Жизнь на Марсе
Возможность жизни на Марсе является предметом интереса астробиологии из-за планеты близости и сходства с Землей . На сегодняшний день на Марсе не обнаружено убедительных доказательств существования прошлой или настоящей жизни. Совокупные данные свидетельствуют о том, что в древний период Ноя на поверхности Марса была жидкая вода и, возможно, она была пригодна для обитания микроорганизмов, но пригодные для жизни условия не обязательно указывают на жизнь. [1] [2]
Научные поиски доказательств существования жизни начались в 19 веке и продолжаются сегодня посредством телескопических исследований и развертывания зондов в поисках воды, химических биосигнатур в почве и камнях на поверхности планеты, а также газов -биомаркеров в атмосфере. [3]
Марс представляет особый интерес для изучения происхождения жизни из-за его сходства с ранней Землей. Это особенно верно, поскольку на Марсе холодный климат и отсутствует тектоника плит или дрейф континентов , поэтому он практически не изменился с конца гесперианского периода. Возраст по меньшей мере двух третей поверхности Марса превышает 3,5 миллиарда лет, и она могла быть обитаемой 4,48 миллиарда лет назад, на 500 миллионов лет раньше, чем появились самые ранние известные земные формы жизни; [4] Таким образом, Марс, возможно, является лучшим примером пребиотических условий, ведущих к жизни, даже если жизнь там не существует или никогда не существовала. [5] [6]
Following the confirmation of the past existence of surface liquid water, the Curiosity, Perseverance and Opportunity rovers started searching for evidence of past life, including a past biosphere based on autotrophic, chemotrophic, or chemolithoautotrophic microorganisms, as well as ancient water, including fluvio-lacustrine environments (plains related to ancient rivers or lakes) that may have been habitable.[7][8][9][10] The search for evidence of habitability, taphonomy (related to fossils), and organic compounds on Mars is now a primary objective for space agencies.
The findings of organic compounds inside sedimentary rocks and of boron on Mars are of interest as they are precursors for prebiotic chemistry. Such findings, along with previous discoveries that liquid water was clearly present on ancient Mars, further supports the possible early habitability of Gale Crater on Mars.[11][12] Currently, the surface of Mars is bathed with ionizing radiation, and Martian soil is rich in perchlorates toxic to microorganisms.[13][14] Therefore, the consensus is that if life exists—or existed—on Mars, it could be found or is best preserved in the subsurface, away from present-day harsh surface processes.
In June 2018, NASA announced the detection of seasonal variation of methane levels on Mars. Methane could be produced by microorganisms or by geological means.[15] The European ExoMars Trace Gas Orbiter started mapping the atmospheric methane in April 2018, and the 2022 ExoMars rover Rosalind Franklin was planned to drill and analyze subsurface samples before the programme's indefinite suspension, while the NASA Mars 2020 rover Perseverance, having landed successfully, will cache dozens of drill samples for their potential transport to Earth laboratories in the late 2020s or 2030s. As of February 8, 2021, an updated status of studies considering the possible detection of lifeforms on Venus (via phosphine) and Mars (via methane) was reported.[16]
Early speculation
[edit]Mars's polar ice caps were discovered in the mid-17th century.[citation needed] In the late 18th century, William Herschel proved they grow and shrink alternately, in the summer and winter of each hemisphere. By the mid-19th century, astronomers knew that Mars had certain other similarities to Earth, for example that the length of a day on Mars was almost the same as a day on Earth. They also knew that its axial tilt was similar to Earth's, which meant it experienced seasons just as Earth does—but of nearly double the length owing to its much longer year. These observations led to increasing speculation that the darker albedo features were water and the brighter ones were land, whence followed speculation on whether Mars may be inhabited by some form of life.[17]
In 1854, William Whewell, a fellow of Trinity College, Cambridge, theorized that Mars had seas, land and possibly life forms.[18] Speculation about life on Mars exploded in the late 19th century, following telescopic observation by some observers of apparent Martian canals—which were later found to be optical illusions. Despite this, in 1895, American astronomer Percival Lowell published his book Mars, followed by Mars and its Canals in 1906,[19] proposing that the canals were the work of a long-gone civilization.[20] This idea led British writer H. G. Wells to write The War of the Worlds in 1897, telling of an invasion by aliens from Mars who were fleeing the planet's desiccation.[21]
The 1907 book Is Mars Habitable? by British naturalist Alfred Russel Wallace was a reply to, and refutation of, Lowell's Mars and Its Canals. Wallace's book concluded that Mars "is not only uninhabited by intelligent beings such as Mr. Lowell postulates, but is absolutely uninhabitable."[22] Historian Charles H. Smith refers to Wallace's book as one of the first works in the field of astrobiology.[23]
Spectroscopic analysis of Mars's atmosphere began in earnest in 1894, when U.S. astronomer William Wallace Campbell showed that neither water nor oxygen were present in the Martian atmosphere.[24] The influential observer Eugène Antoniadi used the 83-cm (32.6 inch) aperture telescope at Meudon Observatory at the 1909 opposition of Mars and saw no canals, the outstanding photos of Mars taken at the new Baillaud dome at the Pic du Midi observatory also brought formal discredit to the Martian canals theory in 1909,[25] and the notion of canals began to fall out of favor.[24]
Habitability
[edit]Chemical, physical, geological, and geographic attributes shape the environments on Mars. Isolated measurements of these factors may be insufficient to deem an environment habitable, but the sum of measurements can help predict locations with greater or lesser habitability potential.[26] The two current ecological approaches for predicting the potential habitability of the Martian surface use 19 or 20 environmental factors, with an emphasis on water availability, temperature, the presence of nutrients, an energy source, and protection from solar ultraviolet and galactic cosmic radiation.[27][28]
Scientists do not know the minimum number of parameters for determination of habitability potential, but they are certain it is greater than one or two of the factors in the table below.[26] Similarly, for each group of parameters, the habitability threshold for each is to be determined.[26] Laboratory simulations show that whenever multiple lethal factors are combined, the survival rates plummet quickly.[29] There are no full-Mars simulations published yet that include all of the biocidal factors combined.[29] Furthermore, the possibility of Martian life having a far different biochemistry and habitability requirements than the terrestrial biosphere is an open question. A common hypothesis is methanogenic Martian life, and while such organisms exist on Earth too, they are exceptionally rare and cannot survive in the majority of terrestrial environments that contain oxygen. [30]
Habitability factors[28] | |
---|---|
Water |
|
Chemical environment |
|
Energy for metabolism |
|
Conducive physical conditions |
|
Past
[edit]Recent models have shown that, even with a dense CO2 atmosphere, early Mars was colder than Earth has ever been.[31][32][33][34] Transiently warm conditions related to impacts or volcanism could have produced conditions favoring the formation of the late Noachian valley networks, even though the mid-late Noachian global conditions were probably icy. Local warming of the environment by volcanism and impacts would have been sporadic, but there should have been many events of water flowing at the surface of Mars.[34] Both the mineralogical and the morphological evidence indicates a degradation of habitability from the mid Hesperian onward. The exact causes are not well understood but may be related to a combination of processes including loss of early atmosphere, or impact erosion, or both.[34] Billions of years ago, before this degradation, the surface of Mars was apparently fairly habitable, consisted of liquid water and clement weather, though it is unknown if life existed on Mars.[35]

The loss of the Martian magnetic field strongly affected surface environments through atmospheric loss and increased radiation; this change significantly degraded surface habitability.[37] When there was a magnetic field, the atmosphere would have been protected from erosion by the solar wind, which would ensure the maintenance of a dense atmosphere, necessary for liquid water to exist on the surface of Mars.[38] The loss of the atmosphere was accompanied by decreasing temperatures. Part of the liquid water inventory sublimed and was transported to the poles, while the rest becametrapped in permafrost, a subsurface ice layer.[34]
Observations on Earth and numerical modeling have shown that a crater-forming impact can result in the creation of a long-lasting hydrothermal system when ice is present in the crust. For example, a 130 km large crater could sustain an active hydrothermal system for up to 2 million years, that is, long enough for microscopic life to emerge,[34] but unlikely to have progressed any further down the evolutionary path.[39]
Soil and rock samples studied in 2013 by NASA's Curiosity rover's onboard instruments brought about additional information on several habitability factors.[40] The rover team identified some of the key chemical ingredients for life in this soil, including sulfur, nitrogen, hydrogen, oxygen, phosphorus and possibly carbon, as well as clay minerals, suggesting a long-ago aqueous environment—perhaps a lake or an ancient streambed—that had neutral acidity and low salinity.[40] On December 9, 2013, NASA reported that, based on evidence from Curiosity studying Aeolis Palus, Gale Crater contained an ancient freshwater lake which could have been a hospitable environment for microbial life.[41][42] The confirmation that liquid water once flowed on Mars, the existence of nutrients, and the previous discovery of a past magnetic field that protected the planet from cosmic and solar radiation,[43][44] together strongly suggest that Mars could have had the environmental factors to support life.[45][46] The assessment of past habitability is not in itself evidence that Martian life has ever actually existed. If it did, it was probably microbial, existing communally in fluids or on sediments, either free-living or as biofilms, respectively.[37] The exploration of terrestrial analogues provide clues as to how and where best look for signs of life on Mars.[47]
Impactite, shown to preserve signs of life on Earth, was discovered on Mars and could contain signs of ancient life, if life ever existed on the planet.[48]
On June 7, 2018, NASA announced that the Curiosity rover had discovered organic molecules in sedimentary rocks dating to three billion years old.[49][50] The detection of organic molecules in rocks indicate that some of the building blocks for life were present.[51][52]
Present
[edit]Conceivably, if life exists (or existed) on Mars, evidence of life could be found, or is best preserved, in the subsurface, away from present-day harsh surface conditions.[53] Present-day life on Mars, or its biosignatures, could occur kilometers below the surface, or in subsurface geothermal hot spots, or it could occur a few meters below the surface. The permafrost layer on Mars is only a couple of centimeters below the surface, and salty brines can be liquid a few centimeters below that but not far down. Water is close to its boiling point even at the deepest points in the Hellas basin, and so cannot remain liquid for long on the surface of Mars in its present state, except after a sudden release of underground water.[54][55][56]
So far, NASA has pursued a "follow the water" strategy on Mars and has not searched for biosignatures for life there directly since the Viking missions. The consensus by astrobiologists is that it may be necessary to access the Martian subsurface to find currently habitable environments.[53]
Cosmic radiation
[edit]In 1965, the Mariner 4 probe discovered that Mars had no global magnetic field that would protect the planet from potentially life-threatening cosmic radiation and solar radiation; observations made in the late 1990s by the Mars Global Surveyor confirmed this discovery.[57] Scientists speculate that the lack of magnetic shielding helped the solar wind blow away much of Mars's atmosphere over the course of several billion years.[58] As a result, the planet has been vulnerable to radiation from space for about 4 billion years.[59]
Recent in-situ data from Curiosity rover indicates that ionizing radiation from galactic cosmic rays (GCR) and solar particle events (SPE) may not be a limiting factor in habitability assessments for present-day surface life on Mars. The level of 76 mGy per year measured by Curiosity is similar to levels inside the ISS.[60]
Cumulative effects
[edit]Curiosity rover measured ionizing radiation levels of 76 mGy per year.[61] This level of ionizing radiation is sterilizing for dormant life on the surface of Mars. It varies considerably in habitability depending on its orbital eccentricity and the tilt of its axis. If the surface life has been reanimated as recently as 450,000 years ago, then rovers on Mars could find dormant but still viable life at a depth of one meter below the surface, according to an estimate.[62] Even the hardiest cells known could not possibly survive the cosmic radiation near the surface of Mars since Mars lost its protective magnetosphere and atmosphere.[63][64] After mapping cosmic radiation levels at various depths on Mars, researchers have concluded that over time, any life within the first several meters of the planet's surface would be killed by lethal doses of cosmic radiation.[63][65][66] The team calculated that the cumulative damage to DNA and RNA by cosmic radiation would limit retrieving viable dormant cells on Mars to depths greater than 7.5 meters below the planet's surface.[65]Even the most radiation-tolerant terrestrial bacteria would survive in dormant spore state only 18,000 years at the surface; at 2 meters—the greatest depth at which the ExoMars rover will be capable of reaching—survival time would be 90,000 to half a million years, depending on the type of rock.[67]
Data collected by the Radiation assessment detector (RAD) instrument on board the Curiosity rover revealed that the absorbed dose measured is 76 mGy/year at the surface,[68] and that "ionizing radiation strongly influences chemical compositions and structures, especially for water, salts, and redox-sensitive components such as organic molecules."[68] Regardless of the source of Martian organic compounds (meteoric, geological, or biological), its carbon bonds are susceptible to breaking and reconfiguring with surrounding elements by ionizing charged particle radiation.[68] These improved subsurface radiation estimates give insight into the potential for the preservation of possible organic biosignatures as a function of depth as well as survival times of possible microbial or bacterial life forms left dormant beneath the surface.[68] The report concludes that the in situ "surface measurements—and subsurface estimates—constrain the preservation window for Martian organic matter following exhumation and exposure to ionizing radiation in the top few meters of the Martian surface."[68]
In September 2017, NASA reported radiation levels on the surface of the planet Mars were temporarily doubled and were associated with an aurora 25 times brighter than any observed earlier, due to a major, and unexpected, solar storm in the middle of the month.[69]
UV radiation
[edit]On UV radiation, a 2014 report concludes [70] that "[T]he Martian UV radiation environment is rapidly lethal to unshielded microbes but can be attenuated by global dust storms and shielded completely by < 1 mm of regolith or by other organisms." In addition, laboratory research published in July 2017 demonstrated that UV irradiated perchlorates cause a 10.8-fold increase in cell death when compared to cells exposed to UV radiation after 60 seconds of exposure.[71][72] The penetration depth of UV radiation into soils is in the sub-millimeter to millimeter range and depends on the properties of the soil.[72]
Perchlorates
[edit]The Martian regolith is known to contain a maximum of 0.5% (w/v) perchlorate (ClO4−) that is toxic for most living organisms,[73] but since they drastically lower the freezing point of water and a few extremophiles can use it as an energy source (see Perchlorates - Biology) and grow at concentrations of up to 30% (w/v) sodium perchlorate[74] by physiologically adapting to increasing perchlorate concentrations,[75] it has prompted speculation of what their influence would be on habitability.[71][74][76][77][78]
Research published in July 2017 shows that when irradiated with a simulated Martian UV flux, perchlorates become even more lethal to bacteria (bactericide). Even dormant spores lost viability within minutes.[71] In addition, two other compounds of the Martian surface, iron oxides and hydrogen peroxide, act in synergy with irradiated perchlorates to cause a 10.8-fold increase in cell death when compared to cells exposed to UV radiation after 60 seconds of exposure.[71][72] It was also found that abraded silicates (quartz and basalt) lead to the formation of toxic reactive oxygen species.[79] The researchers concluded that "the surface of Mars is lethal to vegetative cells and renders much of the surface and near-surface regions uninhabitable."[80] This research demonstrates that the present-day surface is more uninhabitable than previously thought,[71][81] and reinforces the notion to inspect at least a few meters into the ground to ensure the levels of radiation would be relatively low.[81][82]
However, researcher Kennda Lynch discovered the first-known instance of a habitat containing perchlorates and perchlorates-reducing bacteria in an analog environment: a paleolake in Pilot Valley, Great Salt Lake Desert, Utah.[83] She has been studying the biosignatures of these microbes, and is hoping that the Mars Perseverance rover will find matching biosignatures at its Jezero Crater site.[84][85]
Recurrent slope lineae
[edit]Recurrent slope lineae (RSL) features form on Sun-facing slopes at times of the year when the local temperatures reach above the melting point for ice. The streaks grow in spring, widen in late summer and then fade away in autumn. This is hard to model in any other way except as involving liquid water in some form, though the streaks themselves are thought to be a secondary effect and not a direct indication of the dampness of the regolith. Although these features are now confirmed to involve liquid water in some form, the water could be either too cold or too salty for life. At present they are treated as potentially habitable, as "Uncertain Regions, to be treated as Special Regions".).[86][87] They were suspected as involving flowing brines back then.[88][89][90][91]
The thermodynamic availability of water (water activity) strictly limits microbial propagation on Earth, particularly in hypersaline environments, and there are indications that the brine ionic strength is a barrier to the habitability of Mars. Experiments show that high ionic strength, driven to extremes on Mars by the ubiquitous occurrence of divalent ions, "renders these environments uninhabitable despite the presence of biologically available water."[92]
Nitrogen fixation
[edit]After carbon, nitrogen is arguably the most important element needed for life. Thus, measurements of nitrate over the range of 0.1% to 5% are required to address the question of its occurrence and distribution. There is nitrogen (as N2) in the atmosphere at low levels, but this is not adequate to support nitrogen fixation for biological incorporation.[93] Nitrogen in the form of nitrate could be a resource for human exploration both as a nutrient for plant growth and for use in chemical processes. On Earth, nitrates correlate with perchlorates in desert environments, and this may also be true on Mars. Nitrate is expected to be stable on Mars and to have formed by thermal shock from impact or volcanic plume lightning on ancient Mars.[94]
On March 24, 2015, NASA reported that the SAM instrument on the Curiosity rover detected nitrates by heating surface sediments. The nitrogen in nitrate is in a "fixed" state, meaning that it is in an oxidized form that can be used by living organisms. The discovery supports the notion that ancient Mars may have been hospitable for life.[94][95][96] It is suspected that all nitrate on Mars is a relic, with no modern contribution.[97] Nitrate abundance ranges from non-detection to 681 ± 304 mg/kg in the samples examined until late 2017.[97] Modeling indicates that the transient condensed water films on the surface should be transported to lower depths (≈10 m) potentially transporting nitrates, where subsurface microorganisms could thrive.[98]
In contrast, phosphate, one of the chemical nutrients thought to be essential for life, is readily available on Mars.[99]
Low pressure
[edit]Further complicating estimates of the habitability of the Martian surface is the fact that very little is known about the growth of microorganisms at pressures close to those on the surface of Mars. Some teams determined that some bacteria may be capable of cellular replication down to 25 mbar, but that is still above the atmospheric pressures found on Mars (range 1–14 mbar).[100] In another study, twenty-six strains of bacteria were chosen based on their recovery from spacecraft assembly facilities, and only Serratia liquefaciens strain ATCC 27592 exhibited growth at 7 mbar, 0 °C, and CO2-enriched anoxic atmospheres.[100]
Liquid water
[edit]Liquid water is a necessary but not sufficient condition for life as humans know it, as habitability is a function of a multitude of environmental parameters.[101] Liquid water cannot exist on the surface of Mars except at the lowest elevations for minutes or hours.[102][103] Liquid water does not appear at the surface itself,[104] but it could form in minuscule amounts around dust particles in snow heated by the Sun.[105][106][unreliable source?] Also, the ancient equatorial ice sheets beneath the ground may slowly sublimate or melt, accessible from the surface via caves.[107][108][109][110]
Scalloped terrain led to the discovery of a large amount of underground ice
enough water to fill Lake Superior (November 22, 2016)[111][112][113]
Water on Mars exists almost exclusively as water ice, located in the Martian polar ice caps and under the shallow Martian surface even at more temperate latitudes.[114][115] A small amount of water vapor is present in the atmosphere.[116] There are no bodies of liquid water on the Martian surface because its atmospheric pressure at the surface averages 600 pascals (0.087 psi)—about 0.6% of Earth's mean sea level pressure—and because the temperature is far too low, (210 K (−63 °C)) leading to immediate freezing. Despite this, about 3.8 billion years ago,[117] there was a denser atmosphere, higher temperature, and vast amounts of liquid water flowed on the surface,[118][119][120][121] including large oceans.[122][123][124][125][126]


Site of Subglacial Water
(July 25, 2018)
It has been estimated that the primordial oceans on Mars would have covered between 36%[127] and 75% of the planet.[128] On November 22, 2016, NASA reported finding a large amount of underground ice in the Utopia Planitia region of Mars. The volume of water detected has been estimated to be equivalent to the volume of water in Lake Superior.[111][112][113]Analysis of Martian sandstones, using data obtained from orbital spectrometry, suggests that the waters that previously existed on the surface of Mars would have had too high a salinity to support most Earth-like life. Tosca et al. found that the Martian water in the locations they studied all had water activity, aw ≤ 0.78 to 0.86—a level fatal to most Terrestrial life.[129] Haloarchaea, however, are able to live in hypersaline solutions, up to the saturation point.[130]
In June 2000, possible evidence for current liquid water flowing at the surface of Mars was discovered in the form of flood-like gullies.[131][132] Additional similar images were published in 2006, taken by the Mars Global Surveyor, that suggested that water occasionally flows on the surface of Mars. The images showed changes in steep crater walls and sediment deposits, providing the strongest evidence yet that water coursed through them as recently as several years ago.
