Углеродные нанотрубки


Part of a series of articles on |
Nanomaterials |
---|
![]() |
Carbon nanotubes |
Fullerenes |
Other nanoparticles |
Nanostructured materials |
Углеродная нанотрубка ( УНТ ) представляет собой трубку из углерода диаметром в нанометровом диапазоне ( наноразмер ). Они являются одной из аллотропов углерода . Выделяют два широких класса углеродных нанотрубок:
- Одностенные углеродные нанотрубки ( ОУНТ ) имеют диаметр около 0,5–2,0 нанометров , что примерно в 100 000 раз меньше ширины человеческого волоса. Их можно идеализировать как вырезы из двумерного листа графена , свернутого в полый цилиндр. [1]
- Многостенные углеродные нанотрубки ( МУНТ ) состоят из вложенных друг в друга одностенных углеродных нанотрубок. [1] во вложенной конструкции «труба в трубке». [2] Углеродные нанотрубки с двойными и тройными стенками представляют собой особый случай МУНТ.
Углеродные нанотрубки могут проявлять замечательные свойства, такие как исключительная прочность на разрыв. [3] и теплопроводность [4] [5] [6] из-за их наноструктуры и прочности связей между атомами углерода. Некоторые структуры SWCNT обладают высокой электропроводностью. [7] [8] в то время как другие являются полупроводниками . [9] [10] Кроме того, углеродные нанотрубки можно химически модифицировать. [11] Ожидается, что эти свойства будут ценными во многих областях технологий, таких как электроника , оптика , композитные материалы (заменяющие или дополняющие углеродные волокна ), нанотехнологии (включая наномедицину). [12]) и другие применения материаловедения .
The predicted properties for SWCNTs were tantalising, but a path to synthesising them was lacking until 1993, when Iijima and Ichihashi at NEC, and Bethune and others at IBM independently discovered that co-vaporising carbon and transition metals such as iron and cobalt could specifically catalyse SWCNT formation.[13][14] These discoveries triggered research that succeeded in greatly increasing the efficiency of the catalytic production technique,[15] and led to an explosion of work to characterise and find applications for SWCNTs.
Structure of SWCNTs
[edit]Basic details
[edit]

The structure of an ideal (infinitely long) single-walled carbon nanotube is that of a regular hexagonal lattice drawn on an infinite cylindrical surface, whose vertices are the positions of the carbon atoms. Since the length of the carbon-carbon bonds is fairly fixed, there are constraints on the diameter of the cylinder and the arrangement of the atoms on it.[16]
In the study of nanotubes, one defines a zigzag path on a graphene-like lattice as a path that turns 60 degrees, alternating left and right, after stepping through each bond. It is also conventional to define an armchair path as one that makes two left turns of 60 degrees followed by two right turns every four steps. On some carbon nanotubes, there is a closed zigzag path that goes around the tube. One says that the tube is of the zigzag type or configuration, or simply is a zigzag nanotube. If the tube is instead encircled by a closed armchair path, it is said to be of the armchair type, or an armchair nanotube. An infinite nanotube that is of one type consists entirely of closed paths of that type, connected to each other.
The zigzag and armchair configurations are not the only structures that a single-walled nanotube can have. To describe the structure of a general infinitely long tube, one should imagine it being sliced open by a cut parallel to its axis, that goes through some atom A, and then unrolled flat on the plane, so that its atoms and bonds coincide with those of an imaginary graphene sheet—more precisely, with an infinitely long strip of that sheet. The two halves of the atom A will end up on opposite edges of the strip, over two atoms A1 and A2 of the graphene. The line from A1 to A2 will correspond to the circumference of the cylinder that went through the atom A, and will be perpendicular to the edges of the strip. In the graphene lattice, the atoms can be split into two classes, depending on the directions of their three bonds. Half the atoms have their three bonds directed the same way, and half have their three bonds rotated 180 degrees relative to the first half. The atoms A1 and A2, which correspond to the same atom A on the cylinder, must be in the same class. It follows that the circumference of the tube and the angle of the strip are not arbitrary, because they are constrained to the lengths and directions of the lines that connect pairs of graphene atoms in the same class.
Let u and v be two linearly independent vectors that connect the graphene atom A1 to two of its nearest atoms with the same bond directions. That is, if one numbers consecutive carbons around a graphene cell with C1 to C6, then u can be the vector from C1 to C3, and v be the vector from C1 to C5. Then, for any other atom A2 with same class as A1, the vector from A1 to A2 can be written as a linear combination n u + m v, where n and m are integers. And, conversely, each pair of integers (n,m) defines a possible position for A2.[16] Given n and m, one can reverse this theoretical operation by drawing the vector w on the graphene lattice, cutting a strip of the latter along lines perpendicular to w through its endpoints A1 and A2, and rolling the strip into a cylinder so as to bring those two points together. If this construction is applied to a pair (k,0), the result is a zigzag nanotube, with closed zigzag paths of 2k atoms. If it is applied to a pair (k,k), one obtains an armchair tube, with closed armchair paths of 4k atoms.
Types
[edit]The structure of the nanotube is not changed if the strip is rotated by 60 degrees clockwise around A1 before applying the hypothetical reconstruction above. Such a rotation changes the corresponding pair (n,m) to the pair (−2m,n+m). It follows that many possible positions of A2 relative to A1 — that is, many pairs (n,m) — correspond to the same arrangement of atoms on the nanotube. That is the case, for example, of the six pairs (1,2), (−2,3), (−3,1), (−1,−2), (2,−3), and (3,−1). In particular, the pairs (k,0) and (0,k) describe the same nanotube geometry. These redundancies can be avoided by considering only pairs (n,m) such that n > 0 and m ≥ 0; that is, where the direction of the vector w lies between those of u (inclusive) and v (exclusive). It can be verified that every nanotube has exactly one pair (n,m) that satisfies those conditions, which is called the tube's type. Conversely, for every type there is a hypothetical nanotube. In fact, two nanotubes have the same type if and only if one can be conceptually rotated and translated so as to match the other exactly. Instead of the type (n,m), the structure of a carbon nanotube can be specified by giving the length of the vector w (that is, the circumference of the nanotube), and the angle α between the directions of u and w, may range from 0 (inclusive) to 60 degrees clockwise (exclusive). If the diagram is drawn with u horizontal, the latter is the tilt of the strip away from the vertical.
Chirality and mirror symmetry
[edit]A nanotube is chiral if it has type (n,m), with m > 0 and m ≠ n; then its enantiomer (mirror image) has type (m,n), which is different from (n,m). This operation corresponds to mirroring the unrolled strip about the line L through A1 that makes an angle of 30 degrees clockwise from the direction of the u vector (that is, with the direction of the vector u+v). The only types of nanotubes that are achiral are the (k,0) "zigzag" tubes and the (k,k) "armchair" tubes. If two enantiomers are to be considered the same structure, then one may consider only types (n,m) with 0 ≤ m ≤ n and n > 0. Then the angle α between u and w, which may range from 0 to 30 degrees (inclusive both), is called the "chiral angle" of the nanotube.
Circumference and diameter
[edit]From n and m one can also compute the circumference c, which is the length of the vector w, which turns out to be:
in picometres. The diameter of the tube is then , that is
also in picometres. (These formulas are only approximate, especially for small n and m where the bonds are strained; and they do not take into account the thickness of the wall.)
The tilt angle α between u and w and the circumference c are related to the type indices n and m by:
where arg(x,y) is the clockwise angle between the X-axis and the vector (x,y); a function that is available in many programming languages as atan2
(y,x). Conversely, given c and α, one can get the type (n,m) by the formulas:
which must evaluate to integers.
