 г.
- ^ «Развитие архитектуры Arm A-Profile 2021 — Блог об архитектурах и процессорах — Блоги сообщества Arm — Сообщество Arm» . сообщество.arm.com . 8 сентября 2021 г. Проверено 25 сентября 2023 г.
- ^ «Развитие архитектуры Arm A-Profile 2022 — Блог об архитектурах и процессорах — Блоги сообщества Arm — Сообщество Arm» . сообщество.arm.com . 29 сентября 2022 г. Проверено 25 сентября 2023 г.
- ^ «Линейная карта» (PDF) . 2003 . Проверено 1 октября 2012 года .
- ^ Пэрриш, Кевин (14 июля 2011 г.). «Один миллион ядер ARM связан с моделированием мозга» . ЭЭ Таймс . Проверено 2 августа 2011 г.
- ^ «Режим процессора» . Арм Холдингс . Проверено 26 марта 2013 г.
- ^ «КВМ/АРМ» (PDF) . Проверено 14 февраля 2023 г.
- ^ Браш, Дэвид (август 2010 г.). Расширения архитектуры ARMv7-A . 22-й симпозиум IEEE Hot Chips 2010 (HCS). стр. 1–21. дои : 10.1109/HOTCHIPS.2010.7480070 . ISBN 978-1-4673-8875-7 . S2CID 46339775 .
- ^ «Как компилятор ARM поддерживает невыровненный доступ?» . 2011 . Проверено 5 октября 2013 г.
- ^ «Несогласованный доступ к данным» . Проверено 5 октября 2013 г.
- ^ «Техническое справочное руководство Cortex-M0 r0p0» (PDF) . Рука .
- ^ «Справочное руководство по архитектуре ARMv7-M» . Рука . Проверено 18 июля 2022 г.
- ^ Перейти обратно: а б «Справочное руководство по архитектуре ARMv7-A и ARMv7-R; Arm Holdings» . Arm.com . Проверено 19 января 2013 г.
- ^ «Информационный центр АРМ» . Проверено 10 июля 2015 г.
- ^ «Коды состояний 1: Флаги и коды состояний» . ARM-сообщество . 11 сентября 2013 года . Проверено 26 сентября 2019 г.
- ^ «9.1.2. Счет командных циклов» .
- ^ «Компоненты CoreSight: о порте доступа для отладки» .
- ^ «Cortex-M3: порт доступа к отладке (DAP)» .
- ^ Андерсон, Майк. «Опции отладки аппаратного обеспечения ARM» (PDF) .
- ^ «Руководство пользователя отладчика CMSIS-DAP» .
- ^ «ЦМСИС-ДАП» .
- ^ «Расширения набора команд ARM DSP» . Arm.com . Архивировано из оригинала 14 апреля 2009 года . Проверено 18 апреля 2009 г.
- ^ Перейти обратно: а б Кларк, Питер (3 мая 1999 г.). «EPF: ARC, ARM добавляют расширения DSP к своим ядрам RISC» . ЭЭ Таймс . Проверено 15 марта 2024 г.
- ^ Терли, Джим (18 ноября 1996 г.). «ARM настраивает Piccolo для повышения производительности DSP» (PDF) . Отчет микропроцессора . Проверено 15 марта 2024 г.
- ^ «ЦСП и СИМД» . Проверено 10 июля 2015 г.
- ^ «Техническое справочное руководство ARM7TDMI» (PDF) . п. ii.
- ^ Джаггар, Дэйв (1996). Справочное руководство по архитектуре ARM . Прентис Холл. стр. 6–1. ISBN 978-0-13-736299-8 .
- ^ Натан Уиллис (10 июня 2015 г.). «Воскресение архитектуры SuperH» . LWN.net .
- ^ «Архитектура набора команд процессора ARM» . ARM.com. Архивировано из оригинала 15 апреля 2009 года . Проверено 18 апреля 2009 г.
- ^ «ARM нацелена на UC, ASSP, SoC» . Linuxdevices.com. Архивировано из оригинала 9 декабря 2012 года . Проверено 18 апреля 2009 г.
- ^ «Информационный центр АРМ» . Infocenter.arm.com . Проверено 18 апреля 2009 г.