There is disagreement in the scientific community as to whether or not the recent gully streaks were formed by liquid water. Some suggest the flows were merely dry sand flows.[133][134][135] Others suggest it may be liquid brine near the surface,[136][137][138] but the exact source of the water and the mechanism behind its motion are not understood.[139]
In July 2018, scientists reported the discovery of a subglacial lake on Mars, 1.5 km (0.93 mi) below the southern polar ice cap, and extending sideways about 20 km (12 mi), the first known stable body of water on the planet.[140][141][142][143] The lake was discovered using the MARSIS radar on board the Mars Express orbiter, and the profiles were collected between May 2012 and December 2015.[144] The lake is centered at 193°E, 81°S, a flat area that does not exhibit any peculiar topographic characteristics but is surrounded by higher ground, except on its eastern side, where there is a depression.[140]
Silica
[edit]
In May 2007, the Spirit rover disturbed a patch of ground with its inoperative wheel, uncovering an area 90% rich in silica.[145] The feature is reminiscent of the effect of hot spring water or steam coming into contact with volcanic rocks. Scientists consider this as evidence of a past environment that may have been favorable for microbial life and theorize that one possible origin for the silica may have been produced by the interaction of soil with acid vapors produced by volcanic activity in the presence of water.[146]
Based on Earth analogs, hydrothermal systems on Mars would be highly attractive for their potential for preserving organic and inorganic biosignatures.[147][148][149] For this reason, hydrothermal deposits are regarded as important targets in the exploration for fossil evidence of ancient Martian life.[150][151][152]
Possible biosignatures
[edit]In May 2017, evidence of the earliest known life on land on Earth may have been found in 3.48-billion-year-old geyserite and other related mineral deposits (often found around hot springs and geysers) uncovered in the Pilbara Craton of Western Australia.[153][154] These findings may be helpful in deciding where best to search for early signs of life on the planet Mars.[153][154]
Methane
[edit]Methane (CH4) is chemically unstable in the current oxidizing atmosphere of Mars. It would quickly break down due to ultraviolet radiation from the Sun and chemical reactions with other gases. Therefore, a persistent presence of methane in the atmosphere may imply the existence of a source to continually replenish the gas.
Trace amounts of methane, at the level of several parts per billion (ppb), were first reported in Mars's atmosphere by a team at the NASA Goddard Space Flight Center in 2003.[155][156] Large differences in the abundances were measured between observations taken in 2003 and 2006, which suggested that the methane was locally concentrated and probably seasonal.[157] On June 7, 2018, NASA announced it has detected a seasonal variation of methane levels on Mars.[15][158][51][52][159][160][161][50]
The ExoMars Trace Gas Orbiter (TGO), launched in March 2016, began on April 21, 2018, to map the concentration and sources of methane in the atmosphere,[162][163] as well as its decomposition products such as formaldehyde and methanol. As of May 2019, the Trace Gas Orbiter showed that the concentration of methane is under detectable level (< 0.05 ppbv).[164][165]
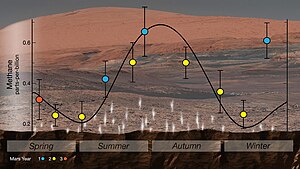
The principal candidates for the origin of Mars's methane include non-biological processes such as water-rock reactions, radiolysis of water, and pyrite formation, all of which produce H2 that could then generate methane and other hydrocarbons via Fischer–Tropsch synthesis with CO and CO2.[166] It has also been shown that methane could be produced by a process involving water, carbon dioxide, and the mineral olivine, which is known to be common on Mars.[167] Although geologic sources of methane such as serpentinization are possible, the lack of current volcanism, hydrothermal activity or hotspots[168] are not favorable for geologic methane.
Living microorganisms, such as methanogens, are another possible source, but no evidence for the presence of such organisms has been found on Mars,[169][170][171] until June 2019 as methane was detected by the Curiosity rover.[172] Methanogens do not require oxygen or organic nutrients, are non-photosynthetic, use hydrogen as their energy source and carbon dioxide (CO2) as their carbon source, so they could exist in subsurface environments on Mars.[173] If microscopic Martian life is producing the methane, it probably resides far below the surface, where it is still warm enough for liquid water to exist.[174]
Since the 2003 discovery of methane in the atmosphere, some scientists have been designing models and in vitro experiments testing the growth of methanogenic bacteria on simulated Martian soil, where all four methanogen strains tested produced substantial levels of methane, even in the presence of 1.0wt% perchlorate salt.[175]
A team led by Levin suggested that both phenomena—methane production and degradation—could be accounted for by an ecology of methane-producing and methane-consuming microorganisms.[176][177]

Research at the University of Arkansas presented in June 2015 suggested that some methanogens could survive in Mars's low pressure. Rebecca Mickol found that in her laboratory, four species of methanogens survived low-pressure conditions that were similar to a subsurface liquid aquifer on Mars. The four species that she tested were Methanothermobacter wolfeii, Methanosarcina barkeri, Methanobacterium formicicum, and Methanococcus maripaludis.[173] In June 2012, scientists reported that measuring the ratio of hydrogen and methane levels on Mars may help determine the likelihood of life on Mars.[169][170] According to the scientists, "low H2/CH4 ratios (less than approximately 40)" would "indicate that life is likely present and active".[169] The observed ratios in the lower Martian atmosphere were "approximately 10 times" higher "suggesting that biological processes may not be responsible for the observed CH4".[169] The scientists suggested measuring the H2 and CH4 flux at the Martian surface for a more accurate assessment. Other scientists have recently reported methods of detecting hydrogen and methane in extraterrestrial atmospheres.[178][179]
Even if rover missions determine that microscopic Martian life is the seasonal source of the methane, the life forms probably reside far below the surface, outside of the rover's reach.[180]
Formaldehyde
[edit]In February 2005, it was announced that the Planetary Fourier Spectrometer (PFS) on the European Space Agency's Mars Express Orbiter had detected traces of formaldehyde in the atmosphere of Mars. Vittorio Formisano, the director of the PFS, has speculated that the formaldehyde could be the byproduct of the oxidation of methane and, according to him, would provide evidence that Mars is either extremely geologically active or harboring colonies of microbial life.[181][182] NASA scientists consider the preliminary findings well worth a follow-up but have also rejected the claims of life.[183][184]
Viking lander biological experiments
[edit]The 1970s Viking program placed two identical landers on the surface of Mars tasked to look for biosignatures of microbial life on the surface. The 'Labeled Release' (LR) experiment gave a positive result for metabolism, while the gas chromatograph–mass spectrometer did not detect organic compounds. The LR was a specific experiment designed to test only a narrowly defined critical aspect of the theory concerning the possibility of life on Mars; therefore, the overall results were declared inconclusive.[24] No Mars lander mission has found meaningful traces of biomolecules or biosignatures. The claim of extant microbial life on Mars is based on old data collected by the Viking landers, currently reinterpreted as sufficient evidence of life, mainly by Gilbert Levin,[185][186] Joseph D. Miller,[187] Navarro,[188] Giorgio Bianciardi and Patricia Ann Straat.
Assessments published in December 2010 by Rafael Navarro-Gonzáles[189][190][191][192] indicate that organic compounds "could have been present" in the soil analyzed by both Viking 1 and 2. The study determined that perchlorate—discovered in 2008 by Phoenix lander[193][194]—can destroy organic compounds when heated, and produce chloromethane and dichloromethane as a byproduct, the identical chlorine compounds discovered by both Viking landers when they performed the same tests on Mars. Because perchlorate would have broken down any Martian organics, the question of whether or not Viking found organic compounds is still wide open.[195][196]
The Labeled Release evidence was not generally accepted initially, and, to this day lacks the consensus of the scientific community.[197]
Meteorites
[edit]As of 2018, there are 224 known Martian meteorites (some of which were found in several fragments).[198] These are valuable because they are the only physical samples of Mars available to Earth-bound laboratories. Some researchers have argued that microscopic morphological features found in ALH84001 are biomorphs, however this interpretation has been highly controversial and is not supported by the majority of researchers in the field.[199]
Seven criteria have been established for the recognition of past life within terrestrial geologic samples. Those criteria are:[199]
- Is the geologic context of the sample compatible with past life?
- Is the age of the sample and its stratigraphic location compatible with possible life?
- Does the sample contain evidence of cellular morphology and colonies?
- Is there any evidence of biominerals showing chemical or mineral disequilibria?
- Is there any evidence of stable isotope patterns unique to biology?
- Are there any organic biomarkers present?
- Are the features indigenous to the sample?
For general acceptance of past life in a geologic sample, essentially most or all of these criteria must be met. All seven criteria have not yet been met for any of the Martian samples.[199]
ALH84001
[edit]
In 1996, the Martian meteorite ALH84001, a specimen that is much older than the majority of Martian meteorites that have been recovered so far, received considerable attention when a group of NASA scientists led by David S. McKay reported microscopic features and geochemical anomalies that they considered to be best explained by the rock having hosted Martian bacteria in the distant past. Some of these features resembled terrestrial bacteria, aside from their being much smaller than any known form of life. Much controversy arose over this claim, and ultimately all of the evidence McKay's team cited as evidence of life was found to be explainable by non-biological processes. Although the scientific community has largely rejected the claim ALH 84001 contains evidence of ancient Martian life, the controversy associated with it is now seen as a historically significant moment in the development of exobiology.[200][201]

Nakhla
[edit]The Nakhla meteorite fell on Earth on June 28, 1911, on the locality of Nakhla, Alexandria, Egypt.[202][203]
In 1998, a team from NASA's Johnson Space Center obtained a small sample for analysis. Researchers found preterrestrial aqueous alteration phases and objects[204] of the size and shape consistent with Earthly fossilized nanobacteria.Analysis with gas chromatography and mass spectrometry (GC-MS) studied its high molecular weight polycyclic aromatic hydrocarbons in 2000, and NASA scientists concluded that as much as 75% of the organic compounds in Nakhla "may not be recent terrestrial contamination".[199][205]
This caused additional interest in this meteorite, so in 2006, NASA managed to obtain an additional and larger sample from the London Natural History Museum. On this second sample, a large dendritic carbon content was observed. When the results and evidence were published in 2006, some independent researchers claimed that the carbon deposits are of biologic origin. It was remarked that since carbon is the fourth most abundant element in the Universe, finding it in curious patterns is not indicative or suggestive of biological origin.[206][207]
Shergotty
[edit]The Shergotty meteorite, a 4 kilograms (8.8 lb) Martian meteorite, fell on Earth on Shergotty, India on August 25, 1865, and was retrieved by witnesses almost immediately.[208] It is composed mostly of pyroxene and thought to have undergone preterrestrial aqueous alteration for several centuries. Certain features in its interior suggest remnants of a biofilm and its associated microbial communities.[199]
Yamato 000593
[edit]Yamato 000593 is the second largest meteorite from Mars found on Earth. Studies suggest the Martian meteorite was formed about 1.3 billion years ago from a lava flow on Mars. An impact occurred on Mars about 12 million years ago and ejected the meteorite from the Martian surface into space. The meteorite landed on Earth in Antarctica about 50,000 years ago. The mass of the meteorite is 13.7 kg (30 lb) and it has been found to contain evidence of past water movement.[209][210][211] At a microscopic level, spheres are found in the meteorite that are rich in carbon compared to surrounding areas that lack such spheres. The carbon-rich spheres may have been formed by biotic activity according to NASA scientists.[209][210][211]
Ichnofossil-like structures
[edit]Organism–substrate interactions and their products are important biosignatures on Earth as they represent direct evidence of biological behaviour.[212] It was the recovery of fossilized products of life-substrate interactions (ichnofossils) that has revealed biological activities in the early history of life on the Earth, e.g., Proterozoic burrows, Archean microborings and stromatolites.[213][214][215][216][217][218] Two major ichnofossil-like structures have been reported from Mars, i.e. the stick-like structures from Vera Rubin Ridge and the microtunnels from Martian Meteorites.
Observations at Vera Rubin Ridge by the Mars Space Laboratory rover Curiosity show millimetric, elongate structures preserved in sedimentary rocks deposited in fluvio-lacustrine environments within Gale Crater. Morphometric and topologic data are unique to the stick-like structures among Martian geological features and show that ichnofossils are among the closest morphological analogues of these unique features.[219] Nevertheless, available data cannot fully disprove two major abiotic hypotheses, that are sedimentary cracking and evaporitic crystal growth as genetic processes for the structures.
Microtunnels have been described from Martian meteorites. They consist of straight to curved microtunnels that may contain areas of enhanced carbon abundance. The morphology of the curved microtunnels is consistent with biogenic traces on Earth, including microbioerosion traces observed in basaltic glasses.[220][221][218] Further studies are needed to confirm biogenicity.