Physical limits
[edit]Narrowest examples
[edit]If n and m are too small, the structure described by the pair (n,m) will describe a molecule that cannot be reasonably called a "tube", and may not even be stable. For example, the structure theoretically described by the pair (1,0) (the limiting "zigzag" type) would be just a chain of carbons. That is a real molecule, the carbyne; which has some characteristics of nanotubes (such as orbital hybridization, high tensile strength, etc.) — but has no hollow space, and may not be obtainable as a condensed phase. The pair (2,0) would theoretically yield a chain of fused 4-cycles; and (1,1), the limiting "armchair" structure, would yield a chain of bi-connected 4-rings. These structures may not be realizable.
The thinnest carbon nanotube proper is the armchair structure with type (2,2), which has a diameter of 0.3 nm. This nanotube was grown inside a multi-walled carbon nanotube. Assigning of the carbon nanotube type was done by a combination of high-resolution transmission electron microscopy (HRTEM), Raman spectroscopy, and density functional theory (DFT) calculations.[17]
The thinnest freestanding single-walled carbon nanotube is about 0.43 nm in diameter.[18] Researchers suggested that it can be either (5,1) or (4,2) SWCNT, but the exact type of the carbon nanotube remains questionable.[19] (3,3), (4,3), and (5,1) carbon nanotubes (all about 0.4 nm in diameter) were unambiguously identified using aberration-corrected high-resolution transmission electron microscopy inside double-walled CNTs.[20]
Length
[edit]The observation of the longest carbon nanotubes grown so far, around 0.5 metre (550 mm) long, was reported in 2013.[21] These nanotubes were grown on silicon substrates using an improved chemical vapor deposition (CVD) method and represent electrically uniform arrays of single-walled carbon nanotubes.[22]
The shortest carbon nanotube can be considered to be the organic compound cycloparaphenylene, which was synthesized in 2008 by Ramesh Jasti.[23] Other small molecule carbon nanotubes have been synthesized since.[24]
Density
[edit]The highest density of CNTs was achieved in 2013, grown on a conductive titanium-coated copper surface that was coated with co-catalysts cobalt and molybdenum at lower than typical temperatures of 450 °C. The tubes averaged a height of 380 nm and a mass density of 1.6 g cm−3. The material showed ohmic conductivity (lowest resistance ~22 kΩ).[25][26]
Variants
[edit]There is no consensus on some terms describing carbon nanotubes in the scientific literature: both "-wall" and "-walled" are being used in combination with "single", "double", "triple", or "multi", and the letter C is often omitted in the abbreviation, for example, multi-walled carbon nanotube (MWNT). The International Standards Organization uses single-wall or multi-wall in its documents.
Multi-walled
[edit]
Multi-walled nanotubes (MWNTs) consist of multiple rolled layers (concentric tubes) of graphene. There are two models that can be used to describe the structures of multi-walled nanotubes. In the Russian Doll model, sheets of graphite are arranged in concentric cylinders, e.g., a (0,8) single-walled nanotube (SWNT) within a larger (0,17) single-walled nanotube. In the Parchment model, a single sheet of graphite is rolled in around itself, resembling a scroll of parchment or a rolled newspaper. The interlayer distance in multi-walled nanotubes is close to the distance between graphene layers in graphite, approximately 3.4 Å. The Russian Doll structure is observed more commonly. Its individual shells can be described as SWNTs, which can be metallic or semiconducting. Because of statistical probability and restrictions on the relative diameters of the individual tubes, one of the shells, and thus the whole MWNT, is usually a zero-gap metal.[27]
Double-walled carbon nanotubes (DWNTs) form a special class of nanotubes because their morphology and properties are similar to those of SWNTs but they are more resistant to attacks by chemicals.[28] This is especially important when it is necessary to graft chemical functions to the surface of the nanotubes (functionalization) to add properties to the CNT. Covalent functionalization of SWNTs will break some C=C double bonds, leaving "holes" in the structure on the nanotube and thus modifying both its mechanical and electrical properties. In the case of DWNTs, only the outer wall is modified. DWNT synthesis on the gram-scale by the CCVD technique was first proposed in 2003[29] from the selective reduction of oxide solutions in methane and hydrogen.
The telescopic motion ability of inner shells[30] and their unique mechanical properties[31] will permit the use of multi-walled nanotubes as the main movable arms in upcoming nanomechanical devices.[speculation?] The retraction force that occurs to telescopic motion is caused by the Lennard-Jones interaction between shells, and its value is about 1.5 nN.[32]
Junctions and crosslinking
[edit]
Junctions between two or more nanotubes have been widely discussed theoretically.[33][34] Such junctions are quite frequently observed in samples prepared by arc discharge as well as by chemical vapor deposition. The electronic properties of such junctions were first considered theoretically by Lambin et al.,[35] who pointed out that a connection between a metallic tube and a semiconducting one would represent a nanoscale heterojunction. Such a junction could therefore form a component of a nanotube-based electronic circuit. The adjacent image shows a junction between two multiwalled nanotubes.
Junctions between nanotubes and graphene have been considered theoretically[36] and studied experimentally.[37] Nanotube-graphene junctions form the basis of pillared graphene, in which parallel graphene sheets are separated by short nanotubes.[38] Pillared graphene represents a class of three-dimensional carbon nanotube architectures.
Recently, several studies have highlighted the prospect of using carbon nanotubes as building blocks to fabricate three-dimensional macroscopic (>100 nm in all three dimensions) all-carbon devices. Lalwani et al. have reported a novel radical-initiated thermal crosslinking method to fabricate macroscopic, free-standing, porous, all-carbon scaffolds using single- and multi-walled carbon nanotubes as building blocks.[39] These scaffolds possess macro-, micro-, and nano-structured pores, and the porosity can be tailored for specific applications. These 3D all-carbon scaffolds/architectures may be used for the fabrication of the next generation of energy storage, supercapacitors, field emission transistors, high-performance catalysis, photovoltaics, and biomedical devices, implants, and sensors.[40][41]
Other morphologies
[edit]Carbon nanobuds are a newly created material combining two previously discovered allotropes of carbon: carbon nanotubes and fullerenes. In this new material, fullerene-like "buds" are covalently bonded to the outer sidewalls of the underlying carbon nanotube. This hybrid material has useful properties of both fullerenes and carbon nanotubes. In particular, they have been found to be exceptionally good field emitters.[42] In composite materials, the attached fullerene molecules may function as molecular anchors preventing slipping of the nanotubes, thus improving the composite's mechanical properties.
A carbon peapod[43][44] is a novel hybrid carbon material which traps fullerene inside a carbon nanotube. It can possess interesting magnetic properties with heating and irradiation. It can also be applied as an oscillator during theoretical investigations and predictions.[45][46]
In theory, a nanotorus is a carbon nanotube bent into a torus (doughnut shape). Nanotori are predicted to have many unique properties, such as magnetic moments 1000 times larger than that previously expected for certain specific radii.[47] Properties such as magnetic moment, thermal stability, etc. vary widely depending on the radius of the torus and the radius of the tube.[47][48]
Graphenated carbon nanotubes are a relatively new hybrid that combines graphitic foliates grown along the sidewalls of multiwalled or bamboo-style CNTs. The foliate density can vary as a function of deposition conditions (e.g., temperature and time) with their structure ranging from a few layers of graphene (< 10) to thicker, more graphite-like.[49] The fundamental advantage of an integrated graphene-CNT structure is the high surface area three-dimensional framework of the CNTs coupled with the high edge density of graphene. Depositing a high density of graphene foliates along the length of aligned CNTs can significantly increase the total charge capacity per unit of nominal area as compared to other carbon nanostructures.[50]
Cup-stacked carbon nanotubes (CSCNTs) differ from other quasi-1D carbon structures, which normally behave as quasi-metallic conductors of electrons. CSCNTs exhibit semiconducting behavior because of the stacking microstructure of graphene layers.[51]
Properties
[edit]Many properties of single-walled carbon nanotubes depend significantly on the (n,m) type, and this dependence is non-monotonic (see Kataura plot). In particular, the band gap can vary from zero to about 2 eV and the electrical conductivity can show metallic or semiconducting behavior.