- ^ «Жазель» . ARM Ltd. Архивировано из оригинала 2 июня 2017 года.
- ^ Том Р. Халфхилл (2005). «ARM усиливает компиляторы Java: новые 16-битные инструкции Thumb-2EE экономят системную память» (PDF) . Архивировано из оригинала (PDF) 5 октября 2007 года.
- ^ Справочное руководство по архитектуре ARM, издания Armv7-A и Armv7-R, выпуск Cb, раздел A2.10, 25 июля 2012 г.
- ^ «Цепочка инструментов компилятора ARM с использованием ассемблера – сопроцессора VFP» . АРМ.com . Проверено 20 августа 2014 г.
- ^ «Директивы VFP и векторная запись» . АРМ.com . Проверено 21 ноября 2011 г.
- ^ Перейти обратно: а б «Различия между ARM Cortex-A8 и Cortex-A9» . Шервин Эмами . Проверено 21 ноября 2011 г.
- ^ «Технические данные FPA10» (PDF) . chrisacorns.computinghistory.org.uk . GEC Plessey Semiconductors. 11 июня 1993 года . Проверено 26 ноября 2020 г.
Что касается IEEE 754-1985, FPA обеспечивает соответствие арифметике с одинарной точностью [...] Иногда умножения с двойной и расширенной точностью могут производиться с ошибкой в 1 или 2 единицы в наименее значимом месте мантиссы. .
- ^ Перейти обратно: а б «Техническое справочное руководство Cortex-A7 MPCore – 1.3 Особенности» . РУКА . Проверено 11 июля 2014 г.
- ^ «ArmHardFloatPort — Debian Wiki» . Wiki.debian.org. 20 августа 2012 года . Проверено 8 января 2014 г.
- ^ «Процессор Cortex-A9» . Arm.com . Проверено 21 ноября 2011 г.
- ^ «О МРЕ Cortex-A9 NEON» . Arm.com . Проверено 21 ноября 2011 г.
- ^ «US20050125476A1» .
- ^ «US20080141004A1» .
- ^ «Опции ARM» . Руководство по сбору компиляторов GNU . Проверено 20 сентября 2019 г.
- ^ Ne10: проект открытой оптимизированной библиотеки программного обеспечения для архитектуры ARM на GitHub.
- ^ Джозеф Ю. «Введение в архитектуру ARMv8.1-M» (PDF) . Проверено 18 июля 2022 г.
- ^ «Genode — исследование технологии ARM TrustZone» . Проверено 10 июля 2015 г.
- ^ «ARM объявляет о доступности программного обеспечения DRM для мобильных потребителей на основе технологии ARM TrustZone» (пресс-релиз). News.thomasnet.com . Проверено 18 апреля 2009 г.
- ^ Лагинимайнеб (8 октября 2015 г.). «Биты, пожалуйста!: Полный эксплойт TrustZone для MSM8974» . Биты, пожалуйста! . Проверено 3 мая 2016 г.
- ^ Ди Шен. «Атака на ваше «доверенное ядро» с использованием TrustZone на Android» (PDF) . Брифинги «Черной шляпы» . Проверено 3 мая 2016 г.
- ^ «Программное обеспечение с открытым исходным кодом ARM TrustZone и ARM Hypervisor» . Откройте виртуализацию. Архивировано из оригинала 14 июня 2013 года . Проверено 14 июня 2013 г.
- ^ «Технология безопасности AMD» . АМД . Архивировано из оригинала 23 июля 2016 года . Проверено 6 июля 2016 г.
- ^ Смит, Райан (13 июня 2012 г.). «APU AMD 2013 будут включать процессор ARM Cortex A5 для функций доверительной зоны» . АнандТех . Проверено 6 июля 2016 г.
- ^ Перейти обратно: а б Шимпи, Ананд Лал (29 апреля 2014 г.). «Обзор производительности AMD Beema Mullins Architecture A10 micro 6700T» . АнандТех . Проверено 6 июля 2016 г.
- ^ Уолтон, Джаред (4 июня 2014 г.). «AMD выпускает мобильные APU Kaveri» . АнандТех . Проверено 6 июля 2016 г.