Geysers
[edit]The seasonal frosting and defrosting of the southern ice cap results in the formation of spider-like radial channels carved on 1-meter thick ice by sunlight. Then, sublimed CO2 – and probably water – increase pressure in their interior producing geyser-like eruptions of cold fluids often mixed with dark basaltic sand or mud.[222][223][224][225] This process is rapid, observed happening in the space of a few days, weeks or months, a growth rate rather unusual in geology – especially for Mars.[226]
A team of Hungarian scientists propose that the geysers' most visible features, dark dune spots and spider channels, may be colonies of photosynthetic Martian microorganisms, which over-winter beneath the ice cap, and as the sunlight returns to the pole during early spring, light penetrates the ice, the microorganisms photosynthesize and heat their immediate surroundings. A pocket of liquid water, which would normally evaporate instantly in the thin Martian atmosphere, is trapped around them by the overlying ice. As this ice layer thins, the microorganisms show through grey. When the layer has completely melted, the microorganisms rapidly desiccate and turn black, surrounded by a grey aureole.[227][228][229] The Hungarian scientists believe that even a complex sublimation process is insufficient to explain the formation and evolution of the dark dune spots in space and time.[230][231] Since their discovery, fiction writer Arthur C. Clarke promoted these formations as deserving of study from an astrobiological perspective.[232]
A multinational European team suggests that if liquid water is present in the spiders' channels during their annual defrost cycle, they might provide a niche where certain microscopic life forms could have retreated and adapted while sheltered from solar radiation.[233] A British team also considers the possibility that organic matter, microbes, or even simple plants might co-exist with these inorganic formations, especially if the mechanism includes liquid water and a geothermal energy source.[226] They also remark that the majority of geological structures may be accounted for without invoking any organic "life on Mars" hypothesis.[226] It has been proposed to develop the Mars Geyser Hopper lander to study the geysers up close.[234]
Forward contamination
[edit]Planetary protection of Mars aims to prevent biological contamination of the planet.[235] A major goal is to preserve the planetary record of natural processes by preventing human-caused microbial introductions, also called forward contamination. There is abundant evidence as to what can happen when organisms from regions on Earth that have been isolated from one another for significant periods of time are introduced into each other's environment. Species that are constrained in one environment can thrive – often out of control – in another environment much to the detriment of the original species that were present. In some ways, this problem could be compounded if life forms from one planet were introduced into the totally alien ecology of another world.[236]
The prime concern of hardware contaminating Mars derives from incomplete spacecraft sterilization of some hardy terrestrial bacteria (extremophiles) despite best efforts.[28][237] Hardware includes landers, crashed probes, end-of-mission disposal of hardware, and the hard landing of entry, descent, and landing systems. This has prompted research on survival rates of radiation-resistant microorganisms including the species Deinococcus radiodurans and genera Brevundimonas, Rhodococcus, and Pseudomonas under simulated Martian conditions.[238] Results from one of these experimental irradiation experiments, combined with previous radiation modeling, indicate that Brevundimonas sp. MV.7 emplaced only 30 cm deep in Martian dust could survive the cosmic radiation for up to 100,000 years before suffering 106 population reduction.[238] The diurnal Mars-like cycles in temperature and relative humidity affected the viability of Deinococcus radiodurans cells quite severely.[239] In other simulations, Deinococcus radiodurans also failed to grow under low atmospheric pressure, under 0 °C, or in the absence of oxygen.[240]
Survival under simulated Martian conditions
[edit]Since the 1950s, researchers have used containers that simulate environmental conditions on Mars to determine the viability of a variety of lifeforms on Mars. Such devices, called "Mars jars" or "Mars simulation chambers", were first described and used in U.S. Air Force research in the 1950s by Hubertus Strughold, and popularized in civilian research by Joshua Lederberg and Carl Sagan.[241]
On April 26, 2012, scientists reported that an extremophile lichen survived and showed remarkable results on the adaptation capacity of photosynthetic activity within the simulation time of 34 days under Martian conditions in the Mars Simulation Laboratory (MSL) maintained by the German Aerospace Center (DLR).[242][243][244][245][246][247] The ability to survive in an environment is not the same as the ability to thrive, reproduce, and evolve in that same environment, necessitating further study.[29][28]
Although numerous studies point to resistance to some of Mars conditions, they do so separately, and none has considered the full range of Martian surface conditions, including temperature, pressure, atmospheric composition, radiation, humidity, oxidizing regolith, and others, all at the same time and in combination.[248] Laboratory simulations show that whenever multiple lethal factors are combined, the survival rates plummet quickly.[29]
Water salinity and temperature
[edit]Astrobiologists funded by NASA are researching the limits of microbial life in solutions with high salt concentrations at low temperature.[249] Any body of liquid water under the polar ice caps or underground is likely to exist under high hydrostatic pressure and have a significant salt concentration. They know that the landing site of Phoenix lander was found to be regolith cemented with water ice and salts, and the soil samples likely contained magnesium sulfate, magnesium perchlorate, sodium perchlorate, potassium perchlorate, sodium chloride and calcium carbonate.[249][250][251] Earth bacteria capable of growth and reproduction in the presence of highly salted solutions, called halophile or "salt-lover", were tested for survival using salts commonly found on Mars and at decreasing temperatures.[249] The species tested include Halomonas, Marinococcus, Nesterenkonia, and Virgibacillus.[249] Laboratory simulations show that whenever multiple Martian environmental factors are combined, the survival rates plummet quickly,[29] however, halophile bacteria were grown in a lab in water solutions containing more than 25% of salts common on Mars, and starting in 2019[needs update], the experiments will incorporate exposure to low temperature, salts, and high pressure.[249]
Mars-like regions on Earth
[edit]On 21 February 2023, scientists reported the findings of a "dark microbiome" of unfamiliar microorganisms in the Atacama Desert in Chile, a Mars-like region of Earth.[252][253]
Missions
[edit]Mars-2
[edit]Mars-1 was the first spacecraft launched to Mars in 1962,[254] but communication was lost while en route to Mars. With Mars-2 and Mars-3 in 1971–1972, information was obtained on the nature of the surface rocks and altitude profiles of the surface density of the soil, its thermal conductivity, and thermal anomalies detected on the surface of Mars. The program found that its northern polar cap has a temperature below −110 °C (−166 °F) and that the water vapor content in the atmosphere of Mars is five thousand times less than on Earth. No signs of life were found.[255]
Signs of life of the Mars space program AMS from orbit were not found. The descent vehicle Mars-2 crashed on landing, the descent vehicle Mars-3 launched 1.5 minutes after landing in the Ptolemaeus crater, but worked only 14.5 seconds/[256]
Mariner 4
[edit]Mariner 4 probe performed the first successful flyby of the planet Mars, returning the first pictures of the Martian surface in 1965. The photographs showed an arid Mars without rivers, oceans, or any signs of life. Further, it revealed that the surface (at least the parts that it photographed) was covered in craters, indicating a lack of plate tectonics and weathering of any kind for the last 4 billion years. The probe also found that Mars has no global magnetic field that would protect the planet from potentially life-threatening cosmic rays. The probe was able to calculate the atmospheric pressure on the planet to be about 0.6 kPa (compared to Earth's 101.3 kPa), meaning that liquid water could not exist on the planet's surface.[24] After Mariner 4, the search for life on Mars changed to a search for bacteria-like living organisms rather than for multicellular organisms, as the environment was clearly too harsh for these.[24][257][258]
Viking orbiters
[edit]Liquid water is necessary for known life and metabolism, so if water was present on Mars, the chances of it having supported life may have been determinant. The Viking orbiters found evidence of possible river valleys in many areas, erosion and, in the southern hemisphere, branched streams.[259][260][261]
Viking biological experiments
[edit]The primary mission of the Viking probes of the mid-1970s was to carry out experiments designed to detect microorganisms in Martian soil because the favorable conditions for the evolution of multicellular organisms ceased some four billion years ago on Mars.[262] The tests were formulated to look for microbial life similar to that found on Earth. Of the four experiments, only the Labeled Release (LR) experiment returned a positive result,[dubious – discuss] showing increased 14CO2 production on first exposure of soil to water and nutrients. All scientists agree on two points from the Viking missions: that radiolabeled 14CO2 was evolved in the Labeled Release experiment, and that the GCMS detected no organic molecules. There are vastly different interpretations of what those results imply: A 2011 astrobiology textbook notes that the GCMS was the decisive factor due to which "For most of the Viking scientists, the final conclusion was that the Viking missions failed to detect life in the Martian soil."[263]
Norman Horowitz was the head of the Jet Propulsion Laboratory bioscience section for the Mariner and Viking missions from 1965 to 1976. Horowitz considered that the great versatility of the carbon atom makes it the element most likely to provide solutions, even exotic solutions, to the problems of survival of life on other planets.[264] However, he also considered that the conditions found on Mars were incompatible with carbon based life.
One of the designers of the Labeled Release experiment, Gilbert Levin, believes his results are a definitive diagnostic for life on Mars.[24] Levin's interpretation is disputed by many scientists.[265] A 2006 astrobiology textbook noted that "With unsterilized Terrestrial samples, though, the addition of more nutrients after the initial incubation would then produce still more radioactive gas as the dormant bacteria sprang into action to consume the new dose of food. This was not true of the Martian soil; on Mars, the second and third nutrient injections did not produce any further release of labeled gas."[266] Other scientists argue that superoxides in the soil could have produced this effect without life being present.[267] An almost general consensus discarded the Labeled Release data as evidence of life, because the gas chromatograph and mass spectrometer, designed to identify natural organic matter, did not detect organic molecules.[185] More recently, high levels of organic chemicals, particularly chlorobenzene, were detected in powder drilled from one of the rocks, named "Cumberland", analyzed by the Curiosity rover.[268][269] The results of the Viking mission concerning life are considered by the general expert community as inconclusive.[24][267][270]
In 2007, during a Seminar of the Geophysical Laboratory of the Carnegie Institution (Washington, D.C., US), Gilbert Levin's investigation was assessed once more.[185] Levin still maintains that his original data were correct, as the positive and negative control experiments were in order.[271] Moreover, Levin's team, on April 12, 2012, reported a statistical speculation, based on old data—reinterpreted mathematically through cluster analysis—of the Labeled Release experiments, that may suggest evidence of "extant microbial life on Mars".[271][272] Critics counter that the method has not yet been proven effective for differentiating between biological and non-biological processes on Earth so it is premature to draw any conclusions.[273]
A research team from the National Autonomous University of Mexico headed by Rafael Navarro-González concluded that the GCMS equipment (TV-GC-MS) used by the Viking program to search for organic molecules, may not be sensitive enough to detect low levels of organics.[192] Klaus Biemann, the principal investigator of the GCMS experiment on Viking wrote a rebuttal.[274] Because of the simplicity of sample handling, TV–GC–MS is still considered the standard method for organic detection on future Mars missions, so Navarro-González suggests that the design of future organic instruments for Mars should include other methods of detection.[192]
After the discovery of perchlorates on Mars by the Phoenix lander, practically the same team of Navarro-González published a paper arguing that the Viking GCMS results were compromised by the presence of perchlorates.[275] A 2011 astrobiology textbook notes that "while perchlorate is too poor an oxidizer to reproduce the LR results (under the conditions of that experiment perchlorate does not oxidize organics), it does oxidize, and thus destroy, organics at the higher temperatures used in the Viking GCMS experiment."[276] Biemann has written a commentary critical of this Navarro-González paper as well,[277] to which the latter have replied;[278] the exchange was published in December 2011.
Phoenix lander, 2008
[edit]
The Phoenix mission landed a robotic spacecraft in the polar region of Mars on May 25, 2008, and it operated until November 10, 2008. One of the mission's two primary objectives was to search for a "habitable zone" in the Martian regolith where microbial life could exist, the other main goal being to study the geological history of water on Mars. The lander has a 2.5 meter robotic arm that was capable of digging shallow trenches in the regolith. There was an electrochemistry experiment which analysed the ions in the regolith and the amount and type of antioxidants on Mars. The Viking program data indicate that oxidants on Mars may vary with latitude, noting that Viking 2 saw fewer oxidants than Viking 1 in its more northerly position. Phoenix landed further north still.[279]Phoenix's preliminary data revealed that Mars soil contains perchlorate, and thus may not be as life-friendly as thought earlier.[280][281][194] The pH and salinity level were viewed as benign from the standpoint of biology. The analysers also indicated the presence of bound water and CO2.[282] A recent analysis of Martian meteorite EETA79001 found 0.6 ppm ClO4−, 1.4 ppm ClO3−, and 16 ppm NO3−, most likely of Martian origin. The ClO3− suggests presence of other highly oxidizing oxychlorines such as ClO2− or ClO, produced both by UV oxidation of Cl and X-ray radiolysis of ClO4−. Thus only highly refractory and/or well-protected (sub-surface) organics are likely to survive.[283] In addition, recent analysis of the Phoenix WCL showed that the Ca(ClO4)2 in the Phoenix soil has not interacted with liquid water of any form, perhaps for as long as 600 Myr. If it had, the highly soluble Ca(ClO4)2 in contact with liquid water would have formed only CaSO4. This suggests a severely arid environment, with minimal or no liquid water interaction.[284]
Mars Science Laboratory
[edit]
The Mars Science Laboratory mission is a NASA project that launched on November 26, 2011, the Curiosity rover, a nuclear-powered robotic vehicle, bearing instruments designed to assess past and present habitability conditions on Mars.[285][286] The Curiosity rover landed on Mars on Aeolis Palus in Gale Crater, near Aeolis Mons (a.k.a. Mount Sharp),[287][288][289][290] on August 6, 2012.[291][292][293]
On December 16, 2014, NASA reported the Curiosity rover detected a "tenfold spike", likely localized, in the amount of methane in the Martian atmosphere. Sample measurements taken "a dozen times over 20 months" showed increases in late 2013 and early 2014, averaging "7 parts of methane per billion in the atmosphere". Before and after that, readings averaged around one-tenth that level.[268][269] In addition, low levels of chlorobenzene (C
6H
5Cl), were detected in powder drilled from one of the rocks, named "Cumberland", analyzed by the Curiosity rover.[268][269]
- Methane measurements in the atmosphere of Mars
by the Curiosity rover (August 2012 to September 2014). - Methane (CH4) on Mars – potential sources and sinks.
- Comparison of organic compounds in Martian rocks – chlorobenzene levels were much higher in the "Cumberland" rock sample.
- Detection of organic compounds in the "Cumberland" rock sample.
- Sample analysis at Mars (SAM) of "Cumberland" rock.[294]
Mars 2020
[edit]The NASA Mars 2020 mission includes the Perseverance rover. Launched on July 30, 2020 it is intended to investigate an astrobiologically relevant ancient environment on Mars. This includes its surface geological processes and history, and an assessment of its past habitability and the potential for preservation of biosignatures within accessible geological materials.[295] Perseverance has been on Mars for 3 years, 169 days.
The Cheyava Falls rock discovered on Mars in June 2024 has been designated by NASA as a "potential biosignature" and was core sampled by the Perseverance rover for possible return to Earth and further examination. Although highly intriguing, no definitive final determination on a biological or abiotic origin of this rock can be made with the data currently available.
Future astrobiology missions
[edit]- ExoMars is a European-led multi-spacecraft programme currently under development by the European Space Agency (ESA) and the Roscosmos for launch in 2016 and 2020.[296] Its primary scientific mission will be to search for possible biosignatures on Mars, past or present. A rover with a 2 m (6.6 ft) core drill will be used to sample various depths beneath the surface where liquid water may be found and where microorganisms or organic biosignatures might survive cosmic radiation.[45] The program was suspended in 2022, and is unlikely to launch before 2028.[297]
- Mars sample-return mission – The best life detection experiment proposed is the examination on Earth of a soil sample from Mars. However, the difficulty of providing and maintaining life support over the months of transit from Mars to Earth remains to be solved. Providing for still unknown environmental and nutritional requirements is daunting, so it was concluded that "investigating carbon-based organic compounds would be one of the more fruitful approaches for seeking potential signs of life in returned samples as opposed to culture-based approaches."[298]
Human colonization of Mars
[edit]Some of the main reasons for colonizing Mars include economic interests, long-term scientific research best carried out by humans as opposed to robotic probes, and sheer curiosity. Surface conditions and the presence of water on Mars make it arguably the most hospitable of the planets in the Solar System, other than Earth. Human colonization of Mars would require in situ resource utilization (ISRU); A NASA report states that "applicable frontier technologies include robotics, machine intelligence, nanotechnology, synthetic biology, 3-D printing/additive manufacturing, and autonomy. These technologies combined with the vast natural resources should enable, pre- and post-human arrival ISRU to greatly increase reliability and safety and reduce cost for human colonization of Mars."[299][300][301]
Interactive Mars map
[edit]
See also
[edit]- Areography (geography of Mars)
- Astrobotany – Study of plants grown in spacecraft
- Carbonates on Mars
- Chemical gardening – Demonstration of metallic salts crystallization
- Chloride-bearing deposits on Mars
- Circumstellar habitable zone – Orbits where planets may have liquid surface water
- Composition of Mars
- Elysium Planitia
- Extraterrestrial life – Life not on Earth
- Fretted terrain
- Geology of Mars
- Glaciers on Mars
- Gravity of Mars
- Groundwater on Mars
- Hecates Tholus
- Hypothetical types of biochemistry – Possible alternative biochemicals used by life forms
- Lakes on Mars
- List of quadrangles on Mars
- List of rocks on Mars
- Magnetic field of Mars
- Mars Geyser Hopper
- Mars habitability analogue environments on Earth
- Mars in fiction § Life on Mars
- Martian craters
- Martian dichotomy
- Martian geyser
- Martian gullies
- Martian soil
- Mineralogy of Mars
- Ore resources on Mars
- Scientific information from the Mars Exploration Rover mission
- Seasonal flows on warm Martian slopes
- Terraforming of Mars – Hypothetical modification of Mars into a habitable planet
- Vallis
- Water on Mars
References
[edit]- ^ Ferreira, Becky (July 24, 2020). "3 Great Mysteries About Life on Mars - How habitable was early Mars? Why did it become less hospitable? And could there be life there now?". The New York Times. Retrieved July 24, 2020.
- ^ Chang, Kenneth (September 12, 2016). "Visions of Life on Mars in Earth's Depths". Financial Times. Archived from the original on September 12, 2016. Retrieved September 12, 2016.
- ^ Mumma, Michael J. (January 8, 2012). The Search for Life on Mars. Origin of Life Gordon Research Conference. Galveston, TX. Archived from the original on June 4, 2016.
- ^ Moser, D. E.; Arcuri, G. A.; Reinhard, D. A.; White, L. F.; Darling, J. R.; Barker, I. R.; Larson, D. J.; Irving, A. J.; McCubbin, F. M.; Tait, K. T.; Roszjar, J.; Wittmann, A.; Davis, C. (2019). "Decline of giant impacts on Mars by 4.48 billion years ago and an early opportunity for habitability". Nature Geoscience. 12 (7): 522–527. Bibcode:2019NatGe..12..522M. doi:10.1038/s41561-019-0380-0.
- ^ McKay, Christopher P.; Stoker, Carol R. (1989). "The early environment and its evolution on Mars: Implication for life". Reviews of Geophysics (Submitted manuscript). 27 (2): 189–214. Bibcode:1989RvGeo..27..189M. doi:10.1029/RG027i002p00189.
- ^ Gaidos, Eric; Selsis, Franck (2007). "From Protoplanets to Protolife: The Emergence and Maintenance of Life". Protostars and Planets V: 929–44. arXiv:astro-ph/0602008. Bibcode:2007prpl.conf..929G.
- ^ Grotzinger, John P. (January 24, 2014). "Introduction to Special Issue - Habitability, Taphonomy, and the Search for Organic Carbon on Mars". Science. 343 (6169): 386–387. Bibcode:2014Sci...343..386G. doi:10.1126/science.1249944. PMID 24458635.
- ^ Various (January 24, 2014). "Special Issue - Table of Contents - Exploring Martian Habitability". Science. 343 (6169): 345–452. Archived from the original on January 29, 2014.
- ^ Various (January 24, 2014). "Special Collection - Curiosity - Exploring Martian Habitability". Science. Archived from the original on January 28, 2014.
- ^ Grotzinger, J. P.; Sumner, D. Y.; Kah, L. C.; Stack, K.; Gupta, S.; Edgar, L.; Rubin, D.; Lewis, K.; Schieber, J.; et al. (January 24, 2014). "A Habitable Fluvio-Lacustrine Environment at Yellowknife Bay, Gale Crater, Mars". Science. 343 (6169): 1242777. Bibcode:2014Sci...343A.386G. CiteSeerX 10.1.1.455.3973. doi:10.1126/science.1242777. PMID 24324272. S2CID 52836398.
- ^ Gasda, Patrick J.; et al. (September 5, 2017). "In situ detection of boron by ChemCam on Mars" (PDF). Geophysical Research Letters. 44 (17): 8739–8748. Bibcode:2017GeoRL..44.8739G. doi:10.1002/2017GL074480.
- ^ Paoletta, Rae (September 6, 2017). "Curiosity Has Discovered Something That Raises More Questions About Life on Mars". Gizmodo. Archived from the original on September 6, 2017. Retrieved September 6, 2017.
- ^ Daley, Jason (July 6, 2017). "Mars Surface May Be Too Toxic for Microbial Life - The combination of UV radiation and perchlorates common on Mars could be deadly for bacteria". Smithsonian. Archived from the original on July 9, 2017. Retrieved July 8, 2017.
- ^ Wadsworth, Jennifer; Cockell, Charles S. (July 6, 2017). "Perchlorates on Mars enhance the bacteriocidal effects of UV light". Scientific Reports. 7 (4662): 4662. Bibcode:2017NatSR...7.4662W. doi:10.1038/s41598-017-04910-3. PMC 5500590. PMID 28684729.
- ^ Jump up to: a b Brown, Dwayne; Wendel, JoAnna; Steigerwald, Bill; Jones, Nancy; Good, Andrew (June 7, 2018). "Release 18-050 - NASA Finds Ancient Organic Material, Mysterious Methane on Mars". NASA. Archived from the original on June 7, 2018. Retrieved June 7, 2018.