Mechanical
[edit]Carbon nanotubes are the strongest and stiffest materials yet discovered in terms of tensile strength and elastic modulus. This strength results from the covalent sp2 bonds formed between the individual carbon atoms. In 2000, a multiwalled carbon nanotube was tested to have a tensile strength of 63 GPa (9,100,000 psi).[3] (For illustration, this translates into the ability to endure tension of a weight equivalent to 6,422 kilograms-force (62,980 N; 14,160 lbf) on a cable with cross-section of 1 mm2 (0.0016 sq in)). Further studies, such as one conducted in 2008, revealed that individual CNT shells have strengths of up to ≈100 GPa (15,000,000 psi), which is in agreement with quantum/atomistic models.[52] Because carbon nanotubes have a low density for a solid of 1.3 to 1.4 g/cm3,[53] its specific strength of up to 48,000 kN·m/kg is the best of known materials, compared to high-carbon steel's 154 kN·m/kg.
Although the strength of individual CNT shells is extremely high, weak shear interactions between adjacent shells and tubes lead to significant reduction in the effective strength of multiwalled carbon nanotubes and carbon nanotube bundles down to only a few GPa.[54] This limitation has been recently addressed by applying high-energy electron irradiation, which crosslinks inner shells and tubes, and effectively increases the strength of these materials to ≈60 GPa for multiwalled carbon nanotubes[52] and ≈17 GPa for double-walled carbon nanotube bundles.[54] CNTs are not nearly as strong under compression. Because of their hollow structure and high aspect ratio, they tend to undergo buckling when placed under compressive, torsional, or bending stress.[55]
On the other hand, there was evidence that in the radial direction they are rather soft. The first transmission electron microscope observation of radial elasticity suggested that even van der Waals forces can deform two adjacent nanotubes. Later, nanoindentations with an atomic force microscope were performed by several groups to quantitatively measure the radial elasticity of multiwalled carbon nanotubes and tapping/contact mode atomic force microscopy was also performed on single-walled carbon nanotubes. Young's modulus of on the order of several GPa showed that CNTs are in fact very soft in the radial direction.[citation needed]
It was reported in 2020, that CNT-filled polymer nanocomposites with 4 wt% and 6 wt% loadings are the most optimal concentrations, as they provide a good balance between mechanical properties and resilience of mechanical properties against UV exposure for the offshore umbilical sheathing layer.[56]
Electrical
[edit]
Unlike graphene, which is a two-dimensional semimetal, carbon nanotubes are either metallic or semiconducting along the tubular axis. For a given (n,m) nanotube, if n = m, the nanotube is metallic; if n − m is a multiple of 3 and n ≠ m, then the nanotube is quasi-metallic with a very small band gap, otherwise the nanotube is a moderate semiconductor.[57]Thus, all armchair (n = m) nanotubes are metallic, and nanotubes (6,4), (9,1), etc. are semiconducting.[58]Carbon nanotubes are not semimetallic because the degenerate point (the point where the π [bonding] band meets the π* [anti-bonding] band, at which the energy goes to zero) is slightly shifted away from the K point in the Brillouin zone because of the curvature of the tube surface, causing hybridization between the σ* and π* anti-bonding bands, modifying the band dispersion.
The rule regarding metallic versus semiconductor behavior has exceptions because curvature effects in small-diameter tubes can strongly influence electrical properties. Thus, a (5,0) SWCNT that should be semiconducting in fact is metallic according to the calculations. Likewise, zigzag and chiral SWCNTs with small diameters that should be metallic have a finite gap (armchair nanotubes remain metallic).[58] In theory, metallic nanotubes can carry an electric current density of 4 × 109 A/cm2, which is more than 1,000 times greater than those of metals such as copper,[59] where for copper interconnects, current densities are limited by electromigration. Carbon nanotubes are thus being explored as interconnects and conductivity-enhancing components in composite materials, and many groups are attempting to commercialize highly conducting electrical wire assembled from individual carbon nanotubes. There are significant challenges to be overcome however, such as undesired current saturation under voltage,[60] and the much more resistive nanotube-to-nanotube junctions and impurities, all of which lower the electrical conductivity of the macroscopic nanotube wires by orders of magnitude, as compared to the conductivity of the individual nanotubes.
Because of its nanoscale cross-section, electrons propagate only along the tube's axis. As a result, carbon nanotubes are frequently referred to as one-dimensional conductors. The maximum electrical conductance of a single-walled carbon nanotube is 2G0, where G0 = 2e2/h is the conductance of a single ballistic quantum channel.[61]
Because of the role of the π-electron system in determining the electronic properties of graphene, doping in carbon nanotubes differs from that of bulk crystalline semiconductors from the same group of the periodic table (e.g., silicon). Graphitic substitution of carbon atoms in the nanotube wall by boron or nitrogen dopants leads to p-type and n-type behavior, respectively, as would be expected in silicon. However, some non-substitutional (intercalated or adsorbed) dopants introduced into a carbon nanotube, such as alkali metals and electron-rich metallocenes, result in n-type conduction because they donate electrons to the π-electron system of the nanotube. By contrast, π-electron acceptors such as FeCl3 or electron-deficient metallocenes function as p-type dopants because they draw π-electrons away from the top of the valence band.
Intrinsic superconductivity has been reported,[62][63][64] although other experiments found no evidence of this, leaving the claim a subject of debate.[65]
In 2021, Michael Strano, the Carbon P. Dubbs Professor of Chemical Engineering at MIT, published department findings on the use of carbon nanotubes to create an electric current.[66] By immersing the structures in an organic solvent, the liquid drew electrons out of the carbon particles. Strano was quoted as saying, "This allows you to do electrochemistry, but with no wires," and represents a significant breakthrough in the technology.[67] Future applications include powering micro- or nanoscale robots, as well as driving alcohol oxidation reactions, which are important in the chemicals industry.[67]
Crystallographic defects also affect the tube's electrical properties. A common result is lowered conductivity through the defective region of the tube. A defect in metallic armchair-type tubes (which can conduct electricity) can cause the surrounding region to become semiconducting, and single monatomic vacancies induce magnetic properties.[68]
Optical
[edit]Carbon nanotubes have useful absorption, photoluminescence (fluorescence), and Raman spectroscopy properties. Spectroscopic methods offer the possibility of quick and non-destructive characterization of relatively large amounts of carbon nanotubes. There is a strong demand for such characterization from the industrial point of view: numerous parameters of nanotube synthesis can be changed, intentionally or unintentionally, to alter the nanotube quality, such as the non-tubular carbon content, structure (chirality) of the produced nanotubes, and structural defects. These features then determine nearly all other significant optical, mechanical, and electrical properties.