- ^ «Корень доверия» (информационный документ). Самсунг Электроникс . Апрель 2016.
- ^ «Справочное руководство по архитектуре ARM» (PDF) . п. Б4-8. Архивировано из оригинала (PDF) 6 февраля 2009 года.
Биты APX и XN (никогда не выполнять) были добавлены в VMSAv6 [Архитектура системы виртуальной памяти].
- ^ Справочное руководство по архитектуре ARM, версии ARMv7-A и ARMv7-R . АРМ Лимитед.
- ^ «Кортекс-А65АЕ» . ARM-разработчик . Проверено 26 апреля 2019 г.
- ^ «AppliedMicro представляет первое в мире 64-битное ядро ARM v8» (пресс-релиз). ПрикладнойМикро. 28 октября 2011 года . Проверено 11 февраля 2014 г.
- ^ «Samsung Exynos 5433 представляет собой SoC A57/A53 ARM» . АнандТех . Проверено 17 сентября 2014 г.
- ^ «Техническое справочное руководство процессора ARM Cortex-A53 MPCore: Расширение криптографии» . РУКА . Проверено 11 сентября 2016 г.
- ^ Перейти обратно: а б «Влияние реализованных уровней исключений» . Изучите архитектуру — Модель исключений AArch64 . Рука.
- ^ «Кортекс-А32» . Разработчик рук .
- ^ «Кортекс-А34» . Разработчик рук .
- ^ «Кортекс-А72» . Разработчик рук .
- ^ Фрумусану, Андрей (3 сентября 2020 г.). «ARM анонсировала Cortex-R82: первый 64-битный процессор реального времени» . АнандТех .
- ^ Фрумусану, Андрей (30 марта 2021 г.). «Arm анонсирует архитектуру Armv9: SVE2, безопасность и следующее десятилетие» . АнандТех .
- ^ Харрод, Алекс (30 марта 2021 г.). «Решение Arm для удовлетворения будущих потребностей в области искусственного интеллекта, безопасности и специализированных вычислений — v9» (пресс-релиз). Арм, ООО
- ^ «Программа соответствия SystemReady» . Рука .
- ^ «Архитектура системы основания руки» . Рука .
- ^ «Архитектура базовой системы сервера Arm» . Рука .
- ^ «Требования к загрузке подставки для рук» . Рука .
- ^ «Базовые требования безопасности при загрузке» . Рука .
- ^ «Спецификация требований Arm SystemReady» . Рука .
- ^ Осборн, Чарли. «ARM анонсирует архитектуру безопасности PSA для устройств IoT» . ЗДНет.
- ^ Перейти обратно: а б Вонг, Уильям (25 октября 2017 г.). «Архитектура безопасности платформы ARM ориентирована на Cortex-M» . Электронный дизайн . Архивировано из оригинала 8 мая 2019 года.
- ^ Хоффенберг, Стив (31 октября 2017 г.). «ARM: Безопасность — это не просто технологический императив, это социальная ответственность» . Исследования ВДЦ . Архивировано из оригинала 28 сентября 2023 года.
- ^ Армасу, Люциан (22 февраля 2018 г.). «ARM раскрывает более подробную информацию об архитектуре безопасности своей платформы IoT» . Аппаратное обеспечение Тома .
- ^ Уильямс, Крис. «ARM PSA IoT API? BRB… Набор инструментов для обеспечения безопасности подключенных к сети комплектов открывает еще больше возможностей» . Регистр .
- ^ Хейс, Кэролайн (25 февраля 2019 г.). «Встроенный мир: Arm представляет в PSA четвертый элемент безопасности» . Еженедельник электроники .
- ^ «Сертификация PSA: укрепление доверия к Интернету вещей» . Сертифицирован PSA .
- ^ Перейти обратно: а б «Сертификация PSA: укрепление доверия, повышение ценности» . ЭЭ Таймс . 4 марта 2019 г.
- ^ «Важность стандартов безопасности и регулирования в эпоху Интернета вещей составляет 6 триллионов долларов» . Интернет вещей сейчас. 16 марта 2020 г.
- ^ МакГрегор, Джим (4 марта 2019 г.). «Arm представляет сертификационное тестирование безопасности для Интернета вещей» . Форбс .