- ^ Chang, Kenneth; Stirone, Shannon (February 8, 2021). "Life on Venus? The Picture Gets Cloudier - Despite doubts from many scientists, a team of researchers who said they had detected an unusual gas in the planet's atmosphere were still confident of their findings". The New York Times. Retrieved February 8, 2021.
- ^ Basalla, George (2006). Civilized life in the universe: scientists on intelligent extraterrestrials. New York: Oxford University Press. p. 52. ISBN 9780195171815.
- ^ mars.nasa.gov. "1800s | Mars Exploration Program". mars.nasa.gov. Archived from the original on January 10, 2019. Retrieved March 23, 2018.
- ^ Dunlap, David W. (October 1, 2015). "Life on Mars? You Read It Here First". New York Times. Archived from the original on October 1, 2015. Retrieved October 1, 2015.
- ^ Wallace, Alfred Russel (1907). Is Mars habitable?: A critical examination of Professor Percival Lowell's book 'Mars and its canals,' with an alternative explanation. London: Macmillan. OCLC 263175453.[page needed]
- ^ Philip Ball, "What the War of the Worlds means now". July 18, 2018. New Statesman (America Edition) July 18, 2018
- ^ Wallace, Alfred R. (1907). Is Mars Habitable? A Critical Examination of Professor Percival Lowell’s Book 'Mars and its Canals,' with an Alternative Explanation, p. 110, Macmillan.
- ^ Smith, Charles H. (2018). Is Mars Habitable? (S730: 1907). The Alfred Russel Wallace Page. Western Kentucky University. Retrieved August 26, 2023.
- ^ Jump up to: a b c d e f g Chambers, Paul (1999). Life on Mars; The Complete Story. London: Bland ford. ISBN 978-0-7137-2747-0.[page needed]
- ^ Dollfus, A. (2010) "The first Pic du Midi photographs of Mars, 1909" [1]
- ^ Jump up to: a b c Conrad, P. G.; Archer, D.; Coll, P.; De La Torre, M.; Edgett, K.; Eigenbrode, J. L.; Fisk, M.; Freissenet, C.; Franz, H.; et al. (2013). "Habitability Assessment at Gale Crater: Implications from Initial Results". 44th Lunar and Planetary Science Conference. 1719 (1719): 2185. Bibcode:2013LPI....44.2185C.
- ^ Schuerger, Andrew C.; Golden, D. C.; Ming, Doug W. (2012). "Biotoxicity of Mars soils: 1. Dry deposition of analog soils on microbial colonies and survival under Martian conditions". Planetary and Space Science. 72 (1): 91–101. Bibcode:2012P&SS...72...91S. doi:10.1016/j.pss.2012.07.026.
- ^ Jump up to: a b c d MEPAG Special Regions-Science Analysis Group; Beaty, D.; Buxbaum, K.; Meyer, M.; Barlow, N.; Boynton, W.; Clark, B.; Deming, J.; Doran, P. T.; et al. (2006). "Findings of the Mars Special Regions Science Analysis Group". Astrobiology. 6 (5): 677–732. Bibcode:2006AsBio...6..677M. doi:10.1089/ast.2006.6.677. PMID 17067257.
- ^ Jump up to: a b c d e Q. Choi, Charles (May 17, 2010). "Mars Contamination Dust-Up". Astrobiology Magazine. Archived from the original on August 20, 2011.
Whenever multiple biocidal factors are combined, the survival rates plummet quickly,
{{cite web}}
: CS1 maint: unfit URL (link) - ^ "Mars rover detects carbon signature that hints at past life source". AAAS Articles DO Group. January 17, 2022. doi:10.1126/science.ada0209. Retrieved November 14, 2023.
- ^ Fairén, A. G. (2010). "A cold and wet Mars Mars". Icarus. 208 (1): 165–175. Bibcode:2010Icar..208..165F. doi:10.1016/j.icarus.2010.01.006.
- ^ Fairén, A. G.; et al. (2009). "Stability against freezing of aqueous solutions on early Mars". Nature. 459 (7245): 401–404. Bibcode:2009Natur.459..401F. doi:10.1038/nature07978. PMID 19458717. S2CID 205216655.
- ^ Fairén, A. G.; et al. (2011). "Cold glacial oceans would have inhibited phyllosilicate sedimentation on early Mars". Nature Geoscience. 4 (10): 667–670. Bibcode:2011NatGe...4..667F. doi:10.1038/ngeo1243.
- ^ Jump up to: a b c d e Westall, Frances; Loizeau, Damien; Foucher, Frederic; Bost, Nicolas; Betrand, Marylene; Vago, Jorge; Kminek, Gerhard (2013). "Habitability on Mars from a Microbial Point of View". Astrobiology. 13 (18): 887–897. Bibcode:2013AsBio..13..887W. doi:10.1089/ast.2013.1000. PMID 24015806. S2CID 14117893.
- ^ "New Instrument Could Spy Signs of Alien Life in Glowing Rocks". Scientific American. July 27, 2022.
- ^ Staff (June 8, 2015). "PIA19673: Spectral Signals Indicating Impact Glass on Mars". NASA. Archived from the original on June 12, 2015. Retrieved June 8, 2015.
- ^ Jump up to: a b Summons, Roger E.; Amend, Jan P.; Bish, David; Buick, Roger; Cody, George D.; Des Marais, David J.; Dromart, Gilles; Eigenbrode, Jennifer L.; et al. (2011). "Preservation of Martian Organic and Environmental Records: Final Report of the Mars Biosignature Working Group". Astrobiology (Submitted manuscript). 11 (2): 157–81. Bibcode:2011AsBio..11..157S. doi:10.1089/ast.2010.0506. hdl:1721.1/66519. PMID 21417945. S2CID 9963677.
There is general consensus that extant microbial life on Mars would probably exist (if at all) in the subsurface and at low abundance.
- ^ Dehant, V.; Lammer, H.; Kulikov, Y. N.; Grießmeier, J. -M.; Breuer, D.; Verhoeven, O.; Karatekin, Ö.; Hoolst, T.; et al. (2007). "Planetary Magnetic Dynamo Effect on Atmospheric Protection of Early Earth and Mars". Geology and Habitability of Terrestrial Planets. Space Sciences Series of ISSI. Vol. 24. pp. 279–300. doi:10.1007/978-0-387-74288-5_10. ISBN 978-0-387-74287-8.
- ^ Rover could discover life on Mars – here's what it would take to prove it Archived January 7, 2018, at the Wayback Machine. Claire Cousins, PhysOrg. January 5, 2018.
- ^ Jump up to: a b "NASA Rover Finds Conditions Once Suited for Ancient Life on Mars". NASA. March 12, 2013. Archived from the original on July 3, 2013.
- ^ Chang, Kenneth (December 9, 2013). "On Mars, an Ancient Lake and Perhaps Life". New York Times. Archived from the original on December 9, 2013.
- ^ Various (December 9, 2013). "Science - Special Collection - Curiosity Rover on Mars". Science. Archived from the original on January 28, 2014.
- ^ Нил-Джонс, Нэнси; О'Кэрролл, Синтия (12 октября 2005 г.). «Новая карта дает больше доказательств того, что Марс когда-то был похож на Землю» . Центр космических полетов Годдарда . НАСА. Архивировано из оригинала 14 сентября 2012 года.
- ^ «Марсианский интерьер: палеомагнетизм» . Марс Экспресс . Европейское космическое агентство. 4 января 2007 года. Архивировано из оригинала 24 марта 2012 года . Проверено 6 июня 2013 г.
- ^ Перейти обратно: а б Уолл, Майк (25 марта 2011 г.). «Вопросы и ответы с марсианским искателем жизни Крисом Карром» . Space.com . Архивировано из оригинала 3 июня 2013 года.
- ^ «Прибор Эймса помог определить первую обитаемую среду на Марсе и получил награду за изобретение» . Исследовательский центр Эймса . Космос Арт. 24 июня 2014. Архивировано из оригинала 12 августа 2014 года . Проверено 11 августа 2014 г.
- ^ Файрен, АГ; и др. (2010). «Астробиология на протяжении веков Марса: изучение земных аналогов для понимания обитаемости Марса». Астробиология . 10 (8): 821–843. Бибкод : 2010AsBio..10..821F . дои : 10.1089/ast.2009.0440 . ПМИД 21087162 .
- ^ Темминг, Мария. «Экзотическое стекло может помочь разгадать тайны Марса» . Научный американец . Архивировано из оригинала 15 июня 2015 года . Проверено 15 июня 2015 г.
- ^ Браун, Дуэйн; и др. (7 июня 2018 г.). «НАСА обнаружило на Марсе древний органический материал и загадочный метан» . НАСА. Архивировано из оригинала 8 июня 2018 года . Проверено 12 июня 2018 г.
- ^ Перейти обратно: а б Эйгенброде, Дженнифер Л.; и др. (8 июня 2018 г.). «Органическое вещество сохранилось в аргиллитах возрастом 3 миллиарда лет в кратере Гейла на Марсе» (PDF) . Наука . 360 (6393): 1096–1101. Бибкод : 2018Sci...360.1096E . дои : 10.1126/science.aas9185 . hdl : 10044/1/60810 . ПМИД 29880683 . S2CID 46983230 .
- ^ Перейти обратно: а б Уолл, Майк (7 июня 2018 г.). «Ровер Curiosity нашел на Марсе древние «строительные блоки для жизни»» . Space.com . Архивировано из оригинала 7 июня 2018 года . Проверено 7 июня 2018 г.
- ^ Перейти обратно: а б Чанг, Кеннет (7 июня 2018 г.). «Жизнь на Марсе? Последнее открытие марсохода ставит ее «на стол» - Цитата: «Идентификация органических молекул в камнях на Красной планете не обязательно указывает на жизнь там, в прошлом или настоящем, но указывает на то, что некоторые здания блоки присутствовали." " . Нью-Йорк Таймс . Архивировано из оригинала 8 июня 2018 года . Проверено 8 июня 2018 г.
- ^ Перейти обратно: а б «Астробиологическая стратегия НАСА» (PDF) . НАСА . 2015. Архивировано из оригинала (PDF) 22 декабря 2016 года . Проверено 12 ноября 2017 г.
Подповерхностные слои: Вероятно, если жизнь существует (или существовала) на Марсе, ледяной луне или каком-либо другом планетарном теле, свидетельства этой жизни можно найти или лучше всего сохранить в недрах, вдали от современных суровых поверхностных процессов.
- ^ «Региональные, а не глобальные процессы привели к огромным марсианским наводнениям» . Институт планетарных наук . КосмическаяСсылка. 11 сентября 2015 года. Архивировано из оригинала 29 сентября 2015 года . Проверено 12 сентября 2015 г.
- ^ Якоски, Б.М.; Филлипс, Р.Дж. (2001). «История нестабильности и климата Марса» . Природа . 412 (6843): 237–244. Бибкод : 2001Natur.412..237J . дои : 10.1038/35084184 . ПМИД 11449285 .
- ^ Карр, Майкл Х. Поверхность Марса . Кембриджская серия планетарных наук (№ 6). ISBN 978-0-511-26688-1 .
- ^ Луманн, Дж.Г.; Рассел, Коннектикут (1997). «Марс: магнитное поле и магнитосфера» . В Ширли, Дж. Х.; Фейнбридж, RW (ред.). Энциклопедия планетарных наук . Нью-Йорк: Чепмен и Холл. стр. 454–6. Архивировано из оригинала 5 марта 2018 года . Проверено 5 марта 2018 г.
- ^ Филлипс, Тони (31 января 2001 г.). «Солнечный ветер на Марсе» . НАСА. Архивировано из оригинала 18 августа 2011 года.
- ^ «Что делает Марс таким враждебным для жизни?» . Новости Би-би-си . 7 января 2013 г. Архивировано из оригинала 30 августа 2013 г.
- ^ Джоанна Карвер и Виктория Джаггард (21 ноября 2012 г.). «Марс безопасен от радиации, но путешествие туда — нет» . Новый учёный . Архивировано из оригинала 12 февраля 2017 года.
- ^ Дональд М. Хасслер; Кэри Зейтлин; Роберт Ф. Виммер-Швайнгрубер; Бент Эресманн; Скот Рафкин; Дженнифер Л. Эйгенброде; Дэвид Э. Бринза; Джеральд Вейгл; Стефан Бетчер; Эккарт Бём; Зенке Бурмейстер; Цзиннань Го; Ян Кёлер; Сезар Мартин; Гюнтер Райтц; Фрэнсис А. Кучинотта; Мён Хи Ким; Дэвид Гринспун; Марк А. Баллок; Арик Познер; Хавьер Гомес-Эльвира; Ашвин Васавада; Джон П. Гротцингер; Научная группа MSL (12 ноября 2013 г.). «Радиационная обстановка на поверхности Марса, измеренная с помощью марсохода Curiosity Марсианской научной лаборатории» (PDF) . Наука . 343 (6169): 7. Бибкод : 2014Sci...343D.386H . дои : 10.1126/science.1244797 . hdl : 1874/309142 . ПМИД 24324275 . S2CID 33661472 . Архивировано (PDF) из оригинала 2 февраля 2014 г.
- ^ Дональд М. Хасслер; Кэри Зейтлин; Роберт Ф. Виммер-Швайнгрубер; Бент Эресманн; Скот Рафкин; Дженнифер Л. Эйгенброде; Дэвид Э. Бринза; Джеральд Вейгл; Стефан Бетчер; Эккарт Бём; Зенке Бурмейстер; Цзиннань Го; Ян Кёлер; Сезар Мартин; Гюнтер Райтц; Фрэнсис А. Кучинотта; Мён Хи Ким; Дэвид Гринспун; Марк А. Баллок; Арик Познер; Хавьер Гомес-Эльвира; Ашвин Васавада; Джон П. Гротцингер; Научная группа MSL (12 ноября 2013 г.). «Радиационная обстановка на поверхности Марса, измеренная с помощью марсохода Curiosity Марсианской научной лаборатории» (PDF) . Наука . 343 (6169): 8. Бибкод : 2014Sci...343D.386H . дои : 10.1126/science.1244797 . hdl : 1874/309142 . ПМИД 24324275 . S2CID 33661472 . Архивировано (PDF) из оригинала 2 февраля 2014 г.
- ^ Перейти обратно: а б Тан, Кер (29 января 2007 г.). «Исследование: поверхность Марса, лишенная жизни» . Space.com . Архивировано из оригинала 29 апреля 2014 года
. После составления карты уровней космического излучения на различных глубинах Марса исследователи пришли к выводу, что любая жизнь в пределах первых нескольких ярдов поверхности планеты будет уничтожена смертельными дозами космического излучения.
- ^ Дартнелл, Льюис Р.; Сторри-Сторри-Ломбарди, Майкл С.; Мюллер, Ян-Петер; Гриффитс, Эндрю. Д.; Коутс, Эндрю Дж.; Уорд, Джон М. (2011). «Влияние космического излучения на поверхность Марса для выживания микробов и обнаружения флуоресцентных биосигнатур» (PDF) . Лунно-планетарный институт . 42 (1608): 1977. Бибкод : 2011LPI....42.1977D . Архивировано (PDF) из оригинала 6 октября 2013 г.
- ^ Перейти обратно: а б Дартнелл, ЛР; Десоргер, Л.; Уорд, Дж. М.; Коутс, Эй Джей (2007). «Моделирование поверхностной и подземной радиационной среды Марса: значение для астробиологии» . Письма о геофизических исследованиях . 34 (2): L02207. Бибкод : 2007GeoRL..34.2207D . дои : 10.1029/2006GL027494 . S2CID 59046908 .
Бактерии или споры, находящиеся в состоянии покоя в условиях замерзания, не могут метаболизироваться и инактивироваться из-за накопления радиационного повреждения. Мы обнаружили, что на глубине 2 м, в зоне действия бура ЭкзоМарс, популяция радиорезистентных клеток должна была реанимироваться в течение последних 450 000 лет, чтобы оставаться жизнеспособной. Для извлечения жизнеспособных клеток, криоконсервированных в предполагаемом паковом льду Цербера, требуется бурение глубиной не менее 7,5 м.
- ^ Ловет, Ричард А. (2 февраля 2007 г.). «Жизнь на Марсе может находиться слишком глубоко, чтобы ее можно было найти, заключают эксперты» . Национальные географические новости . Архивировано из оригинала 21 февраля 2014 года.
Это связано с тем, что любые бактерии, которые когда-то могли жить на поверхности, уже давно уничтожены космическим излучением, проникающим сквозь тонкую марсианскую атмосферу.
- ^ Ловет, Ричард А. (2 февраля 2007 г.). «Жизнь на Марсе может находиться слишком глубоко, чтобы ее можно было найти, заключают эксперты» . Национальные географические новости . Архивировано из оригинала 21 февраля 2014 года.
- ^ Перейти обратно: а б с д и Хасслер, Дональд М.; Цейтлин, К; и др. (24 января 2014 г.). «Радиационная обстановка на поверхности Марса, измеренная с помощью марсохода Curiosity Марсианской научной лаборатории» (PDF) . Наука . 343 (6169): 1244797. Бибкод : 2014Sci...343D.386H . дои : 10.1126/science.1244797 . hdl : 1874/309142 . ПМИД 24324275 . S2CID 33661472 . Архивировано (PDF) из оригинала 2 февраля 2014 г.
- ^ Скотт, Джим (30 сентября 2017 г.). «Крупная солнечная буря вызывает глобальное сияние и удваивает уровень радиации на поверхности Марса» . Физика.орг . Архивировано из оригинала 30 сентября 2017 года . Проверено 30 сентября 2017 г.
- ^ Раммель, Джон Д.; Бити, Дэвид В.; Джонс, Мелисса А.; Бейкерманс, Кориен; Барлоу, Надин Г.; Бостон, Пенелопа Дж.; Шеврие, Винсент Ф.; Кларк, Бентон С.; де Вера, Жан-Пьер П.; Гоф, Райна В.; Холлсворт, Джон Э.; Руководитель Джеймс В.; Хипкин, Виктория Дж.; Кифт, Томас Л.; МакИвен, Альфред С.; Меллон, Майкл Т.; Микуки, Джилл А.; Николсон, Уэйн Л.; Омелон, Кристофер Р.; Петерсон, Рональд; Роден, Эрик Э.; Шервуд Лоллар, Барбара; Танака, Кеннет Л.; Виола, Донна; Рэй, Джеймс Дж. (2014). «Новый анализ «особых регионов» Марса: результаты второй группы научного анализа особых регионов MEPAG (SR-SAG2)» (PDF) . Астробиология . 14 (11): 887–968. Бибкод : 2014AsBio..14..887R . дои : 10.1089/ast.2014.1227 . ISSN 1531-1074 . ПМИД 25401393 . Архивировано (PDF) из оригинала 13 февраля 2017 г.