Carbon nanotube optical properties have been explored for use in applications such as for light-emitting diodes (LEDs)[69][70] and photo-detectors[71] based on a single nanotube have been produced in the lab. Their unique feature is not the efficiency, which is yet relatively low, but the narrow selectivity in the wavelength of emission and detection of light and the possibility of its fine-tuning through the nanotube structure. In addition, bolometer[72] and optoelectronic memory[73] devices have been realised on ensembles of single-walled carbon nanotubes. Nanotube fluorescence has been investigated for the purposes of imaging and sensing in biomedical applications.[74][75][76]
Thermal
[edit]All nanotubes are expected to be very good thermal conductors along the tube, exhibiting a property known as "ballistic conduction", but good insulators lateral to the tube axis. Measurements show that an individual SWNT has a room-temperature thermal conductivity along its axis of about 3500 W·m−1·K−1;[77] compare this to copper, a metal well known for its good thermal conductivity, which transmits 385 W·m−1·K−1. An individual SWNT has a room-temperature thermal conductivity lateral to its axis (in the radial direction) of about 1.52 W·m−1·K−1,[78] which is about as thermally conductive as soil. Macroscopic assemblies of nanotubes such as films or fibres have reached up to 1500 W·m−1·K−1 so far.[79] Networks composed of nanotubes demonstrate different values of thermal conductivity, from the level of thermal insulation with the thermal conductivity of 0.1 W·m−1·K−1 to such high values.[80] That is dependent on the amount of contribution to the thermal resistance of the system caused by the presence of impurities, misalignments and other factors. The temperature stability of carbon nanotubes is estimated to be up to 2800 °C in vacuum and about 750 °C in air.[81]
Crystallographic defects strongly affect the tube's thermal properties. Such defects lead to phonon scattering, which in turn increases the relaxation rate of the phonons. This reduces the mean free path and reduces the thermal conductivity of nanotube structures. Phonon transport simulations indicate that substitutional defects such as nitrogen or boron will primarily lead to the scattering of high-frequency optical phonons. However, larger-scale defects such as Stone–Wales defects cause phonon scattering over a wide range of frequencies, leading to a greater reduction in thermal conductivity.[82]
Synthesis
[edit]Techniques have been developed to produce nanotubes in sizeable quantities, including arc discharge, laser ablation, chemical vapor deposition (CVD) and high-pressure carbon monoxide disproportionation (HiPCO). Among these arc discharge, laser ablation are batch by batch process, Chemical Vapor Deposition can be used both for batch by batch or continuous processes,[83][84] and HiPCO is gas phase continuous process.[85] Most of these processes take place in a vacuum or with process gases. The CVD growth method is popular, as it yields high quantity and has a degree of control over diameter, length and morphology. Using particulate catalysts, large quantities of nanotubes can be synthesized by these methods, and industrialisation is well on its way, with several CNT and CNT fibers factory around the world. One problem of CVD processes is the high variability in the nanotube's characteristics [86] The HiPCO process advances in catalysis and continuous growth are making CNTs more commercially viable.[87] The HiPCO process helps in producing high purity single-walled carbon nanotubes in higher quantity. The HiPCO reactor operates at high temperature 900-1100 °C and high pressure ~30-50 bar.[88] It uses carbon monoxide as the carbon source and iron pentacarbonyl or nickel tetracarbonyl as a catalyst. These catalysts provide a nucleation site for the nanotubes to grow,[85] while cheaper iron-based catalysts like Ferrocene can be used for CVD process.
Vertically aligned carbon nanotube arrays are also grown by thermal chemical vapor deposition. A substrate (quartz, silicon, stainless steel, carbon fibers, etc.) is coated with a catalytic metal (Fe, Co, Ni) layer. Typically that layer is iron and is deposited via sputtering to a thickness of 1–5 nm. A 10–50 nm underlayer of alumina is often also put down on the substrate first. This imparts controllable wetting and good interfacial properties.When the substrate is heated to the growth temperature (~600 to 850 °C), the continuous iron film breaks up into small islands with each island then nucleating a carbon nanotube. The sputtered thickness controls the island size and this in turn determines the nanotube diameter. Thinner iron layers drive down the diameter of the islands and drive down the diameter of the nanotubes grown. The amount of time the metal island can sit at the growth temperature is limited as they are mobile and can merge into larger (but fewer) islands. Annealing at the growth temperature reduces the site density (number of CNT/mm2) while increasing the catalyst diameter.
The as-prepared carbon nanotubes always have impurities such as other forms of carbon (amorphous carbon, fullerene, etc.) and non-carbonaceous impurities (metal used for catalyst).[89][90] These impurities need to be removed to make use of the carbon nanotubes in applications.[91]
Purification
[edit]As-synthesized carbon nanotubes typically contain impurities and most importantly different chiralities of carbon nanotubes. Therefore, multiple methods have been developed to purify them including polymer-assisted,[92][93][94] density gradient ultracentrifugation (DGU),[95][96] chromatography [97][98][99] and aqueous two-phase extraction (ATPE).[100][101][102][103] These methods have been reviewed in multiple articles.[104][105][106]
Certain polymers selectively disperse or wrap CNTs of a particular chirality, metallic character or diameter. For example, poly(phenylenevinylenes) disperses CNTs of specific diameters (0.75–0.84 nm) and polyfluorenes are highly selective for semiconducting CNTs. It involves mainly two steps, sonicate the mixture (CNTs and polymers in solvent), centrifuge and the supernatant are desired CNTs.
Density gradient ultracentrifugation is a method based on the density difference of CNTs, so that different components are layered in centrifuge tubes under centrifugal force. Chromatography-based methods include size exclusion (SEC), ion-exchange (IEX) and gel chromatography. For SEC, CNTs are separated due to the difference in size using a stationary phase with different pore size. As for IEX, the separation is achieved based on their differential adsorption and desorption onto chemically functionalized resins packed in an IEX column, so understanding the interaction between CNTs mixtures and resins is important. The first IEX is reported to separate DNA-SWCNTs.[107] Gel chromatography is based on the partition of CNTs between stationary and mobile phase, it's found semiconducting CNTs are more strongly attracted by gel than metallic CNTs.[108][109] While it shows potential, the current application is limited to the separation of semiconducting (n,m) species.
ATPE uses two water-soluble polymers such as polyethylene glycol (PEG) and dextran. When mixed, two immiscible aqueous phases form spontaneously, and each of the two phases shows a different affinity to CNTs. Partition depends on the solvation energy difference between two similar phases of microscale volumes. By changing the separation system or temperatures, and adding strong oxidants, reductants, or salts, the partition of CNTs species into the two phases can be adjusted.
Despite the progress that has been made to separate and purify CNTs, many challenges remain, such as the growth of chirality-controlled CNTs, so that no further purification is needed, or large-scale purification.
Advantages of monochiral CNTs
[edit]Monochiral CNTs have the advantage that they do contain less or no impurities, well-defined non-congested optical spectra. This allows to create for example CNT-based biosensors with higher sensitivity and selectivity.[110] For example, monochiral SWCNTs are necessary for multiplexed and ratiometric sensing schemes,[111][112] enhanced sensitivity [113] of biocompatibility.[114]
Functionalization
[edit]CNTs are known to have weak dispersibility in many solvents such as water as a consequence of strong intermolecular p–p interactions. This hinders the processability of CNTs in industrial applications. To tackle the issue, various techniques have been developed to modify the surface of CNTs in order to improve their stability and solubility in water. This enhances the processing and manipulation of insoluble CNTs rendering them useful for synthesizing innovative CNT nanofluids with impressive properties that are tunable for a wide range of applications.Chemical routes such as covalent functionalization have been studied extensively, which involves the oxidation of CNTs via strong acids (e.g. sulfuric acid, nitric acid, or a mixture of both) in order to set the carboxylic groups onto the surface of the CNTs as the final product or for further modification by esterification or amination. Free radical grafting is a promising technique among covalent functionalization methods, in which alkyl or aryl peroxides, substituted anilines, and diazonium salts are used as the starting agents.