- ^ Спид, Ричард (26 февраля 2019 г.). «Azure IoT направляется в космос, чтобы поддерживать связь на периферии, благодаря Inmarsat» . Регистр .
- ^ «Технические характеристики ОС-9» . Микропрограммное обеспечение .
- ^ Перейти обратно: а б «Фарос» . СоурсФордж . Проверено 24 мая 2018 г.
- ^ «Безопасная и надежная виртуализация PikeOS» . Проверено 10 июля 2013 г.
- ^ Перейти обратно: а б «Сертифицированные по безопасности операционные системы реального времени – поддерживаемые процессоры» .
- ^ «Порт платформы ARM» . сайт opensolaris.org. Архивировано из оригинала 2 декабря 2012 года . Проверено 29 декабря 2012 г.
- ^ «Мультивизор на базе INTEGRITY от Green Hills Software представляет первое в отрасли 64-битное безопасное решение виртуализации» . ghs.com . Проверено 14 марта 2018 г.
- ^ «Операционная система реального времени Enea OSE для 5G и LTE-A | Enea» . Enea.com . Архивировано из оригинала 1 января 2019 года . Проверено 17 апреля 2018 г.
- ^ «Поддерживаемые платформы» . docs.sel4.systems . Проверено 23 ноября 2018 г.
- ^ «Платформа разработки программного обеспечения QNX (SDP 7.0) | BlackBerry QNX» . blackberry.qnx.com . Проверено 27 июля 2020 г.
- ^ «Wind River выпускает 64-битную ОСРВ VxWorks» (пресс-релиз). Речные системы ветров . 28 февраля 2011 года . Проверено 24 октября 2023 г.
- ^ «Тюрьма-АРМ» . Тюремная вики . 20 июня 2022 г.
- ^ Линус Торвальдс (1 октября 2012 г.). «Re: [GIT PULL] Arm64: порт ядра Linux» . Список рассылки ядра Linux (Список рассылки) . Проверено 2 мая 2019 г.
- ^ Ларабель, Майкл (27 февраля 2013 г.). «64-битная ARM-версия Ubuntu/Debian загружается» . Фороникс . Проверено 17 августа 2014 г.
- ^ «Новости проекта Debian – 14 августа 2014 г.» . Дебиан . 14 августа 2014 года . Проверено 17 августа 2014 г.
- ^ «Сервер Ubuntu для ARM» . Ubuntu.com .
- ^ «Архитектуры/AArch64» . Проверено 16 января 2015 г.
- ^ «Портал:ARM/AArch64» . Проверено 16 января 2015 г.
- ^ «Примечания к выпуску SUSE Linux Enterprise 12 SP2» . Проверено 11 ноября 2016 г.
- ^ «Red Hat представляет поддержку ARM-серверов для Red Hat Enterprise Linux» . redhat.com . Проверено 18 января 2019 г.
- ^ «Обновление проекта 64-битной архитектуры ARM» . Фонд FreeBSD. 24 ноября 2014 г.
- ^ «OpenBSD/arm64» . Проверено 25 сентября 2023 г.
- ^ «NetBSD/arm64» . Проверено 5 августа 2018 г.
- ^ «HP и Asus анонсируют первые ПК с Windows 10 ARM: 20 часов автономной работы, гигабитный LTE» . Арс Техника . Проверено 22 января 2018 г.
Эта новая версия Windows 10 — первая 64-битная операционная система Microsoft на ARM. Он будет запускать x86- и 32-битные приложения ARM из Магазина, а со временем и 64-битные приложения ARM. Однако Microsoft еще не завершила работу над 64-битным ARM SDK. Многие компоненты уже установлены (например, есть 64-битный компилятор ARM), но компания еще не принимает 64-битные ARM-приложения, отправляемые в Магазин, а также нет никаких 64-битных настольных приложений ARM.
- ^ Хасан, Мехеди (10 декабря 2016 г.). «В Windows 10 на ARM64 появляются первые скомпилированные приложения» . MSPoweruser .
- ^ Филиппидис, Катрина (1 июня 2018 г.). «VLC становится одним из первых приложений для Windows ARM64» . Engadget .