- ^ Перейти обратно: а б с д и Уодсворт, Дж; Кокелл, CS (2017). «Перхлораты на Марсе усиливают бактерицидное действие ультрафиолета» . Научный представитель . 7 (1): 4662. Бибкод : 2017NatSR...7.4662W . дои : 10.1038/s41598-017-04910-3 . ПМК 5500590 . ПМИД 28684729 .
- ^ Перейти обратно: а б с Эртем, Г.; Эртем, MC; Маккей, CP; Хазен, РМ (2017). «Защита биомолекул от воздействия радиации минералами-аналогами Марса и почвами». Международный журнал астробиологии . 16 (3): 280–285. Бибкод : 2017IJAsB..16..280E . дои : 10.1017/S1473550416000331 . S2CID 125294279 .
- ^ Мацубара, Тошитака; Фудзисима, Косукэ; Салтиков, Чад В.; Накамура, Сатоши ; Ротшильд, Линн Дж. (2017). «Земные аналоги прошлой и будущей жизни на Марсе: выделение устойчивых к перхлоратам галофилов из Большого Содового озера» . Международный журнал астробиологии . 16 (3): 218–228. Бибкод : 2017IJAsB..16..218M . дои : 10.1017/S1473550416000458 .
- ^ Перейти обратно: а б Хайнц, Джейкоб; Кран, Тим; Шульце-Макух, Дирк (28 апреля 2020 г.). «Новый рекорд микробной толерантности к перхлоратам: рост грибков в рассолах NaClO4 и его последствия для предполагаемой жизни на Марсе» . Жизнь . 10 (5): 53. Бибкод : 2020Жизнь...10...53Ч . дои : 10.3390/life10050053 . ISSN 2075-1729 . ПМК 7281446 . ПМИД 32353964 .
- ^ Хайнц, Джейкоб; Дёллингер, Йорг; Маус, Дебора; Шнайдер, Энди; Лэш, Питер; Гроссарт, Ганс-Петер; Шульце-Макух, Дирк (10 августа 2022 г.). «Специфические для перхлората протеомные стрессовые реакции Debaryomyces hansenii могут обеспечить выживание микробов в марсианских рассолах» . Экологическая микробиология . 24 (11): 1462–2920.16152. Бибкод : 2022EnvMi..24.5051H . дои : 10.1111/1462-2920.16152 . ISSN 1462-2912 . ПМИД 35920032 .
- ^ Аль Суди, Амер Ф.; Фархат, Омар; Чен, Фэй; Кларк, Бентон С.; Шнегурт, Марк А. (2017). «Толерантность роста бактерий к концентрациям хлората и перхлората, характерным для Марса» . Международный журнал астробиологии . 16 (3): 229–235. Бибкод : 2017IJAsB..16..229A . дои : 10.1017/S1473550416000434 .
- ^ Чанг, Кеннет (5 октября 2015 г.). «Марс довольно чистый. Ее работа в НАСА — поддерживать его таким» . Нью-Йорк Таймс . Нью-Йорк Таймс. Архивировано из оригинала 6 октября 2015 года.
- ^ Хайнц, Джейкоб; Вааен, Аннемик К.; Айро, Алессандро; Алибранди, Армандо; Ширмак, Янош; Шульце-Макух, Дирк (1 ноября 2019 г.). «Рост бактерий в хлоридных и перхлоратных рассолах: галотолерантность и реакция Planococcus halocryophilus на солевой стресс» . Астробиология . 19 (11): 1377–1387. Бибкод : 2019AsBio..19.1377H . дои : 10.1089/ast.2019.2069 . ISSN 1531-1074 . ПМК 6818489 . ПМИД 31386567 .
- ^ Бак, Эббе Н.; Ларсен, Майкл Г.; Мёллер, Ральф; Ниссен, Сайлас Б.; Дженсен, Лассе Р.; Норнберг, Пер; Йенсен, Свенд Дж.К.; Финстер, Кай (12 сентября 2017 г.). «Силикаты, разрушенные в моделируемых марсианских условиях, эффективно убивают бактерии — вызов для жизни на Марсе» . Границы микробиологии . 8 : 1709. дои : 10.3389/fmicb.2017.01709 . ПМК 5601068 . ПМИД 28955310 .
- ↑ Почему жизнь на Марсе может быть невозможной. Архивировано 7 сентября 2017 года в Wayback Machine . Джеффри Клюгер. Время – Наука; 6 июля 2017 г.
- ^ Перейти обратно: а б Марсианская почва может быть токсичной для микробов. Архивировано 11 сентября 2017 года в Wayback Machine . Майк Уолл. Space.com. 6 июля 2017 г.
- ^ Марсианская почва, вероятно, токсична для клеток — означает ли это, что люди не смогут выращивать там овощи? Архивировано 11 сентября 2017 года в Wayback Machine . Дэвид Коуди. Мир сегодня . 7 июля 2017 г.
- ^ Линч, Кеннда Л.; Джексон, В. Эндрю; Рей, Кевин; Спир, Джон Р.; Розенцвейг, Фрэнк; Мунаката-Марр, Джунко (1 марта 2019 г.). «Доказательства биотического восстановления перхлоратов в естественно богатых перхлоратами отложениях бассейна Пилот-Вэлли, штат Юта» . Астробиология . 19 (5): 629–641. Бибкод : 2019AsBio..19..629L . дои : 10.1089/ast.2018.1864 . ISSN 1531-1074 . ПМИД 30822097 . S2CID 73492950 .
- ↑ Чанг, Кеннет (28 июля 2020 г.). «Как НАСА нашело идеальную дыру на Марсе для посадки» . Нью-Йорк Таймс . ISSN 0362-4331. Проверено 02 марта 2021 г.
- ↑ Дэйнс, Гэри (14 августа 2020 г.). «В поисках жизни в древних озерах» (4 сезон, 15 серия). Гравитационный помощник.НАСА. Подкаст. Проверено 02 марта 2021 г.
- ^ Раммель, Джон Д.; Бити, Дэвид В.; Джонс, Мелисса А.; Бейкерманс, Кориен; Барлоу, Надин Г.; Бостон, Пенелопа Дж.; Шеврие, Винсент Ф.; Кларк, Бентон С.; де Вера, Жан-Пьер П.; Гоф, Райна В.; Холлсуорт, Джон Э.; Руководитель Джеймс В.; Хипкин, Виктория Дж.; Кифт, Томас Л.; МакИвен, Альфред С.; Меллон, Майкл Т.; Микуки, Джилл А.; Николсон, Уэйн Л.; Омелон, Кристофер Р.; Петерсон, Рональд; Роден, Эрик Э.; Шервуд Лоллар, Барбара; Танака, Кеннет Л.; Виола, Донна; Рэй, Джеймс Дж. (2014). «Новый анализ жидких «особых регионов»: результаты второй группы научного анализа особых регионов MEPAG (SR-SAG2)» (PDF) . Астробиология . 14 (11): 887–968. Бибкод : 2014AsBio..14..887R . дои : 10.1089/ast.2014.1227 . ISSN 1531-1074 . ПМИД 25401393 .
- ^ «Теплые сезонные потоки на склоне кратера Ньютона» . Пресс-релиз НАСА . 23 июля 2018 г. Архивировано из оригинала 12 февраля 2017 г.
- ^ Амос, Джонатан. «Марсианские соляные полосы, «нарисованные жидкой водой» » . Би-би-си Наука. Архивировано из оригинала 25 ноября 2016 года.
- ^ Персонал (28 сентября 2015 г.). «Основное видео — Пресс-конференция НАСА — Доказательства наличия жидкой воды на современном Марсе» . НАСА . Архивировано из оригинала 1 октября 2015 года . Проверено 30 сентября 2015 г.
- ^ Персонал (28 сентября 2015 г.). «Видео завершено — Пресс-конференция НАСА — Вода течет на современном Марсе м» . НАСА . Архивировано из оригинала 15 октября 2015 года . Проверено 30 сентября 2015 г.
- ^ Оджа, Л.; Вильгельм, МБ; Мурчи, СЛ; МакИвен, А.С.; Рэй, Джей-Джей; Хэнли, Дж.; Массе, М.; Хойнацкий, М. (2015). «Спектральные доказательства наличия гидратированных солей в повторяющихся наклонных линиях на Марсе». Природа Геонауки . 8 (11): 829–832. Бибкод : 2015NatGe...8..829O . дои : 10.1038/ngeo2546 .
- ^ Фокс-Пауэлл, Марк Г.; Холлсворт, Джон Э.; Казинс, Клэр Р.; Кокелл, Чарльз С. (2016). «Ионная сила является барьером для обитаемости Марса» (PDF) . Астробиология . 16 (6): 427–442. Бибкод : 2016AsBio..16..427F . дои : 10.1089/ast.2015.1432 . hdl : 10023/10912 . ПМИД 27213516 . S2CID 4314602 .
- ^ Маккей, Кристофер П.; Стокер, Кэрол Р.; Гласс, Брайан Дж.; Даве, Арвен И.; Давила, Альфонсо Ф.; Хелдманн, Дженнифер Л.; Маринова Маргарита М.; Файрен, Альберто Г.; Куинн, Ричард С.; и др. (5 апреля 2013 г.). « Миссия ледокола Life на Марс: поиск биомолекулярных доказательств существования жизни». Астробиология . 13 (4): 334–353. Бибкод : 2013AsBio..13..334M . дои : 10.1089/ast.2012.0878 . ПМИД 23560417 .
- ^ Перейти обратно: а б Стерн, Дженнифер С. (24 марта 2015 г.). «Доказательства наличия местного азота в осадочных и эоловых отложениях в результате исследований марсохода Curiosity в кратере Гейла на Марсе» . Труды Национальной академии наук Соединенных Штатов Америки . 112 (14): 4245–4250. Бибкод : 2015PNAS..112.4245S . дои : 10.1073/pnas.1420932112 . ПМЦ 4394254 . ПМИД 25831544 .
- ^ Нил-Джонс, Нэнси; Штайгервальд, Уильям; Вебстер, Гай; Браун, Дуэйн (24 марта 2015 г.). «Ровер Curiosity обнаружил на Марсе биологически полезный азот» . НАСА . Архивировано из оригинала 27 марта 2015 года . Проверено 25 марта 2015 г.
- ^ «Марсоход Curiosity обнаружил «полезный азот» » . НАСА . Новости Би-би-си. 25 марта 2015 года. Архивировано из оригинала 27 марта 2015 года . Проверено 25 марта 2015 г.
- ^ Перейти обратно: а б Азот на Марсе: выводы Curiosity (PDF) . Дж. К. Стерн, Б. Саттер, В. А. Джексон, Рафаэль Наварро-Гонсалес, Кристофер П. Маккей, Дуглас В. Минг, П. Дуглас Арчер, Д. П. Главин1, А. Г. Фэйрен иПол Р. Махаффи. Лунная и планетарная наука XLVIII (2017).
- ^ Бокс, CS; Рука, КП; Нилсон, К.Х.; Юнг, Ю.Л.; Саис-Лопес, А. (2012). «Активный цикл азота на Марсе, достаточный для поддержания подземной биосферы» (PDF) . Международный журнал астробиологии . 11 (2): 109–115. Бибкод : 2012IJAsB..11..109B . дои : 10.1017/S1473550411000401 . S2CID 40894966 .
- ^ Адкок, Коннектикут; Хаусрат, Э.М.; Форстер, премьер-министр (2013). «Легкодоступный фосфат из минералов в ранней водной среде Марса». Природа Геонауки . 6 (10): 824–827. Бибкод : 2013NatGe...6..824A . дои : 10.1038/ngeo1923 .
- ^ Перейти обратно: а б Шуергер, Эндрю К.; Ульрих, Ричард; Берри, Бонни Дж.; Николсон, Уэйн Л. (февраль 2013 г.). «Рост Serratia liquefaciens при давлении 7 мбар, 0 ° C и в аноксической атмосфере, обогащенной CO2» . Астробиология . 13 (2): 115–131. Бибкод : 2013AsBio..13..115S . дои : 10.1089/ast.2011.0811 . ПМЦ 3582281 . ПМИД 23289858 .
- ^ Хейс, Линда; и др. (октябрь 2015 г.). «Астробиологическая стратегия 2015» (PDF) . НАСА . Архивировано из оригинала (PDF) 22 декабря 2016 года . Проверено 21 сентября 2017 г.
- ^ Хелдманн, Дженнифер Л.; Тун, Оуэн Б.; Поллард, Уэйн Х.; Меллон, Майкл Т.; Питлик, Джон; Маккей, Кристофер П.; Андерсен, Дейл Т. (2005). «Образование марсианских оврагов под действием жидкой воды, текущей в современных условиях марсианской окружающей среды». Журнал геофизических исследований . 110 (Е5): E05004. Бибкод : 2005JGRE..110.5004H . дои : 10.1029/2004JE002261 . hdl : 2060/20050169988 . S2CID 1578727 .
- ^ Костама, В.-П.; Креславский, М.А.; Хед, JW (2006). «Современная высокоширотная ледяная мантия на северных равнинах Марса: характеристики и возраст размещения». Письма о геофизических исследованиях . 33 (11): 11201. Бибкод : 2006GeoRL..3311201K . CiteSeerX 10.1.1.553.1127 . дои : 10.1029/2006GL025946 . S2CID 17229252 .
- ^ Хехт, Майкл Х.; Васавада, Ашвин Р. (2006). «Переходная жидкая вода возле искусственного источника тепла на Марсе». Международный журнал науки и исследования Марса . 2 : 83–96. Бибкод : 2006IJMSE...2...83H . дои : 10.1555/mars.2006.0006 .
- ^ Сига, Дэвид (7 декабря 2009 г.). «Водная ниша может способствовать развитию жизни на Марсе» . Новый учёный . Архивировано из оригинала 7 октября 2013 года.
- ^ Виеру, Тудор (7 декабря 2009 г.). «Парниковый эффект на Марсе может способствовать возникновению жизни» . Софтпедия. Архивировано из оригинала 31 июля 2013 года.
- ^ Меллон, Майкл Т. (10 мая 2011 г.). «Подповерхностный лед на Марсе: обзор льда и воды в экваториальных регионах» (PDF) . Заседание подкомитета планетарной защиты . Университет Колорадо. Архивировано из оригинала (PDF) 28 февраля 2014 г.
- ^ Бритт, Роберт Рой (22 февраля 2005 г.). «Пакеты со льдом и метан на Марсе предполагают возможность существования современной жизни» . space.com . Архивировано из оригинала 3 мая 2013 года.
- ^ Меллон, Майкл Т.; Якоски, Брюс М.; Поставко, Сьюзен Э. (1997). «Стойкость экваториального подземного льда на Марсе» . Журнал геофизических исследований . 102 (Е8): 19357–69. Бибкод : 1997JGR...10219357M . дои : 10.1029/97JE01346 .
- ^ Арфстрем, доктор медицинских наук (2012). «Концептуальная модель экваториальных ледяных щитов Марса». Сравнительная климатология планет земной группы . 1675 : 8001. Бибкод : 2012LPICo1675.8001A .
- ^ Перейти обратно: а б Персонал (22 ноября 2016 г.). «Зубчатая местность привела к обнаружению погребенного льда на Марсе» . НАСА . Архивировано из оригинала 24 ноября 2016 года . Проверено 23 ноября 2016 г.
- ^ Перейти обратно: а б «Озеро замерзшей воды размером с Нью-Мексико обнаружено на Марсе – НАСА» . Регистр. 22 ноября 2016 года. Архивировано из оригинала 23 ноября 2016 года . Проверено 23 ноября 2016 г.
- ^ Перейти обратно: а б «Марсианские ледяные отложения содержат столько же воды, сколько озеро Верхнее» . НАСА. 22 ноября 2016 года. Архивировано из оригинала 23 ноября 2016 года . Проверено 23 ноября 2016 г.
- ^ «Марсианская Одиссея: Отдел новостей» . Mars.jpl.nasa.gov. 28 мая 2002 г. Архивировано из оригинала 6 июня 2011 г.
- ^ Фельдман, WC (2004). «Глобальное распространение приповерхностного водорода на Марсе» . Журнал геофизических исследований . 109 (Е9). Бибкод : 2004JGRE..109.9006F . дои : 10.1029/2003JE002160 .
- ^ «Mars Global Surveyor измеряет водные облака» . Архивировано из оригинала 12 августа 2009 года . Проверено 7 марта 2009 г.
- ^ Бейкер, VR; Стром, Р.Г.; Гулик, ВК; Каргель, Дж.С.; Комацу, Г.; Кале, В.С. (1991). «Древние океаны, ледниковые щиты и гидрологический цикл на Марсе». Природа . 352 (6336): 589–594. Бибкод : 1991Natur.352..589B . дои : 10.1038/352589a0 . S2CID 4321529 .
- ^ «Воспоминания: 10 лет назад было объявлено о наличии воды на Марсе» . SPACE.com. 22 июня 2000 г. Архивировано из оригинала 22 декабря 2010 г.
- ^ «Дело о пропавшей марсианской воде» . Наука@НАСА . Архивировано из оригинала 27 марта 2009 года . Проверено 7 марта 2009 г.
- ^ «Марсоход Opportunity исследует глиняные подсказки в скалах» . НАСА . Лаборатория реактивного движения. 17 мая 2013 г. Архивировано из оригинала 11 июня 2013 г.
- ^ «Ровер НАСА помогает раскрыть возможные тайны марсианской жизни» . НАСА . 29 ноября 2005 г. Архивировано из оригинала 22 ноября 2013 г.
- ^ «Картирование Марса: наука, воображение и рождение мира». Оливер Мортон, 2002. ISBN 0-312-24551-3 [ нужна страница ]
- ^ «PSRD: Древние паводки и моря на Марсе» . Psrd.hawaii.edu. 16 июля 2003 г. Архивировано из оригинала 4 января 2011 г.
- ^ «Данные гамма-излучения позволяют предположить, что на древнем Марсе были океаны» . КосмическаяСсылка. 17 ноября 2008 г.