Free radical grafting of macromolecules (as the functional group) onto the surface of CNTs can improve the solubility of CNTs compared to common acid treatments which involve the attachment of small molecules such as hydroxyl onto the surface of CNTs. The solubility of CNTs can be improved significantly by free-radical grafting because the large functional molecules facilitate the dispersion of CNTs in a variety of solvents even at a low degree of functionalization. Recently an innovative environmentally friendly approach has been developed for the covalent functionalization of multi-walled carbon nanotubes (MWCNTs) using clove buds. This approach is innovative and green because it does not use toxic and hazardous acids which are typically used in common carbon nanomaterial functionalization procedures. The MWCNTs are functionalized in one pot using a free radical grafting reaction. The clove-functionalized MWCNTs are then dispersed in water producing a highly stable multi-walled carbon nanotube aqueous suspension (nanofluids).[115]
Modeling
[edit]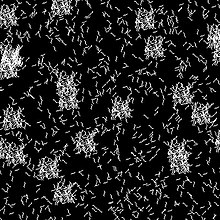
Carbon nanotubes are modelled in a similar manner as traditional composites in which a reinforcement phase is surrounded by a matrix phase. Ideal models such as cylindrical, hexagonal and square models are common. The size of the micromechanics model is highly function of the studied mechanical properties. The concept of representative volume element (RVE) is used to determine the appropriate size and configuration of the computer model to replicate the actual behavior of the CNT-reinforced nanocomposite. Depending on the material property of interest (thermal, electrical, modulus, creep), one RVE might predict the property better than the alternatives. While the implementation of the ideal model is computationally efficient, they do not represent microstructural features observed in scanning electron microscopy of actual nanocomposites. To incorporate realistic modeling, computer models are also generated to incorporate variability such as waviness, orientation and agglomeration of multiwall or single-wall carbon nanotubes.[116]
Metrology
[edit]There are many metrology standards and reference materials available for carbon nanotubes.[117]
For single-wall carbon nanotubes, ISO/TS 10868 describes a measurement method for the diameter, purity, and fraction of metallic nanotubes through optical absorption spectroscopy,[118] while ISO/TS 10797 and ISO/TS 10798 establish methods to characterize the morphology and elemental composition of single-wall carbon nanotubes, using transmission electron microscopy and scanning electron microscopy respectively, coupled with energy dispersive X-ray spectrometry analysis.[119][120]
NIST SRM 2483 is a soot of single-wall carbon nanotubes used as a reference material for elemental analysis, and was characterized using thermogravimetric analysis, prompt gamma activation analysis, induced neutron activation analysis, inductively coupled plasma mass spectroscopy, resonant Raman scattering, UV-visible-near infrared fluorescence spectroscopy and absorption spectroscopy, scanning electron microscopy, and transmission electron microscopy.[121][122] The Canadian National Research Council also offers a certified reference material SWCNT-1 for elemental analysis using neutron activation analysis and inductively coupled plasma mass spectroscopy.[117][123] NIST RM 8281 is a mixture of three lengths of single-wall carbon nanotube.[121][124]
For multiwall carbon nanotubes, ISO/TR 10929 identifies the basic properties and the content of impurities,[125] while ISO/TS 11888 describes morphology using scanning electron microscopy, transmission electron microscopy, viscometry, and light scattering analysis.[126] ISO/TS 10798 is also valid for multiwall carbon nanotubes.[120]
Chemical modification
[edit]Carbon nanotubes can be functionalized to attain desired properties that can be used in a wide variety of applications.[127] The two main methods of carbon nanotube functionalization are covalent and non-covalent modifications. Because of their apparent hydrophobic nature,[128] carbon nanotubes tend to agglomerate hindering their dispersion in solvents or viscous polymer melts. The resulting nanotube bundles or aggregates reduce the mechanical performance of the final composite. The surface of the carbon nanotubes can be modified to reduce the hydrophobicity and improve interfacial adhesion to a bulk polymer through chemical attachment.[11]
The surface of carbon nanotubes can be chemically modified by coating spinel nanoparticles by hydrothermal synthesis[129] and can be used for water oxidation purposes.[130]
In addition, the surface of carbon nanotubes can be fluorinated or halofluorinated by heating while in contact with a fluoroorganic substance, thereby forming partially fluorinated carbons (so-called Fluocar materials) with grafted (halo)fluoroalkyl functionality.[131][132]
Applications
[edit]
Carbon nanotubes are currently used in multiple industrial and consumer applications. These include battery components, polymer composites, to improve the mechanical, thermal and electrical properties of the bulk product, and as a highly absorptive black paint. Many other applications are under development, including field effect transistors for electronics, high-strength fabrics, biosensors for biomedical and agricultural applications, and many others.
Biosensing
[edit]SWCNTs have nanoscale dimensions that fit to the size of biological species. Due to this size compatibility and their large surface-to-volume ratio, they are sensitive to changes in their chemical environment.[133][134] Through covalent and non-covalent surface functionalization, SWCNTs can be precisely tailored for selective molecular interactions with a target analyte.[110] The SWCNT represents the transduction unit that converts the interaction into a signal change (optical or electrical). Due to continuous progress in the development of detection strategies, there are numerous examples of the use of SWCNTs as highly sensitive nanosensors (even down to the single molecule level[135][136][137]) for a variety of important biomolecules. Examples include the detection of reactive oxygen and nitrogen species,[138][139][140][141] neurotransmitters,[137][142][143][144][113] other small molecules,[145][146][147] lipids,[148][149] proteins,[150][151] sugars,[152][153] DNA/RNA,[154][155] enzymes[156][157] as well as bacteria.[158]
Potential future applications include biomedical and environmental applications such as monitoring plant health in agriculture,[138][139][159] standoff process control in bioreactors, research/diagnostics of neuronal communication[160] and numerous diseases such as coagulation disorders,[161] diabetes,[153][162] cancer,[163] microbial and viral infections,[158][164] testing the efficacy of pharmaceuticals[165] or infection monitoring using smart implants. In industry, SWCNTs are already used as sensors in the detection of gases and odors in the form of an electronic nose[166] or in enzyme screening.[167]

The signal change manifests itself in an increase or decrease in the current (electrical)[134] or in a change in the intensity or wavelength of the fluorescence emission (optical).[110] Depending on the type of application, both electrical or optical signal transmission can be advantageous.[168] For sensitive measurement of electronic changes, field-effect transistors (FET) are often used in which the flow of charges within the SWCNTs is measured. The FET structures allow easy on-chip integration and can be parallelized to detect multiple target analytes simultaneously.[147] However, such sensors are more invasive for in vivo applications, as the entire device has to be inserted into the body. Optical detection with semiconducting SWCNTs is based on the radiative recombination of excitons in the near-infrared (NIR) by prior optical (fluorescence[169]) or electrical excitation (electroluminescence[170][171]). The emission in the NIR enables detection in the biological transparency window, where optical sensor applications benefit from reduced scattering and autofluorescence of biological samples and consequently a high signal-to-noise ratio.[172] Compared to optical sensors in the UV or visible range, the penetration depth in biological tissue is also increased. In addition to the advantage of a contactless readout SWCNTs have excellent photostability,[173] which enables long-term sensor applications. Furthermore, the nanoscale size of SWCNTs allows dense coating of surfaces which enables chemical imaging, e.g. of cellular release processes with high spatial and temporal resolution.[137][113] Detection of several target analytes is possible by the spatial arrangement of different SWCNT sensors in arrays[158][174][175] or by hyperspectral detection[158][176] based on monochiral SWCNT sensors that emit at different emission wavelengths. For fluorescence applications, however, optical filters to distinguish between excitation and emission and a NIR-sensitive detector must be used. Standard silicon detectors can also be used if monochiral SWCNTs (extractable by special purification processes) emitting closer to the visible range (800 - 900 nm) are used.[113][177] In order to avoid susceptibility of optical sensors to fluctuating ambient light, internal references such as SWCNTs that are modified to be non-responsive or stable NIR emitters[158][178] can be used. An alternative is to measure fluorescence lifetimes[179] instead of fluorescence intensities. Overall, SWCNTs therefore have great potential as building blocks for various biosensors.To render SWCNTs suitable for biosensing, their surface needs to be modified to ensure colloidal stability and provide a handle for biological recognition. Therefore, biosensing and surface modifications (functionalization) are closely related.[110][180][181]
Other current applications
[edit]- Easton-Bell Sports, Inc. have been in partnership with Zyvex Performance Materials, using CNT technology in a number of their bicycle components – including flat and riser handlebars, cranks, forks, seatposts, stems and aero bars.
- Amroy Europe Oy manufactures Hybtonite carbon nano-epoxy resins where carbon nanotubes have been chemically activated to bond to epoxy, resulting in a composite material that is 20% to 30% stronger than other composite materials. It has been used for wind turbines, marine paints and a variety of sports gear such as skis, ice hockey sticks, baseball bats, hunting arrows, and surfboards.[182]
- Surrey NanoSystems synthesizes carbon nanotubes to create vantablack ultra-absorptive black paint.