- ^ Свитгалл, Марк (15 ноября 2018 г.). «Официальная поддержка Windows 10 на платформе ARM» . Разработчик Windows. Блоги Windows . Майкрософт . Проверено 17 декабря 2019 г.
- ^ Гартенберг, Хаим (12 ноября 2020 г.). «macOS Big Sur теперь доступна для загрузки» . Грань . Проверено 13 ноября 2020 г.
- ^ Клевер, Джули (23 июня 2020 г.). «Rosetta не будет поддерживать приложения виртуализации x86 под управлением Windows» . МакСлухи . Проверено 13 ноября 2020 г.
- ^ «ARM — Официальная винная вики» . Проверено 10 июля 2015 г.
- ^ «ARM64 — Официальная винная вики» . Проверено 10 июля 2015 г.
- ^ «Обновления безопасности ARM» . ARM-разработчик . Проверено 24 мая 2018 г.
Библиография
[ редактировать ]- Эванс, Мэтт (27 декабря 2019 г.). Выступление Архимеда «Окончательный Желудь» . Расписание 36-го Конгресса Хаос-коммуникаций. Ютуб . Архивировано из оригинала 11 декабря 2021 года – на сайте media.ccc.de.
Дальнейшее чтение
[ редактировать ]Внешние ссылки
[ редактировать ]
- Официальный сайт
, ООО АРМ.
Руководства по архитектуре
[ редактировать ]- АРМ Лимитед (1996–2005). «Справочное руководство по архитектуре ARM» . документация-service.arm.com . Проверено 16 июля 2021 г. - охватывает ARMv4, ARMv4T, ARMv5T, (ARMv5TExP), ARMv5TE, ARMv5TEJ и ARMv6.
- АРМ Лимитед (2007–2018 гг.). «Справочное руководство по архитектуре Armv6-M» . Документация ARM . Проверено 17 июля 2021 г.
- АРМ Лимитед (2007–2018 гг.). «Справочное руководство по архитектуре ARM для версий ARMv7-A и ARMv7-R» . Документация ARM . Проверено 17 июля 2021 г.
- АРМ Лимитед (2006–2021 гг.). «Справочное руководство по архитектуре ARMv7-M» . Документация ARM . Проверено 24 августа 2022 г.
- АРМ Лимитед (2013–2022 гг.). «Справочное руководство по архитектуре кронштейнов для архитектуры А-профиля» . Документация ARM . Проверено 24 августа 2022 г.
- АРМ Лимитед (2016–2020 гг.). «Дополнение к справочному руководству по архитектуре ARM — ARMv8 для профиля архитектуры ARMv8-R AArch32» . Документация ARM . Проверено 17 июля 2021 г.
- АРМ Лимитед (2020–2022 гг.). «Дополнение к справочному руководству по архитектуре Arm — Armv8, для профиля архитектуры Armv8-R AArch64» . Документация ARM . Проверено 24 августа 2022 г.
- АРМ Лимитед (2015–2022 гг.). «Справочное руководство по архитектуре Armv8-M» . Документация ARM . Проверено 24 августа 2022 г.
- АРМ Лимитед (2021 г.). «Архитектура набора команд Arm Armv9-A A64» . Документация ARM . Проверено 17 июля 2021 г.
- «Расширения виртуализации ARM» . Архивировано из оригинала 18 декабря 2013 года.
Карточки с краткими рекомендациями
[ редактировать ]Инструкции
[ редактировать ]- Большой палец. Архивировано 20 июня 2020 г. в Wayback Machine.
- ARM и Thumb-2. Архивировано 20 июня 2020 года на Wayback Machine.
- Векторные числа с плавающей запятой. Архивировано 19 июня 2020 года на Wayback Machine.
Коды операций
[ редактировать ]- Большой палец. Архивировано 30 июля 2022 года в Wayback Machine . Дополнительный архив: 22 августа 2022 г.
- ARM. Архивировано 7 июня 2022 года в Wayback Machine . Дополнительный архив: 22 августа 2022 г.
- Директивы ассемблера GNU. Архивировано 30 апреля 2022 года на Wayback Machine . Дополнительный архив: 22 августа 2022 г.