- ^ Карр, Майкл Х.; Хед, Джеймс В. (2003). «Океаны на Марсе: оценка данных наблюдений и возможная судьба» . Журнал геофизических исследований: Планеты . 108 (E5): 5042. Бибкод : 2003JGRE..108.5042C . дои : 10.1029/2002JE001963 .
- ^ Харвуд, Уильям (25 января 2013 г.). «Марсоход «Оппортьюнити» отмечает 10-й год работы на Марсе» . Космический полет сейчас. Архивировано из оригинала 24 декабря 2013 года.
- ^ Ди Ахилле, Гаэтано; Хайнек, Брайан М. (2010). «Древний океан на Марсе поддерживается глобальным распределением дельт и долин». Природа Геонауки . 3 (7): 459–63. Бибкод : 2010NatGe...3..459D . дои : 10.1038/ngeo891 .
- «Древний океан мог покрывать треть Марса» . ScienceDaily (пресс-релиз). 14 июня 2010 г.
- ^ Смит, Делавэр; Шегрен, ВЛ; Тайлер, ГЛ; Бальмино, Г.; Лемуан, ФГ; Коноплив А.С. (1999). «Гравитационное поле Марса: результаты Mars Global Surveyor». Наука . 286 (5437): 94–7. Бибкод : 1999Sci...286...94S . дои : 10.1126/science.286.5437.94 . ПМИД 10506567 .
- ^ Тоска, Николас Дж.; Нолл, Эндрю Х.; МакЛеннан, Скотт М. (2008). «Активность воды и проблема жизни на раннем Марсе». Наука . 320 (5880): 1204–7. Бибкод : 2008Sci...320.1204T . дои : 10.1126/science.1155432 . ПМИД 18511686 . S2CID 27253871 .
- ^ ДасСарма, Шиладитья (2006). «Экстремальные галофилы — модели для астробиологии» . Микроб . 1 (3): 120–6. Архивировано из оригинала 22 июля 2011 года.
- ^ Малин, Майкл С.; Эджетт, Кеннет С. (2000). «Доказательства недавнего просачивания подземных вод и поверхностного стока на Марсе». Наука . 288 (5475): 2330–5. Бибкод : 2000Sci...288.2330M . дои : 10.1126/science.288.5475.2330 . ПМИД 10875910 .
- ^ Мартинес, генеральный менеджер; Ренно, НЕТ; Эллиотт, HM; Фишер, Э. (2013). Жидкая вода на Марсе в наши дни: теоретические ожидания, данные наблюдений и предпочтительные места (PDF) . Конференция по обитаемости современного Марса. Лос-Анджелес. Архивировано (PDF) из оригинала 25 февраля 2014 г.
- ^ Колб, К.; Пеллетье, Джон Д.; МакИвен, Альфред С. (2010). «Моделирование формирования ярких отложений на склонах, связанных с оврагами в кратере Хейл, Марс: последствия для современной жидкой воды». Икар . 205 (1): 113–137. Бибкод : 2010Icar..205..113K . дои : 10.1016/j.icarus.2009.09.009 .
- ^ "Пресс-релиз" . Университет Аризоны. 16 марта 2006 г. Архивировано из оригинала 21 июля 2006 г.
{{cite web}}
: CS1 maint: неподходящий URL ( ссылка ) - ^ Керр, Ричард (8 декабря 2006 г.). «Лебединая песня Марсианского орбитального аппарата: Красная планета меняется » . Наука . 314 (5805): 1528–1529. дои : 10.1126/science.314.5805.1528 . ПМИД 17158298 . S2CID 46381976 .
- ^ «НАСА обнаружило возможные признаки текущей воды на Марсе» . voanews.com. 3 августа 2011 г. Архивировано из оригинала 17 сентября 2011 г.
- ^ Исследовательский центр Эймса (6 июня 2009 г.). «Учёные НАСА нашли доказательства наличия жидкой воды на замёрзшем раннем Марсе» . КосмическаяСсылка.
- ^ «Мертвый космический корабль на Марсе жив в новом исследовании» . SPACE.com. 10 июня 2008 г. Архивировано из оригинала 24 ноября 2010 г.
- ^ МакИвен, Альфред С.; Оджа, Лухендра; Дандас, Колин М.; Мэттсон, Сара С.; Бирн, Шейн; Рэй, Джеймс Дж.; Калл, Селби К.; Мурчи, Скотт Л.; и др. (2011). «Сезонные потоки на теплых марсианских склонах». Наука . 333 (6043): 740–3. Бибкод : 2011Sci...333..740M . дои : 10.1126/science.1204816 . ПМИД 21817049 . S2CID 10460581 .
- ^ Перейти обратно: а б Оросей, Р.; и др. (25 июля 2018 г.). «Радиолокационные доказательства наличия подледной жидкой воды на Марсе» . Наука . 361 (6401): 490–493. arXiv : 2004.04587 . Бибкод : 2018Sci...361..490O . дои : 10.1126/science.aar7268 . hdl : 11573/1148029 . ПМИД 30045881 .
- ^ Чанг, Кеннет; Прощай, Деннис (25 июля 2018 г.). «На Марсе обнаружено водное озеро, повышающее потенциал инопланетной жизни. Это открытие предполагает, что водные условия под ледяной южной полярной шапкой, возможно, послужили одним из важнейших строительных блоков жизни на Красной планете» . Нью-Йорк Таймс . Архивировано из оригинала 25 июля 2018 года . Проверено 25 июля 2018 г.
- ^ «Под поверхностью Марса обнаружен огромный резервуар жидкой воды» . ЭврекАлерт . 25 июля 2018 года. Архивировано из оригинала 25 июля 2018 года . Проверено 25 июля 2018 г.
- ^ Хэлтон, Мэри (25 июля 2018 г.). «На Марсе обнаружено жидкое водное озеро» . Новости Би-би-си . Архивировано из оригинала 25 июля 2018 года . Проверено 25 июля 2018 г.
- ^ Дополнительные материалы для: Оросей, Р; Лауро, ЮВ; Комбс, Е; Чикетти, А; Корадини, М; Бедра, Б; Ди Поль, форвард; Фламини, Э; Мэтьюз, Э; Пайола, М; Солдаты, Ф; Картаччи, М; Кассенти, Ф; Холодильник, А; Джоппи, С; Мартуфи, Р; Масдеа, А; Митра, Г; Ненна, К; Ношезе, Р; Оставайся, М; Сеу, Р. (2018). «Радиолокационные доказательства наличия подледной жидкой воды на Марсе» . Наука . 361 (6401): 490–493. arXiv : 2004.04587 . Бибкод : 2018Sci...361..490O . дои : 10.1126/science.aar7268 . ПМИД 30045881 .
- ^ «Марсоход Spirit обнаруживает неожиданные доказательства влажного прошлого» (пресс-релиз). Лаборатория реактивного движения . 21 мая 2007 г. Архивировано из оригинала 24 мая 2007 г.
- ^ Рафф, Стивен В.; Фармер, Джек Д. (2016). «Отложения кремнезема на Марсе, черты которых напоминают биосигнатуры горячих источников в Эль-Татио в Чили» . Природные коммуникации . 7 : 13554. Бибкод : 2016NatCo...713554R . дои : 10.1038/ncomms13554 . hdl : 2286/RI44704 . ПМЦ 5473637 . ПМИД 27853166 .
- ^ Левей, Р.Дж. (2010). «Минерализованные железоокисляющие бактерии из гидротермальных источников: нацеливание на биосигнатуры на Марсе». Тезисы осеннего собрания АГУ . 12 : P12A–07. Бибкод : 2010АГУФМ.П12А..07Л .
- ^ Уолтер, MR; Де Марэ, Дэвид Дж. (1993). «Сохранение биологической информации в отложениях термальных источников: разработка стратегии поиска ископаемой жизни на Марсе». Икар . 101 (1): 129–43. Бибкод : 1993Icar..101..129W . дои : 10.1006/icar.1993.1011 . ПМИД 11536937 .
- ^ Аллен, Карлтон С.; Альберт, Фред Г.; Чафец, Генри С.; Комби, Джоан; Грэм, Кэтрин Р.; Кифт, Томас Л.; Киветт, Стивен Дж.; Маккей, Дэвид С.; и др. (2000). «Микроскопические физические биомаркеры в карбонатных горячих источниках: значение для поиска жизни на Марсе». Икар . 147 (1): 49–67. Бибкод : 2000Icar..147...49A . дои : 10.1006/icar.2000.6435 . ПМИД 11543582 .
- ^ Уэйд, Мэнсон Л.; Агрести, Дэвид Г.; Вдовяк, Томас Дж.; Армендарес, Лоуренс П.; Фармер, Джек Д. (1999). «Мессбауэровское исследование богатых железом земных гидротермальных жерловых систем: уроки для исследования Марса» . Журнал геофизических исследований . 104 (Е4): 8489–507. Бибкод : 1999JGR...104.8489W . дои : 10.1029/1998JE900049 . ПМИД 11542933 .
- ^ Агрести, генеральный директор; Вдовяк, Ти Джей; Уэйд, ML; Армендарес, LP; Фермер, доктор юридических наук (1995). «Мессбауэровское исследование месторождений железа в горячих источниках». Тезисы докладов конференции по лунным и планетным наукам . 26 : 7. Бибкод : 1995LPI....26....7A .
- ^ Агрести, генеральный директор; Вдовяк, Ти Джей; Уэйд, ML; Армендарес, LP (1997). «Мессбауэровская спектроскопия месторождений железа термальных источников как марсианских аналогов». Ранний Марс: геологическая и гидрологическая эволюция . 916 : 1. Бибкод : 1997LPICo.916....1A .
- ^ Перейти обратно: а б Персонал (9 мая 2017 г.). «Самые древние свидетельства жизни на суше обнаружены в австралийских скалах возрастом 3,48 миллиарда лет» . Физика.орг . Архивировано из оригинала 10 мая 2017 года . Проверено 13 мая 2017 г.
- ^ Перейти обратно: а б Джокич, Тара; Ван Кранендонк, Мартин Дж.; Кэмпбелл, Кэтлин А.; Уолтер, Малкольм Р.; Уорд, Колин Р. (9 мая 2017 г.). «Самые ранние признаки жизни на суше сохранились в отложениях горячих источников возрастом около 3,5 млрд лет» . Природные коммуникации . 8 : 15263. Бибкод : 2017NatCo...815263D . дои : 10.1038/ncomms15263 . ПМЦ 5436104 . ПМИД 28486437 .
- ^ Мама, MJ; Новак, Р.Э.; ДиСанти, Массачусетс; Бонев, Б.П. (2003). «Чувствительный поиск метана на Марсе». Бюллетень Американского астрономического общества . 35 : 937. Бибкод : 2003DPS....35.1418M .
- ^ Найе, Роберт (28 сентября 2004 г.). «Марсианский метан повышает шансы на жизнь» . Небо и телескоп . Проверено 20 декабря 2014 г.
- ^ Хэнд, Эрик (2018). «Марсианский метан повышается и падает в зависимости от сезона». Наука . 359 (6371): 16–17. Бибкод : 2018Sci...359...16H . дои : 10.1126/science.359.6371.16 . ПМИД 29301992 .
- ^ НАСА (7 июня 2018 г.). «На Марсе обнаружена древняя органика — видео (03:17)» . НАСА . Архивировано из оригинала 7 июня 2018 года . Проверено 7 июня 2018 г.
- ^ Воосен, Пол (2018). «Ровер НАСА Curiosity столкнулся с органической грязью на Марсе» . Наука . 260 (6393): 1054–55. Бибкод : 2018Sci...360.1054V . дои : 10.1126/science.360.6393.1054 . ПМИД 29880665 . S2CID 47015070 .
- ^ тен Кейт, Инге Лоес (8 июня 2018 г.). «Органические молекулы на Марсе». Наука . 360 (6393): 1068–1069. Бибкод : 2018Sci...360.1068T . дои : 10.1126/science.aat2662 . hdl : 1874/366378 . ПМИД 29880670 . S2CID 46952468 .
- ^ Вебстер, Кристофер Р.; и др. (8 июня 2018 г.). «Фоновые уровни метана в атмосфере Марса демонстрируют сильные сезонные колебания» . Наука . 360 (6393): 1093–1096. Бибкод : 2018Sci...360.1093W . дои : 10.1126/science.aaq0131 . ПМИД 29880682 .
- ^ Уолл, Майк (23 февраля 2018 г.). «Орбитальный аппарат, нюхающий метан, завершает «тормозное» погружение в атмосферу Марса» . Space.com . Архивировано из оригинала 12 июня 2018 года . Проверено 24 февраля 2018 г.
- ^ Сведхем, Хакан; Ваго, Хорхе Л.; Бруинсма, Шон; Мюллер-Водарг, Инго; и др. (2017). Орбитальный аппарат ExoMars Trace Gas Orbiter предоставляет данные об атмосфере во время аэроторможения на финальную орбиту . 49-е ежегодное собрание Отдела планетарных наук. 15–20 октября 2017 г. Прово, штат Юта. Бибкод : 2017ДПС....4941801С . 418.01.
- ^ Ваго, Хорхе Л.; Сведхем, Хокан; Зеленый, Лев; Этиопа, Джузеппе; Уилсон, Колин Ф.; Лопес-Морено, Хосе-Хуан; Беллуччи, Джанкарло; Патель, Маниш Р.; Нифс, Эдди (апрель 2019 г.). «По данным ранних наблюдений орбитального аппарата ExoMars Trace Gas Orbiter, метана на Марсе не обнаружено» (PDF) . Природа . 568 (7753): 517–520. Бибкод : 2019Natur.568..517K . дои : 10.1038/s41586-019-1096-4 . ISSN 1476-4687 . ПМИД 30971829 . S2CID 106411228 .
- ^ эс. «Первые результаты орбитального корабля ExoMars Trace Gas» . Европейское космическое агентство . Проверено 12 июня 2019 г.
- ^ Мама, Майкл; и др. (2010). «Астробиология Марса: метан и другие газы-кандидаты-биомаркеры, а также соответствующие междисциплинарные исследования на Земле и Марсе» (PDF) . Научная конференция по астробиологии 2010 . Система астрофизических данных . Гринбелт, Мэриленд: Центр космических полетов Годдарда . Проверено 24 июля 2010 г.
- ^ Озе, К.; Шарма, М. (2005). «Есть оливин, будет газ: серпентинизация и абиогенное производство метана на Марсе» . Геофиз. Рез. Летт . 32 (10): L10203. Бибкод : 2005GeoRL..3210203O . дои : 10.1029/2005GL022691 . S2CID 28981740 .
- ^ «Охота на молодые потоки лавы» . Письма о геофизических исследованиях . Красная Планета. 1 июня 2011 г. Архивировано из оригинала 4 октября 2013 г.
- ^ Перейти обратно: а б с д Озе, Кристофер; Джонс, Камилла; Голдсмит, Джонас И.; Розенбауэр, Роберт Дж. (7 июня 2012 г.). «Дифференциация биотического и абиотического происхождения метана на гидротермально активных поверхностях планет» . ПНАС . 109 (25): 9750–9754. Бибкод : 2012PNAS..109.9750O . дои : 10.1073/pnas.1205223109 . ПМЦ 3382529 . ПМИД 22679287 .
- ^ Перейти обратно: а б Персонал (25 июня 2012 г.). «Марсианская жизнь может оставить следы в воздухе Красной планеты: исследование» . Space.com . Архивировано из оригинала 30 июня 2012 года.
- ^ Краснопольский Владимир А.; Майяр, Жан Пьер; Оуэн, Тобиас К. (декабрь 2004 г.). «Обнаружение метана в марсианской атмосфере: свидетельства жизни?». Икар . 172 (2): 537–547. Бибкод : 2004Icar..172..537K . дои : 10.1016/j.icarus.2004.07.004 .
- ^ «Марсоход НАСА на Марсе обнаружил облако газа, намекающее на возможность существования жизни» . Нью-Йорк Таймс . 22 июня 2019 г.
- ^ Перейти обратно: а б «Земные организмы выживают в марсианских условиях низкого давления» . Университет Арканзаса . 2 июня 2015 года. Архивировано из оригинала 4 июня 2015 года . Проверено 4 июня 2015 г.
- ^ Штайгервальд, Билл (15 января 2009 г.). «Марсианский метан показывает, что Красная планета не мертвая планета» . Центр космических полетов имени Годдарда НАСА . НАСА. Архивировано из оригинала 16 января 2009 года.
Если микроскопическая марсианская жизнь производит метан, он, вероятно, находится далеко под поверхностью, где еще достаточно тепло для существования жидкой воды.
- ^ Крал, Т.А.; Гудхарт, Т.; Хоу, КЛ; Гэвин, П. (2009). «Могут ли метаногены расти в перхлоратной среде на Марсе?». 72-е ежегодное собрание Метеоритического общества . 72 : 5136. Бибкод : 2009M&PSA..72.5136K .
- ^ Хоу, КЛ; Гэвин, П.; Гудхарт, Т.; Крал, Т.А. (2009). «Производство метана метаногенами в средах с добавками перхлоратов». 40-я конференция по наукам о Луне и планетах . 40 : 1287. Бибкод : 2009LPI....40.1287H .
- ^ Левин, Гилберт В.; Страат, Патрисия Энн (2009). «Метан и жизнь на Марсе». В Гувере, Ричард Б.; Левин, Гилберт V; Розанов Алексей Юрьевич; Ретерфорд, Курт Д. (ред.). Приборы и методы для астробиологии и планетарных миссий XII . Том. 7441. стр. 12–27. Бибкод : 2009SPIE.7441E..0DL . дои : 10.1117/12.829183 . ISBN 978-0-8194-7731-6 . S2CID 73595154 .
- ^ Броги, Маттео; Снеллен, Игнас АГ; де Крок, Ремко Дж.; Альбрехт, Саймон; Биркби, Джейн; де Муай, Эрнест Дж.В. (28 июня 2012 г.). «Сигнатура орбитального движения с дневной стороны планеты τ Boötis b». Природа . 486 (7404): 502–504. arXiv : 1206.6109 . Бибкод : 2012Natur.486..502B . дои : 10.1038/nature11161 . ПМИД 22739313 . S2CID 4368217 .
- ^ Манн, Адам (27 июня 2012 г.). «Новый взгляд на экзопланеты поможет в поисках инопланетян» Wired . Архивировано из оригинала 29 августа 2012 года.
- ^ Штайгервальд, Билл (15 января 2009 г.). «Марсианский метан показывает, что Красная планета не мертвая планета» . Центр космических полетов имени Годдарда НАСА . НАСА. Архивировано из оригинала 17 января 2009 года.