- "Gecko tape" (also called "nano tape") is often commercially sold as double-sided adhesive tape. It can be used to hang lightweight items such as pictures and decorative items on smooth walls without punching holes in the wall. The carbon nanotube arrays comprising the synthetic setae leave no residue after removal and can stay sticky in extreme temperatures.[183]
- Tips for atomic force microscope probes.[184]
Applications under development
[edit]Applications of nanotubes in development in academia and industry include:
- Utilizing carbon nanotubes as the channel material of carbon nanotube field-effect transistors.[185]
- Using carbon nanotubes as a scaffold for diverse microfabrication techniques.[186]
- Energy dissipation in self-organized nanostructures under the influence of an electric field.[187]
- Using carbon nanotubes for environmental monitoring due to their active surface area and their ability to absorb gases.[188]
- Jack Andraka used carbon nanotubes in his pancreatic cancer test. His method of testing won the Intel International Science and Engineering Fair Gordon E. Moore Award in the spring of 2012.[189]
- The Boeing Company has patented the use of carbon nanotubes for structural health monitoring[190] of composites used in aircraft structures. This technology is hoped to greatly reduce the risk of an in-flight failure caused by structural degradation of aircraft.
- Zyvex Technologies has also built a 54' maritime vessel, the Piranha Unmanned Surface Vessel, as a technology demonstrator for what is possible using CNT technology. CNTs help improve the structural performance of the vessel, resulting in a lightweight 8,000 lb boat that can carry a payload of 15,000 lb over a range of 2,500 miles.[191]
- IMEC is using carbon nanotubes for pellicles in semiconductor lithography.[192]
- In tissue engineering, carbon nanotubes have been used as scaffolding for bone growth.[193]
Carbon nanotubes can serve as additives to various structural materials. For instance, nanotubes form a tiny portion of the material(s) in some (primarily carbon fiber) baseball bats, golf clubs, car parts, or damascus steel.[194][195]
IBM expected carbon nanotube transistors to be used on Integrated Circuits by 2020.[196]
Potential/Future applications
[edit]The strength and flexibility of carbon nanotubes makes them of potential use in controlling other nanoscale structures, which suggests they will have an important role in nanotechnology engineering.[197] The highest tensile strength of an individual multi-walled carbon nanotube has been tested to be 63 GPa.[3] Carbon nanotubes were found in Damascus steel from the 17th century, possibly helping to account for the legendary strength of the swords made of it.[198][199] Recently, several studies have highlighted the prospect of using carbon nanotubes as building blocks to fabricate three-dimensional macroscopic (>1mm in all three dimensions) all-carbon devices. Lalwani et al. have reported a novel radical initiated thermal crosslinking method to fabricated macroscopic, free-standing, porous, all-carbon scaffolds using single- and multi-walled carbon nanotubes as building blocks.[39] These scaffolds possess macro-, micro-, and nano- structured pores and the porosity can be tailored for specific applications. These 3D all-carbon scaffolds/architectures may be used for the fabrication of the next generation of energy storage, supercapacitors, field emission transistors, high-performance catalysis,[200] photovoltaics, and biomedical devices and implants.
CNTs are potential candidates for future via and wire material in nano-scale VLSI circuits. Eliminating electromigration reliability concerns that plague today's Cu interconnects, isolated (single and multi-wall) CNTs can carry current densities in excess of 1000 MA/cm2 without electromigration damage.[201]
Single-walled nanotubes are likely candidates for miniaturizing electronics. The most basic building block of these systems is an electric wire, and SWNTs with diameters of an order of a nanometre can be excellent conductors.[7][202] One useful application of SWNTs is in the development of the first intermolecular field-effect transistors (FET). The first intermolecular logic gate using SWCNT FETs was made in 2001.[203] A logic gate requires both a p-FET and an n-FET. Because SWNTs are p-FETs when exposed to oxygen and n-FETs otherwise, it is possible to expose half of an SWNT to oxygen and protect the other half from it. The resulting SWNT acts as a not logic gate with both p- and n-type FETs in the same molecule.
Large quantities of pure CNTs can be made into a freestanding sheet or film by surface-engineered tape-casting (SETC) fabrication technique which is a scalable method to fabricate flexible and foldable sheets with superior properties.[204][205] Another reported form factor is CNT fiber (a.k.a. filament) by wet spinning.[206] The fiber is either directly spun from the synthesis pot or spun from pre-made dissolved CNTs. Individual fibers can be turned into a yarn. Apart from its strength and flexibility, the main advantage is making an electrically conducting yarn. The electronic properties of individual CNT fibers (i.e. bundle of individual CNT) are governed by the two-dimensional structure of CNTs. The fibers were measured to have a resistivity only one order of magnitude higher than metallic conductors at 300 K (27 °C; 80 °F). By further optimizing the CNTs and CNT fibers, CNT fibers with improved electrical properties could be developed.[201][207]
CNT-based yarns are suitable for applications in energy and electrochemical water treatment when coated with an ion-exchange membrane.[208] Also, CNT-based yarns could replace copper as a winding material. Pyrhönen et al. (2015) have built a motor using CNT winding.[209][210]
Safety and health
[edit]
The National Institute for Occupational Safety and Health (NIOSH) is the leading United States federal agency conducting research and providing guidance on the occupational safety and health implications and applications of nanomaterials. Early scientific studies have indicated that nanoscale particles may pose a greater health risk than bulk materials due to a relative increase in surface area per unit mass. Increase in length and diameter of CNT is correlated to increased toxicity[211] and pathological alterations in lung.[212] The biological interactions of nanotubes are not well understood, and the field is open to continued toxicological studies. It is often difficult to separate confounding factors, and since carbon is relatively biologically inert, some of the toxicity attributed to carbon nanotubes may be instead due to residual metal catalyst contamination. In previous studies, only Mitsui-7 was reliably demonstrated to be carcinogenic, although for unclear/unknown reasons.[213] Unlike many common mineral fibers (such as asbestos), most SWCNTs and MWCNTs do not fit the size and aspect-ratio criteria to be classified as respirable fibers. In 2013, given that the long-term health effects have not yet been measured, NIOSH published a Current Intelligence Bulletin[214] detailing the potential hazards and recommended exposure limit for carbon nanotubes and fibers.[215] The U.S. National Institute for Occupational Safety and Health has determined non-regulatory recommended exposure limits (RELs) of 1 μg/m3 for carbon nanotubes and carbon nanofibers as background-corrected elemental carbon as an 8-hour time-weighted average (TWA) respirable mass concentration.[216] Although CNT caused pulmonary inflammation and toxicity in mice, exposure to aerosols generated from sanding of composites containing polymer-coated MWCNTs, representative of the actual end-product, did not exert such toxicity.[217]
As of October 2016, single-wall carbon nanotubes have been registered through the European Union's Registration, Evaluation, Authorization and Restriction of Chemicals (REACH) regulations, based on evaluation of the potentially hazardous properties of SWCNT. Based on this registration, SWCNT commercialization is allowed in the EU up to 100 metric tons.[218] Currently, the type of SWCNT registered through REACH is limited to the specific type of single-wall carbon nanotubes manufactured by OCSiAl, which submitted the application.[219]
History
[edit]The true identity of the discoverers of carbon nanotubes is a subject of some controversy.[220] A 2006 editorial written by Marc Monthioux and Vladimir Kuznetsov in the journal Carbon described the origin of the carbon nanotube.[221] A large percentage of academic and popular literature attributes the discovery of hollow, nanometre-size tubes composed of graphitic carbon to Sumio Iijima of NEC in 1991. His paper initiated a flurry of excitement and could be credited with inspiring the many scientists now studying applications of carbon nanotubes. Though Iijima has been given much of the credit for discovering carbon nanotubes, it turns out that the timeline of carbon nanotubes goes back much further than 1991.[220]
In 1952, L. V. Radushkevich and V. M. Lukyanovich published clear images of 50-nanometre diameter tubes made of carbon in the Journal of Physical Chemistry Of Russia.[222] This discovery was largely unnoticed, as the article was published in Russian, and Western scientists' access to Soviet press was limited during the Cold War. Monthioux and Kuznetsov mentioned in their Carbon editorial:[221]
The fact is, Radushkevich and Lukyanovich [...] should be credited for the discovery that carbon filaments could be hollow and have a nanometre-size diameter, that is to say for the discovery of carbon nanotubes.
In 1976, Morinobu Endo of CNRS observed hollow tubes of rolled up graphite sheets synthesised by a chemical vapour-growth technique.[2] The first specimens observed would later come to be known as single-walled carbon nanotubes (SWNTs).[223] Endo, in his early review of vapor-phase-grown carbon fibers (VPCF), also reminded us that he had observed a hollow tube, linearly extended with parallel carbon layer faces near the fiber core.[224] This appears to be the observation of multi-walled carbon nanotubes at the center of the fiber.[223] The mass-produced MWCNTs today are strongly related to the VPGCF developed by Endo.[223] In fact, they call it the "Endo-process", out of respect for his early work and patents.[223][225] In 1979, John Abrahamson presented evidence of carbon nanotubes at the 14th Biennial Conference of Carbon at Pennsylvania State University. The conference paper described carbon nanotubes as carbon fibers that were produced on carbon anodes during arc discharge. A characterization of these fibers was given, as well as hypotheses for their growth in a nitrogen atmosphere at low pressures.[226]
In 1981, a group of Soviet scientists published the results of chemical and structural characterization of carbon nanoparticles produced by a thermocatalytic disproportionation of carbon monoxide. Using TEM images and XRD patterns, the authors suggested that their "carbon multi-layer tubular crystals" were formed by rolling graphene layers into cylinders. They speculated that via this rolling, many different arrangements of graphene hexagonal nets are possible. They suggested two such possible arrangements: a circular arrangement (armchair nanotube); and a spiral, helical arrangement (chiral tube).[227]
In 1987, Howard G. Tennent of Hyperion Catalysis was issued a U.S. patent for the production of "cylindrical discrete carbon fibrils" with a "constant diameter between about 3.5 and about 70 nanometers..., length 102 times the diameter, and an outer region of multiple essentially continuous layers of ordered carbon atoms and a distinct inner core...."[228]
Helping to create the initial excitement associated with carbon nanotubes were Iijima's 1991 discovery of multi-walled carbon nanotubes in the insoluble material of arc-burned graphite rods;[1] and Mintmire, Dunlap, and White's independent prediction that if single-walled carbon nanotubes could be made, they would exhibit remarkable conducting properties.[7] Nanotube research accelerated greatly following the independent discoveries[13][14] by Iijima and Ichihashi at NEC and Bethune et al. at IBM of methods to specifically produce single-walled carbon nanotubes by adding transition-metal catalysts to the carbon in an arc discharge. Thess et al.[15] refined this catalytic method by vaporizing the carbon/transition-metal combination in a high-temperature furnace, which greatly improved the yield and purity of the SWNTs and made them widely available for characterization and application experiments. The arc discharge technique, well known to produce the famed Buckminsterfullerene on a preparative scale[clarify],[229] thus played a role in the discoveries of both multi- and single-wall nanotubes, extending the run of serendipitous discoveries relating to fullerenes. The discovery of nanotubes remains a contentious issue. Many believe that Iijima's report in 1991 is of particular importance because it brought carbon nanotubes into the awareness of the scientific community as a whole.[220][223]
In 2020, during an archaeological excavation of Keezhadi in Tamil Nadu, India, ~2600-year-old pottery was discovered whose coatings appear to contain carbon nanotubes. The robust mechanical properties of the nanotubes are partially why the coatings have lasted for so many years, say the scientists.[230]
See also
[edit]- Buckypaper
- Carbide-derived carbon
- Carbon nanocone
- Carbon nanofibers
- Carbon nanoscrolls
- Компьютер на углеродных нанотрубках
- Углеродные нанотрубки в фотовольтаике
- Огромная карбоновая трубка
- Алмазная нанонить
- Нитчатый углерод
- Молекулярное моделирование
- Наноцветок
- Нано-двутавр
- Ninithi (программное обеспечение для моделирования нанотрубок)
- Оптические свойства углеродных нанотрубок
- Органический полупроводник
Ссылки
[ редактировать ]Эта статья включает в себя цитируемый общедоступный текст Национального института наук о здоровье окружающей среды (NIEHS).
- ^ Перейти обратно: а б с Иидзима С. (7 ноября 1991 г.). «Спиральные микротрубочки графитового углерода». Природа . 354 (6348): 56–58. Бибкод : 1991Natur.354...56I . дои : 10.1038/354056a0 . S2CID 4302490 .
- ^ Перейти обратно: а б Оберлин А., Эндо М., Кояма Т. (март 1976 г.). «Нитевидный рост углерода за счет разложения бензола». Журнал роста кристаллов . 32 (3): 335–349. Бибкод : 1976JCrGr..32..335O . дои : 10.1016/0022-0248(76)90115-9 .
- ^ Перейти обратно: а б с Ю М.Ф., Лури О., Дайер М.Дж., Молони К., Келли Т.Ф., Руофф Р.С. (январь 2000 г.). «Прочность и механизм разрушения многостенных углеродных нанотрубок под действием растягивающей нагрузки». Наука . 287 (5453): 637–640. Бибкод : 2000Sci...287..637Y . дои : 10.1126/science.287.5453.637 . ПМИД 10649994 . S2CID 10758240 .
- ^ Садри Р., Ахмади Г., Тогун Х., Дахари М., Кази С.Н., Садегинежад Э. и др. (28 марта 2014 г.). «Экспериментальное исследование теплопроводности и вязкости наножидкостей, содержащих углеродные нанотрубки» . Письма о наномасштабных исследованиях . 9 (1): 151. Бибкод : 2014НРЛ.....9..151С . дои : 10.1186/1556-276X-9-151 . ПМК 4006636 . ПМИД 24678607 .
- ^ Бербер С., Квон Ю.К., Томанек Д. (май 2000 г.). «Необычайно высокая теплопроводность углеродных нанотрубок». Письма о физических отзывах . 84 (20): 4613–4616. arXiv : cond-mat/0002414 . Бибкод : 2000PhRvL..84.4613B . дои : 10.1103/PhysRevLett.84.4613 . ПМИД 10990753 . S2CID 9006722 .
- ^ Ким П., Ши Л., Маджумдар А., МакЮэн П.Л. (ноябрь 2001 г.). «Измерения теплопереноса отдельных многостенных нанотрубок». Письма о физических отзывах . 87 (21): 215502. arXiv : cond-mat/0106578 . Бибкод : 2001PhRvL..87u5502K . doi : 10.1103/PhysRevLett.87.215502 . ПМИД 11736348 . S2CID 12533685 .
- ^ Перейти обратно: а б с Минтмайр Дж.В., Данлэп Б.И., Уайт Коннектикут (февраль 1992 г.). «Являются ли фуллереновые трубочки металлическими?». Письма о физических отзывах . 68 (5): 631–634. Бибкод : 1992PhRvL..68..631M . doi : 10.1103/PhysRevLett.68.631 . ПМИД 10045950 .
- ^ Танс С.Дж., Деворет М.Х., Дай Х., Тесс А., Смолли Р.Э., Герлигс Л.Дж. и др. (апрель 1997 г.). «Отдельные одностенные углеродные нанотрубки как квантовые провода» . Природа . 386 (6624): 474–477. Бибкод : 1997Natur.386..474T . дои : 10.1038/386474a0 . S2CID 4366705 .
- ^ Хамада Н., Савада С.И., Осияма А. (март 1992 г.). «Новые одномерные проводники: графитовые микротрубочки». Письма о физических отзывах . 68 (10): 1579–1581. Бибкод : 1992PhRvL..68.1579H . doi : 10.1103/PhysRevLett.68.1579 . ПМИД 10045167 .