- ^ Пеплоу, Марк (25 февраля 2005 г.). «Заявление о формальдегиде разжигает марсианские дебаты». Природа . дои : 10.1038/news050221-15 . S2CID 128986558 .
- ^ Хоган, Дженни (16 февраля 2005 г.). «Запах жизни на Красной планете» . Новый учёный . Архивировано из оригинала 22 апреля 2008 года.
- ^ Пеплоу, Марк (7 сентября 2005 г.). «Марсианский метановый зонд в беде». Природа . дои : 10.1038/news050905-10 .
- ^ «Заявление НАСА о ложном утверждении о наличии жизни на Марсе» . Новости НАСА . НАСА . 18 февраля 2005 г. Архивировано из оригинала 22 сентября 2008 г.
- ^ Перейти обратно: а б с Левин, Гилберт В. (2007). «Анализ доказательств жизни на Марсе». Электронейробиология . 15 (2): 39–47. arXiv : 0705.3176 . Бибкод : 2007arXiv0705.3176L .
- ^ Левин, Гилберт В. (10 октября 2019 г.). «Я убежден, что мы нашли доказательства жизни на Марсе в 1970-х годах» . Сеть блогов Scientific American . Проверено 14 января 2020 г.
- ^ Клотц, Ирен (12 апреля 2012 г.). «Марсианские роботы-викинги нашли жизнь » (пресс-релиз). Дискавери Коммуникейшнс , ООО. Архивировано из оригинала 26 января 2013 года.
- ^ Крокко, Марио; Контрерас, Северная Каролина (2008). Folia Neurobiológica Argentina Vol. XI, «Палиндром: сознательные живые существа как инструменты природы; природа как инструмент сознательных живых существ» . Analysis Editions, Буэнос-Айрес – Росарио – Баия-Бланка. п. 70. ИСБН 978-987-29362-0-4 .
- ^ Наварро-Гонсалес, Рафаэль; Варгас, Эдгар; де ла Роса, Хосе; Рага, Алехандро К.; Маккей, Кристофер П. (15 декабря 2010 г.). «Повторный анализ результатов «Викинга» предполагает наличие перхлората и органики в средних широтах Марса» . Журнал геофизических исследований: Планеты . 115 (Е12010): Е12010. Бибкод : 2010JGRE..11512010N . дои : 10.1029/2010JE003599 . Архивировано из оригинала 9 января 2011 года . Проверено 7 января 2011 г.
- ^ Наварро-Гонсалес, Рафаэль; Варгас, Эдгар; де ла Роса, Хосе; Рага, Алехандро К.; Маккей, Кристофер П. (2011). «Поправка к «Повторному анализу результатов Викинга предполагает наличие перхлората и органики в средних широтах Марса» » . Журнал геофизических исследований . 116 (Е8): E08011. Бибкод : 2011JGRE..116.8011N . дои : 10.1029/2011JE003854 .
- ^ Наварро-Гонсалес, Рафаэль; Варгас, Эдгар; де ла Роса, Хосе; Рага, Алехандро К.; Маккей, Кристофер П. (2010). «Повторный анализ результатов «Викинга» предполагает наличие перхлората и органики в средних широтах Марса». Журнал геофизических исследований . Том. 115. Бибкод : 2010JGRE..11512010N . дои : 10.1029/2010JE003599 .
- ^ Джонсон, Джон (6 августа 2008 г.). «Перхлорат обнаружен в марсианской почве» . Лос-Анджелес Таймс . Архивировано из оригинала 18 марта 2009 года.
- ^ Перейти обратно: а б «Марсианская жизнь или нет? Команда НАСА « Феникс » анализирует результаты» . Наука Дейли. 6 августа 2008 г. Архивировано из оригинала 5 марта 2016 г.
- ^ «Нашли ли марсоходы викингов строительные блоки жизни? Недостающая часть вдохновляет на новый взгляд на головоломку» . ScienceDaily . 5 сентября 2010 года. Архивировано из оригинала 8 сентября 2010 года . Проверено 23 сентября 2010 г.
- ^ Наварро-Гонсалес, Рафаэль; и др. (2011). «Комментарий к статье «Повторный анализ результатов «Викинга» предполагает наличие перхлората и органики в средних широтах Марса» . Журнал геофизических исследований . 116 (E12): E12001. Bibcode : 2011JGRE..11612001B . doi : 10.1029/2011JE003869 .
- ^ Левин, Гилберт В.; Страат, Патрисия Энн. МАРС: мертвый или живой? (PDF) . Съезд Марсинского общества. Архивировано (PDF) из оригинала 19 августа 2014 г.
- ^ Актуальный список марсианских метеоритов, заархивированный 24 июля 2018 года в Wayback Machine . Доктор Тони Ирвинг из Вашингтонского университета. Международная ассоциация коллекционеров метеоритов (IMCA Inc).
- ^ Перейти обратно: а б с д и Гибсон, Э.К. младший; Вестолл, Ф.; Маккей, Д.С.; Томас-Кепрта, К.; Вентворт, С.; Романек, CS (1999). «Доказательства древней марсианской жизни» (PDF) . Пятая Международная конференция по Марсу, 19–24 июля 1999 г., Пасадена, Калифорния, Научная конференция по Луне и планетам (Аннотация). НАСА. п. 6142. Бибкод : 1999ficm.conf.6142G . Архивировано (PDF) из оригинала 19 марта 2015 г.
- ^ Кренсон, Мэтт (6 августа 2006 г.). «Спустя 10 лет мало кто верит в жизнь на Марсе» . Space.com . Ассошиэйтед Пресс . Архивировано из оригинала 9 августа 2006 года.
- ^ Маккей, Дэвид С.; Гибсон, Эверетт К.; Томас-Кепрта, Кэти Л.; Вали, Ходжатолла; Романек, Кристофер С.; Клеметт, Саймон Дж.; Чиллер, Ксавье Д.Ф.; Мехлинг, Клод Р.; Заре, Ричард Н. (1996). «Поиски прошлой жизни на Марсе: возможная реликтовая биогенная активность марсианского метеорита ALH84001». Наука . 273 (5277): 924–30. Бибкод : 1996Sci...273..924M . дои : 10.1126/science.273.5277.924 . ПМИД 8688069 . S2CID 40690489 .
- ^ Баалке, Рон (1995). «Метеорит Нахла» . Лаборатория реактивного движения . НАСА. Архивировано из оригинала 14 сентября 2008 года . Проверено 17 августа 2008 г.
- ^ «Вращающееся изображение фрагмента метеорита Нахла» . Лондон: Музей естественной истории. 2008. Архивировано из оригинала 16 июля 2006 года.
- ^ Ринкон, Пол (8 февраля 2006 г.). «Космический рок возобновляет дискуссию о Марсе» . Новости Би-би-си . Архивировано из оригинала 22 февраля 2006 года.
- ^ Мейер, К. (2004). «Сборник марсианских метеоритов» (PDF) . НАСА. Архивировано (PDF) из оригинала 23 сентября 2008 г.
- ^ Уайтхаус, Дэвид (27 августа 1999 г.). «Жизнь на Марсе – новые претензии» . Новости Би-би-си . Архивировано из оригинала 2 мая 2008 года.
- ^ Сборник справок по научным исследованиям метеорита Нахла: «Ссылки на Нахлу» . Архивировано из оригинала 4 сентября 2008 года . Проверено 21 августа 2008 г.
- ^ «Шерготский метеорит» . Лаборатория реактивного движения, НАСА. Архивировано из оригинала 18 января 2011 года.
- ^ Перейти обратно: а б Вебстер, Гай (27 февраля 2014 г.). «Ученые НАСА нашли доказательства наличия воды в метеорите, возобновив дебаты о жизни на Марсе» . НАСА . Архивировано из оригинала 1 марта 2014 года.
- ^ Перейти обратно: а б Уайт, Лорен М.; Гибсон, Эверетт К.; Томнас-Кепрта, Кэти Л.; Клеметт, Саймон Дж.; Маккей, Дэвид (19 февраля 2014 г.). «Предполагаемые местные углеродсодержащие изменения в марсианском метеорите Ямато 000593» . Астробиология . 14 (2): 170–181. Бибкод : 2014AsBio..14..170W . дои : 10.1089/ast.2011.0733 . ПМЦ 3929347 . ПМИД 24552234 .
- ^ Перейти обратно: а б Гэннон, Меган (28 февраля 2014 г.). «Марсианский метеорит со странными «туннелями» и «сферами» возрождает дебаты о древней марсианской жизни» . Space.com . Архивировано из оригинала 1 марта 2014 года.
- ^ Зейлахер, Адольф. (2007). Трассовый анализ окаменелостей . Берлин: Шпрингер. ISBN 978-3-540-47226-1 . OCLC 191467085 .
- ^ Маклафлин, Н.; Штаудигель, Х.; Фурнес, Х.; Эйкманн, Б.; Иварссон, М. (2010). «Механизмы микротоннелирования в каменных субстратах: отличие эндолитных биосигнатур от абиотических микротоннелей» . Геобиология . 8 (4): 245–255. Бибкод : 2010Gbio....8..245M . дои : 10.1111/j.1472-4669.2010.00243.x . ISSN 1472-4669 . ПМИД 20491948 . S2CID 46368300 .
- ^ Натман, Аллен П.; Беннетт, Вики С.; Друг, Кларк Р.Л.; Ван Кранендонк, Мартин Дж.; Чивас, Аллан Р. (сентябрь 2016 г.). «Быстрое возникновение жизни, продемонстрированное открытием микробных структур возрастом 3700 миллионов лет» . Природа . 537 (7621): 535–538. Бибкод : 2016Natur.537..535N . дои : 10.1038/nature19355 . ISSN 1476-4687 . ПМИД 27580034 . S2CID 205250494 .
- ^ Омото, Хироши; Раннегар, Брюс; Камп, Ли Р.; Фогель, Мэрилин Л.; Камбер, Бальц; Анбар, Ариэль Д.; Кнаут, Пол Л.; Лоу, Дональд Р.; Самнер, Дон Ю.; Ватанабэ, Юмико (1 октября 2008 г.). «Биосигнатуры в древних камнях: краткое изложение обсуждений на полевом семинаре по биосигнатурам в древних камнях» . Астробиология . 8 (5): 883–907. Бибкод : 2008AsBio...8..883O . дои : 10.1089/ast.2008.0257 . ISSN 1531-1074 . ПМИД 19025466 .
- ^ Йенсен, Сорен (1 февраля 2003 г.). «Протерозойские и самые ранние кембрийские следы ископаемых: закономерности, проблемы и перспективы» . Интегративная и сравнительная биология . 43 (1): 219–228. дои : 10.1093/icb/43.1.219 . ISSN 1540-7063 . ПМИД 21680425 .
- ^ Альбани, Абдерразак Эль; Мангано, М. Габриэла; Буатойс, Луис А.; Бенгтсон, Стефан; Рибулло, Армель; Беккер, Андрей; Конхаузер, Курт; Лайонс, Тимоти; Роллион-Бард, Клэр; Банколе, Олабоде; Багекема, Стеллина Гвенаэль Лекеле (26 февраля 2019 г.). «Подвижность организмов в насыщенной кислородом мелководной морской среде 2,1 миллиарда лет назад» . Труды Национальной академии наук . 116 (9): 3431–3436. Бибкод : 2019PNAS..116.3431E . дои : 10.1073/pnas.1815721116 . ISSN 0027-8424 . ПМК 6397584 . ПМИД 30808737 .
- ^ Перейти обратно: а б Бокон, Андреа; Нето де Карвалью, Карлос; Барбьери, Роберто; Бернардини, Федерико; Кавалацци, Барбара; Челани, Антонио; Феллетти, Фабрицио; Ферретти, Анналиса; Шёнлауб, Ганс Петер; Тодаро, Антонио; Тунис, Клаудио (1 августа 2017 г.). «Организм-субстратные взаимодействия и астробиология: потенциал, модели и методы» . Обзоры наук о Земле . 171 : 141–180. Бибкод : 2017ESRv..171..141B . doi : 10.1016/j.earscirev.2017.05.009 . ISSN 0012-8252 .
- ^ Бокон, Андреа; Нето Де Карвальо, Карлос; Феллетти, Фабрицио; Кабелла, Роберто (2020). «Ихнофоссилии, трещины или кристаллы? Тест на биогенность палообразных структур с хребта Вера Рубин, Марс» . Геонауки . 10 (2): 39. Бибкод : 2020Geosc..10...39B . doi : 10.3390/geosciences10020039 . HDL : 2434/717600 .
- ^ Фиск, г-н; Попа, Р.; Мейсон, Оу; Сторри-Ломбарди, Мак; Виченци, член парламента (1 февраля 2006 г.). «Биовыветривание силикатов железа и магния на Земле (и Марсе?)» . Астробиология . 6 (1): 48–68. Бибкод : 2006AsBio...6...48F . дои : 10.1089/ast.2006.6.48 . ISSN 1531-1074 . ПМИД 16551226 .
- ^ Маккей, Д.С.; Гибсон, ЕК; Томас-Кепрта, КЛ; Вали, Х.; Романек, CS; Клеметт, С.Дж.; Чиллер, XDF; Мехлинг, ЧР; Заре, Р.Н. (16 августа 1996 г.). «Поиски прошлой жизни на Марсе: возможная реликтовая биогенная активность марсианского метеорита ALH84001» . Наука . 273 (5277): 924–930. Бибкод : 1996Sci...273..924M . дои : 10.1126/science.273.5277.924 . ISSN 0036-8075 . ПМИД 8688069 . S2CID 40690489 .
- ^ «Результаты НАСА позволяют предположить, что из ледяной шапки Марса вырываются самолеты» . Лаборатория реактивного движения . НАСА. 16 августа 2006 г. Архивировано из оригинала 10 октября 2009 г.
- ^ Киффер, Х.Х. (2000). «Ежегодные прерывистые плиты CO2, лед и струи на Марсе». Международная конференция по полярной науке и исследованию Марса (1057): 93. Бибкод : 2000mpse.conf...93K .
- ^ Портянкина Г.; Маркевич, WJ; Гарсиа-Комас, М.; Келлер, Хьюстон; Бибринг, Ж.-П.; Нойкум, Г. (2006). «Моделирование извержений гейзерного типа в загадочной области южной полярной шапки Марса». Четвертая международная конференция по полярной науке и исследованию Марса . 1323 : 8040. Бибкод : 2006LPICo1323.8040P .
- ^ Киффер, Хью Х.; Кристенсен, Филип Р.; Титус, Тимоти Н. (2006). «Струи CO2 образуются в результате сублимации под полупрозрачным плитовым льдом в сезонной южной полярной ледяной шапке Марса». Природа . 442 (7104): 793–6. Бибкод : 2006Natur.442..793K . дои : 10.1038/nature04945 . ПМИД 16915284 . S2CID 4418194 .
- ^ Перейти обратно: а б с Несс, Питер К.; Грег М. Орм (2002). «Модели паучьего ущелья и растительные особенности на Марсе - возможные геофизические и биогеофизические способы происхождения» (PDF) . Журнал Британского межпланетного общества (JBIS) . 55 : 85–108. Архивировано из оригинала (PDF) 20 февраля 2012 года . Проверено 3 сентября 2009 г.
- ^ Хорват, А.; Ганти, Т.; Гестези, А.; Берчи, С.; Сатмари, Э. (2001). «Вероятные свидетельства недавней биологической активности на Марсе: появление и рост темных пятен дюн в южной полярной области». 32-я ежегодная конференция по науке о Луне и планетах . 32 : 1543. Бибкод : 2001LPI....32.1543H .
- ^ Поч, Т.; Хорват, А.; Ганти, Т.; Берчи, С.; Сатемари, Э. (2004). «Возможна криптобиотическая кора на Марсе?». Материалы третьего европейского семинара по экзоастробиологии . 545 : 265–6. Бибкод : 2004ESASP.545..265P .
- ^ Ганти, Тибор; Хорват, Андраш; Берчи, Санисло; Гестези, Альберт; Сатмари, Эорс (2003). «Пятна темных дюн: возможные биомаркеры на Марсе?». Происхождение жизни и эволюция биосферы . 33 (4/5): 515–57. Бибкод : 2003ОЛЕВ...33..515Г . дои : 10.1023/А:1025705828948 . ПМИД 14604189 . S2CID 23727267 .
- ^ Хорват, А.; Ганти, Т.; Берчи, С.; Гестези, А.; Сатмари, Э. (2002). «Морфологический анализ пятен темных дюн на Марсе: новые аспекты биологической интерпретации». 33-я ежегодная конференция по науке о Луне и планетах . 33 : 1108. Бибкод : 2002LPI....33.1108H .
- ^ Андраш Сик, Акош Керестури. «Пятна темных дюн – может быть, оно живое?» . Монохром. Архивировано из оригинала 3 сентября 2009 года . Проверено 4 сентября 2009 г. (Аудиоинтервью, MP3 6 мин.)
- ^ Орм, Грег М.; Несс, Питер К. (9 июня 2003 г.). «Марсианские пауки» (PDF) . Марсбаги . 10 (23): 5–7. Архивировано из оригинала (PDF) 27 сентября 2007 г.
- ^ Манрубия, Южная Каролина; Арбалеты Прието, О.; Гонсалес Кесслер, К.; Фернандес Ремолар, Д.; Кордова-Мыловар, К.; Селсис, Ф.; Берчи, С.; Ганти, Т.; Хорват, А. (2004). Сравнительный анализ геологических особенностей и сезонных процессов в регионах «Город инков» и «Питьюса Патера» на Марсе . Том. 545. стр. 100-1. 77–80. Бибкод : 2004ESASP.545...77M . ISBN 978-92-9092-856-0 .
{{cite book}}
:|journal=
игнорируется ( помогите ) - ^ Лэндис, Джеффри; Олесон, Стивен; Макгуайр, Мелисса (2012). Исследование конструкции бункера для марсианского гейзера . 50-е совещание AIAA по аэрокосмическим наукам. Нэшвилл. дои : 10.2514/6.2012-631 . hdl : 2060/20120004036 . Архивировано из оригинала 3 июня 2016 года.
- ^ Комитет по астробиологической стратегии исследования Марса; Национальный исследовательский совет (2007). «Планетарная защита миссий на Марс» . Астробиологическая стратегия исследования Марса . Пресса национальных академий. стр. 95–98. ISBN 978-0-309-10851-5 .
- ^ Коуинг, Кейт (11 апреля 2013 г.). «Планетарная защита: работа в процессе» . Астробиология . Архивировано из оригинала 16 июня 2013 года . Проверено 2 июня 2013 г.
- ^ Дебус, А. (2005). «Оценка и оценка загрязнения Марса». Достижения в космических исследованиях . 35 (9): 1648–53. Бибкод : 2005AdSpR..35.1648D . дои : 10.1016/j.asr.2005.04.084 . ПМИД 16175730 .
- ^ Перейти обратно: а б Дартнелл, Льюис Р.; Хантер, Стефани Дж.; Ловелл, Кейт В.; Коутс, Эндрю Дж.; Уорд, Джон М. (2010). «Устойчивость к низкотемпературному ионизирующему излучению Deinococcus radiodurans и бактерий антарктической сухой долины». Астробиология . 10 (7): 717–32. Бибкод : 2010AsBio..10..717D . дои : 10.1089/ast.2009.0439 . ПМИД 20950171 .
- ^ де ла Вега, У. Погода; Реттберг, П.; Рейтц, Г. (2007). «Моделирование климатических условий на поверхности Марса и их влияние на Deinococcus radiodurans ». Достижения в космических исследованиях . 40 (11): 1672–7. Бибкод : 2007AdSpR..40.1672D . дои : 10.1016/j.asr.2007.05.022 .
- ^ Шуергер, Эндрю К.; Ульрих, Ричард; Берри, Бонни Дж.; Николсон., Уэйн Л. (февраль 2013 г.). «Рост Serratia liquefaciens при давлении 7 мбар, 0 ° C и в аноксической атмосфере, обогащенной CO2» . Астробиология . 13 (2): 115–131. Бибкод : 2013AsBio..13..115S . дои : 10.1089/ast.2011.0811 . ПМЦ 3582281 . ПМИД 23289858 .
- ^ Скоулз, Сара (24 июля 2020 г.). «Доктор из нацистской Германии и истоки охоты за жизнью на Марсе» . Нью-Йорк Таймс . ISSN 0362-4331 . Проверено 24 июля 2020 г.
- ^ де Вера, Жан-Пьер; Мёльманн, Дидрих; Бутина, Фредерика; Лорек, Андреас; Вернеке, Роланд; Отт, Зиглинде (2010). «Потенциал выживания и фотосинтетическая активность лишайников в условиях Марса: лабораторное исследование». Астробиология . 10 (2): 215–27. Бибкод : 2010AsBio..10..215D . дои : 10.1089/ast.2009.0362 . ПМИД 20402583 .
- ^ де Вера, Ж.-ПП; Шульце-Макух, Д.; Хан, А.; Лорек, А.; Конц, А.; Мёльманн, Д.; Спон, Т. (2012). «Адаптационный потенциал экстремофилов к условиям поверхности Марса и его значение для обитаемости Марса». Генеральная ассамблея ЕГУ 2012 . 14 : 2113. Бибкод : 2012EGUGA..14.2113D .
- ^ «Выжить в условиях Марса» . ДЛР. 26 апреля 2012 г. Архивировано из оригинала 13 ноября 2012 г.
- ^ де Вера, Жан-Пьер (2012). «Лишайники как выжившие в космосе и на Марсе». Грибная экология . 5 (4): 472–9. Бибкод : 2012FunE....5..472D . дои : 10.1016/j.funeco.2012.01.008 .
- ^ де ла Торре Ноэцель, Р.; Санчес Иниго, Ф.Дж.; Рэббоу, Э.; Хорнек, Г.; де Вера, JP; Санчо, LG (июнь 2007 г.). «Лишайники выживают в космосе: результаты эксперимента ЛИШАЙНИКИ 2005 года». Астробиология . 7 (3): 443–454. Бибкод : 2007AsBio...7..443S . дои : 10.1089/ast.2006.0046 . ПМИД 17630840 .
- ^ Санчес, Ф.Дж.; Матео-Марти, Э.; Раджио, Дж.; Мессен, Дж.; Мартинес-Фриас, Дж.; Санчо, LG; Отт, С.; де ла Торре, Р. (2012). «Сопротивление лишайника Circinaria gyrosa (номинальное обозначение Provis.) к моделируемым условиям Марса - модельный тест на выживаемость эукариотического экстремофила». Планетарная и космическая наука . 72 (1): 102–10. Бибкод : 2012P&SS...72..102S . дои : 10.1016/j.pss.2012.08.005 .
- ^ Файрен, Альберто Г.; Парро, Виктор; Шульце-Макух, Дирк; Уайт, Лайл (2018). «Является ли поиск марсианской жизни приоритетом марсианского сообщества?» . Астробиология . 18 (2): 101–107. Бибкод : 2018AsBio..18..101F . дои : 10.1089/ast.2017.1772 . ПМК 5820680 . ПМИД 29359967 .
- ^ Перейти обратно: а б с д и Рост бактерий и выживание в экстремальных химических и физических условиях Марса и ледяных миров. Шнегурт, Марк; Чен, Фэй; Кларк, Бентон; Уилкс, Джонатан; Заид, Хади; Джоад, Мэриленд; Махди, Аммар; Збиб, Хасан. 42-я Научная ассамблея КОСПАР. Состоялось 14–22 июля 2018 г., Пасадена, Калифорния, США, Abstract id. Ф3.1-14-18.
- ^ Богатая хлоратом почва может помочь нам найти жидкую воду на Марсе. Архивировано 9 января 2019 года в Wayback Machine Лизы Каспин-Пауэлл, журнал Astrobiology . 3 января 2019 г. Опубликовано Space.com .
- ^ Тонер, Джей Ди; Кэтлинг, округ Колумбия (2018). «Хлоратные рассолы на Марсе: последствия возникновения жидкой воды и расплывания». Письма о Земле и планетологии . 497 : 161–168. Бибкод : 2018E&PSL.497..161T . дои : 10.1016/j.epsl.2018.06.011 . S2CID 134197775 .
- ^ Ахенбах, Джоэл (21 февраля 2023 г.). «Странная ДНК, найденная в пустыне, дает уроки по поиску жизни на Марсе» . Вашингтон Пост . Проверено 21 февраля 2023 г.
- ^ Азуа-Бустос, Армандо; и др. (21 февраля 2023 г.). «Темный микробиом и чрезвычайно низкое содержание органических веществ в окаменелой дельте Атакамы раскрывают пределы обнаружения жизни на Марсе» . Природные коммуникации . 14 (808): 808. Бибкод : 2023NatCo..14..808A . дои : 10.1038/s41467-023-36172-1 . ПМЦ 9944251 . ПМИД 36810853 .
- ^ Роббинс, Стюарт (2008). « Марсианская программа «Путешествие по Галактике»: Марс ~ 1960–1974» . СДЖР Дизайн. Архивировано из оригинала 4 февраля 2014 года . Проверено 26 января 2014 г.
- ^ Михос, Крис (11 января 2006 г.). «Марс (1960–1974): Марс-1» . Кафедра астрономии Университета Кейс Вестерн Резерв . Архивировано из оригинала 13 октября 2013 года . Проверено 26 января 2014 г.
- ^ «Российский спускаемый аппарат «Марс-3» может быть найден российскими любителями» . Планетарное общество . Проверено 6 июня 2023 г.
- ^ Момсен, Билл (2006). «Маринер IV - Первый пролет Марса: немного личного опыта» . п. 1. Архивировано из оригинала 20 июня 2002 года . Проверено 11 февраля 2009 г.
- ^ Момсен, Билл (2006). «Маринер IV - Первый пролет Марса: немного личного опыта» . п. 2. Архивировано из оригинала 30 декабря 2008 года . Проверено 11 февраля 2009 г.
- ^ Стром, Р.Г.; Крофт, Стивен К.; Барлоу, Надин Г. (1992). Запись о марсианских кратерах . Издательство Университета Аризоны. Бибкод : 1992mars.book..383S . ISBN 978-0-8165-1257-7 . [ нужна страница ]
- ^ Реберн, П. (1998). «Раскрытие тайн Красной планеты Марс». Национальное географическое общество . [ нужна страница ]
- ^ Мур, П.; и др. (1990). Атлас Солнечной системы . Нью-Йорк: Издательство Митчелл Бизли. [ нужна страница ]
- ^ «Астробиология» . Биологический кабинет. 26 сентября 2006 г. Архивировано из оригинала 12 декабря 2010 г.
- ^ Пласко, Кевин В.; Гросс, Майкл (2011). Астробиология: Краткое введение . Джу Пресс. стр. 282–283. ISBN 978-1-4214-0194-2 . Архивировано из оригинала 20 сентября 2014 года.
- ^ Горовиц, Нью-Хэмпшир (1986). Утопия и Назад и поиски жизни в Солнечной системе. Нью-Йорк: WH Freeman and Company. ISBN 0-7167-1766-2
- ^ Стенджер, Ричард (7 ноября 2000 г.). «План возвращения образцов с Марса несет в себе микробный риск, предупреждает группа» . Си-Эн-Эн. Архивировано из оригинала 7 октября 2013 года.
- ^ Пласко, Кевин В.; Гросс, Майкл (2006). Астробиология: Краткое введение . Джу Пресс. п. 223 . ISBN 978-0-8018-8366-8 .
- ^ Перейти обратно: а б Пласко, Кевин В.; Гросс, Майкл (2011). Астробиология: Краткое введение (2-е изд.). Джу Пресс. стр. 285–286. ISBN 978-1-4214-0194-2 . Архивировано из оригинала 1 апреля 2017 года.
- ^ Перейти обратно: а б с Вебстер, Гай; Нил-Джонс, Нэнси; Браун, Дуэйн (16 декабря 2014 г.). «Ровер НАСА обнаружил на Марсе активную и древнюю органическую химию» . НАСА . Архивировано из оригинала 17 декабря 2014 года . Проверено 16 декабря 2014 г.
- ^ Перейти обратно: а б с Чанг, Кеннет (16 декабря 2014 г.). « Великий момент»: марсоход нашел подсказку о том, что на Марсе может быть жизнь . Нью-Йорк Таймс . Архивировано из оригинала 16 декабря 2014 года . Проверено 16 декабря 2014 г.
- ^ Кляйн, Гарольд П.; Горовиц, Норман Х.; Левин, Гилберт В.; Ояма, Вэнс И.; Ледерберг, Джошуа; Рич, Александр; Хаббард, Джерри С.; Хобби, Джордж Л.; Страат, Патрисия А. (1976). «Биологическое исследование викингов: предварительные результаты». Наука . 194 (4260): 99–105. Бибкод : 1976Наука...194...99К . дои : 10.1126/science.194.4260.99 . ПМИД 17793090 . S2CID 24957458 .
- ^ Перейти обратно: а б Бьянкарди, Джорджо; Миллер, Джозеф Д.; Страат, Патрисия Энн; Левин, Гилберт В. (2012). «Анализ сложности экспериментов по выпуску меченных викингов» . Международный журнал авиационных и космических наук . 13 (1): 14–26. Бибкод : 2012IJASS..13...14B . дои : 10.5139/IJASS.2012.13.1.14 .
- ^ «Жизнь на Марсе обнаружена миссией НАСА «Викинг»?» . 15 апреля 2012 г. Архивировано из оригинала 4 июля 2013 г.
- ^ Клотц, Ирен (12 апреля 2012 г.). «Марсианские роботы-викинги нашли жизнь » . ДискавериНьюс . Архивировано из оригинала 14 апреля 2012 года.
- ^ Биманн, Клаус (2007). «О способности газового хроматографа-масс-спектрометра «Викинг» обнаруживать органические вещества» . Труды Национальной академии наук Соединенных Штатов Америки . 104 (25): 10310–10313. Бибкод : 2007PNAS..10410310B . дои : 10.1073/pnas.0703732104 . ЧВК 1965509 . ПМИД 17548829 .
- ^ Вебстер, Гай; Гувер, Рэйчел; Марлер, Рут; Фриас, Габриэла (3 сентября 2010 г.). «Недостающая часть вдохновляет на новый взгляд на загадку Марса» . Лаборатория реактивного движения НАСА. Архивировано из оригинала 3 ноября 2010 года . Проверено 24 октября 2010 г.
- ^ Пласко, Кевин В.; Гросс, Майкл (2011). Астробиология: Краткое введение (2-е изд.). Джу Пресс. стр. 282–283. ISBN 978-1-4214-0194-2 . Архивировано из оригинала 20 сентября 2014 года.
- ^ Биманн, К.; Бада, Дж.Л. (2011). «Комментарий Рафаэля Наварро-Гонсалеса и др. к статье «Повторный анализ результатов «Викинга» предполагает наличие перхлората и органических веществ в средних широтах Марса» . Журнал геофизических исследований . 116 (Е12): Е12001. Бибкод : 2011JGRE..11612001B . дои : 10.1029/2011JE003869 .
- ^ Наварро-Гонсалес Р.; Маккей, CP (2011). «Ответ на комментарий Бимана и Бады: «Повторный анализ результатов «Викинга» предполагает наличие перхлората и органических веществ в средних широтах Марса » . Журнал геофизических исследований . 116 (Е12): Е12002. Бибкод : 2011JGRE..11612002N . дои : 10.1029/2011JE003880 .
- ^ «Объединяя жизненный потенциал» . Марс Дейли . Архивировано из оригинала 5 августа 2014 года . Проверено 10 марта 2007 г.
- ^ «Космический аппарат НАСА подтвердил наличие перхлората на Марсе» . НАСА . 5 августа 2008 г. Архивировано из оригинала 3 марта 2009 г.
- ^ Джонсон, Джон (6 августа 2008 г.). «Перхлорат обнаружен в марсианской почве» . Лос-Анджелес Таймс . Архивировано из оригинала 18 марта 2009 года.
- ^ Лакдавалла, Эмили (26 июня 2008 г.). « Обновление на 30-е сол Феникса : щелочная почва, не очень соленая, «ничего экстремального» в этом!» . Блог Планетарного общества . Планетарное общество . Архивировано из оригинала 30 июня 2008 года.
- ^ Кунавес, СП; и др. (2014). «Свидетельства наличия марсианского перхлората, хлората и нитрата в марсианском метеорите EETA79001: последствия для окислителей и органических веществ». Икар . 2014 (229): 206–213. Бибкод : 2014Icar..229..206K . дои : 10.1016/j.icarus.2013.11.012 .
- ^ Кунавес, СП; и др. (2014). «Идентификация исходных солей перхлората на месте посадки на Марс в Фениксе и последствия». Икар . 232 : 226–231. Бибкод : 2014Icar..232..226K . дои : 10.1016/j.icarus.2014.01.016 .
- ^ «Запуск марсианской научной лаборатории» . 26 ноября 2011 г. Архивировано из оригинала 4 июля 2012 г.
- ^ «НАСА запускает на Марс суперразмерный вездеход: «Вперед, вперед!» " . Нью-Йорк Таймс . Ассошиэйтед Пресс . 26 ноября 2011 г.
- ^ Геологическая служба США (16 мая 2012 г.). «Три новых имени одобрены для использования на Марсе» . Геологическая служба США . Архивировано из оригинала 28 июля 2012 года . Проверено 3 мая 2019 г.
- ^ Сотрудники НАСА (27 марта 2012 г.). « Гора Шарп» на Марсе по сравнению с тремя большими горами на Земле . НАСА . Архивировано из оригинала 31 марта 2012 года.
- ^ Эгл, округ Колумбия (28 марта 2012 г.). « Гора Шарп» на Марсе связывает прошлое и будущее геологии» . НАСА . Архивировано из оригинала 31 марта 2012 года.
- ^ Персонал (29 марта 2012 г.). «Новый марсоход НАСА исследует возвышающуюся гору Шарп » . Space.com . Архивировано из оригинала 30 марта 2012 года.
- ^ Вебстер, Гай; Браун, Дуэйн (22 июля 2011 г.). «Следующий марсоход НАСА приземлится в кратере Гейла» . Лаборатория реактивного движения НАСА . Архивировано из оригинала 26 июля 2011 года.
- ^ Чоу, Деннис (22 июля 2011 г.). «Следующий марсоход НАСА приземлится в огромном кратере Гейла» . Space.com . Архивировано из оригинала 23 июля 2011 года.
- ^ Амос, Джонатан (22 июля 2011 г.). «Марсоход нацелен на глубокий кратер» . Новости Би-би-си . Архивировано из оригинала 22 июля 2011 года.
- ^ «Летучие вещества, выделяющиеся при нагревании порошка марсианской породы «Камберленд» | Изображение Марса» . mars.nasa.gov . Архивировано из оригинала 24 февраля 2017 года . Проверено 23 февраля 2017 г.
- ^ Коуинг, Кейт (21 декабря 2012 г.). «Группа научных определений для марсохода 2020 года» . НАСА . Наука Ссылка. Архивировано из оригинала 3 февраля 2013 года.
- ^ «ЭкзоМарс: ЕКА и Роскосмос готовятся к миссии на Марс» . Европейское космическое агентство (ЕКА) . 14 марта 2013 г. Архивировано из оригинала 16 марта 2013 г.
- ^ Фауст, Джефф (3 мая 2022 г.). «Официальный представитель ExoMars говорит, что запуск маловероятен раньше 2028 года» . Космические новости . Проверено 5 мая 2022 г.
- ^ Соображения планирования, связанные с органическим загрязнением марсианских образцов и последствиями для марсохода Mars 2020. По данным Комиссии по органическому загрязнению 2014 года. НАСА. 24 сентября 2014 г.
- ^ Моисей, Роберт В.; Бушнелл, Деннис М. (апрель 2016 г.). «Пограничное использование ресурсов на месте для обеспечения устойчивого присутствия человека на Марсе» (PDF) . НАСА . Архивировано (PDF) из оригинала 2 мая 2017 г. Проверено 3 октября 2017 г.
- ^ «Устав о слушаниях Комитета по науке Палаты представителей: Лунная наука и ресурсы: варианты будущего» . spaceref.com . Апрель 2004 г. Архивировано из оригинала 3 июля 2012 г. Проверено 12 июня 2015 г.
- ^ «Космическая гонка возобновилась? Россия стреляет на Луну и Марс» . Новости АВС . 2 сентября 2007 г. Архивировано из оригинала 22 сентября 2017 г. Проверено 2 сентября 2007 г.
Внешние ссылки
[ редактировать ]- Ученый говорит, что жизнь на Марсе возможна уже сегодня
- Древнее соленое море на Марсе признано самым важным научным достижением 2004 года – Journal Science
- Найденный на Земле марсианский метеор свидетельствует о том, что на Марсе когда-то существовала микробная жизнь
- Журнал Scientific American (выпуск за ноябрь 2005 г.) Жизнь пришла из другого мира?
- Аудиоинтервью о «Пятнах темных дюн»