- ^ Уайлдер Дж.В., Венема Л.К., Ринцлер А.Г., Смолли Р.Э., Деккер С. (1 января 1998 г.). «Электронная структура атомно-разрешенных углеродных нанотрубок». Природа . 391 (6662): 59–62. Бибкод : 1998Natur.391...59W . дои : 10.1038/34139 . S2CID 205003208 .
- ^ Перейти обратно: а б Карусис Н., Тагматархис Н., Тасис Д. (сентябрь 2010 г.). «Текущий прогресс в химической модификации углеродных нанотрубок». Химические обзоры . 110 (9): 5366–5397. дои : 10.1021/cr100018g . ПМИД 20545303 .
- ^ Шарма М., Алессандро П., Чериямундат С., Лопус М. (2024). «Терапевтическое и диагностическое применение углеродных нанотрубок при раке: последние достижения и проблемы» . Журнал по борьбе с наркотиками . 32 (3): 287–299. дои : 10.1080/1061186X.2024.2309575 . ПМИД 38252035 .
- ^ Перейти обратно: а б Иидзима С., Ичихаши Т. (17 июня 1993 г.). «Однооболочечные углеродные нанотрубки диаметром 1 нм». Природа . 363 (6430): 603–605. Бибкод : 1993Natur.363..603I . дои : 10.1038/363603a0 . S2CID 4314177 .
- ^ Перейти обратно: а б Бетьюн Д.С., Кианг CH, Де Врис М.С., Горман Г., Савой Р., Васкес Дж. и др. (17 июня 1993 г.). «Кобальт-катализируемый рост углеродных нанотрубок с одноатомными слоями стенок». Природа . 363 (6430): 605–607. Бибкод : 1993Natur.363..605B . дои : 10.1038/363605a0 . S2CID 4321984 .
- ^ Перейти обратно: а б Тесс А., Ли Р., Николаев П., Дай Х., Пети П., Роберт Дж. и др. (июль 1996 г.). «Кристаллические веревки металлических углеродных нанотрубок». Наука . 273 (5274): 483–487. Бибкод : 1996Sci...273..483T . дои : 10.1126/science.273.5274.483 . ПМИД 8662534 . S2CID 13284203 .
- ^ Перейти обратно: а б Синнотт С.Б., Эндрюс Р. (июль 2001 г.). «Углеродные нанотрубки: синтез, свойства и применение». Критические обзоры по наукам о твердом теле и материалах . 26 (3): 145–249. Бибкод : 2001CRSSM..26..145S . дои : 10.1080/20014091104189 . S2CID 95444574 .
- ^ Чжао X, Лю Ю, Иноуэ С, Сузуки Т, Джонс РО, Андо Й (март 2004 г.). «Самая маленькая углеродная нанотрубка имеет диаметр 3 а» (PDF) . Письма о физических отзывах . 92 (12): 125502. Бибкод : 2004PhRvL..92l5502Z . doi : 10.1103/PhysRevLett.92.125502 . ПМИД 15089683 . Архивировано (PDF) из оригинала 9 октября 2022 года.
- ^ Торрес-Диас АС (2017). «От мезомасштабной к наномасштабной механике в одностенных углеродных нанотрубках» . Карбон . 123 : 145–150. Бибкод : 2017Carbo.123..145T . doi : 10.1016/j.carbon.2017.07.036 .
- ^ Хаяши Т., Ким Я.А., Матоба Т., Исака М., Нисимура К., Цукада Т. и др. (2003). «Самая маленькая отдельно стоящая одностенная углеродная нанотрубка». Нано-буквы . 3 (7): 887–889. Бибкод : 2003NanoL...3..887H . дои : 10.1021/nl034080r .
- ^ Гуан Л., Суэнага К., Иидзима С. (февраль 2008 г.). «Самая маленькая углеродная нанотрубка, присвоенная с точностью до атомного разрешения». Нано-буквы . 8 (2): 459–462. Бибкод : 2008NanoL...8..459G . дои : 10.1021/nl072396j . ПМИД 18186659 .
- ^ Чжан Р., Чжан Ю, Чжан Ц, Се Х, Цянь В, Вэй Ф (июль 2013 г.). «Выращивание углеродных нанотрубок полуметровой длины на основе распределения Шульца-Флори». АСУ Нано . 7 (7): 6156–6161. дои : 10.1021/nn401995z . ПМИД 23806050 .
- ^ Ван X, Ли Q, Се J, Цзинь Z, Ван J, Ли Y и др. (сентябрь 2009 г.). «Изготовление сверхдлинных и электрически однородных одностенных углеродных нанотрубок на чистых подложках». Нано-буквы . 9 (9): 3137–3141. Бибкод : 2009NanoL...9.3137W . CiteSeerX 10.1.1.454.2744 . дои : 10.1021/nl901260b . ПМИД 19650638 .
- ^ Джасти Р., Бхаттачарджи Дж., Нитон Дж.Б., Бертоцци Ч.Р. (декабрь 2008 г.). «Синтез, характеристика и теория [9]-, [12]- и [18] циклопарафенилена: углеродные нанокольцевые структуры» . Журнал Американского химического общества . 130 (52): 17646–17647. дои : 10.1021/ja807126u . ПМК 2709987 . ПМИД 19055403 .
- ^ Чунг К.Ю., Сегава Ю., Итами К. (ноябрь 2020 г.). «Синтетические стратегии углеродных нанолент и родственных ленточных полициклических ароматических углеводородов». Химия: Европейский журнал . 26 (65): 14791–14801. дои : 10.1002/chem.202002316 . ПМИД 32572996 . S2CID 219983922 .
- ^ «Самый плотный массив углеродных нанотрубок, выращенный на сегодняшний день» . КурцвейлАИ. 27 сентября 2013 г.
- ^ Сугиме Х., Эсконьяуреги С., Ян Дж., Д'Арси Л., Оливер Р.А., Бхардвадж С. и др. (12 августа 2013 г.). «Низкотемпературный рост лесов углеродных нанотрубок сверхвысокой плотности на проводящих подложках». Письма по прикладной физике . 103 (7): 073116. Бибкод : 2013ApPhL.103g3116S . дои : 10.1063/1.4818619 .
- ^ Дас С (март 2013 г.). «Обзор углеродных нанотрубок - новая эра нанотехнологий» (PDF) . Международный журнал новых технологий и передовой инженерии . 3 (3): 774–781. CiteSeerX 10.1.1.413.7576 . Архивировано (PDF) из оригинала 9 октября 2022 года.
- ^ Пяо И., Чен К.Ф., Грин А.А., Квон Х., Херсам М.С., Ли К.С. и др. (7 июля 2011 г.). «Оптические и электрические свойства внутренних трубок в углеродных нанотрубках с селективно функционализированными внешними стенками». Журнал физической химии . 2 (13): 1577–1582. дои : 10.1021/jz200687u .
- ^ Флао Э., Бакса Р., Пейни А., Лоран С. (июнь 2003 г.). «CCVD-синтез двустенных углеродных нанотрубок в граммовом масштабе» (PDF) . Химические коммуникации (12): 1442–1443. дои : 10.1039/b301514a . ПМИД 12841282 . S2CID 30627446 . Архивировано (PDF) из оригинала 9 октября 2022 года.
- ^ Камингс Дж., Зеттл А. (июль 2000 г.). «Наноразмерный линейный подшипник с низким коэффициентом трения, изготовленный из многостенных углеродных нанотрубок». Наука . 289 (5479): 602–604. Бибкод : 2000Sci...289..602C . CiteSeerX 10.1.1.859.7671 . дои : 10.1126/science.289.5479.602 . ПМИД 10915618 .
- ^ Трейси М.М., Эббесен Т.В., Гибсон Дж.М. (1996). «Исключительно высокий модуль Юнга наблюдается для отдельных углеродных нанотрубок». Природа . 381 (6584): 678–680. Бибкод : 1996Natur.381..678T . дои : 10.1038/381678a0 . S2CID 4332264 .
- ^ Завальнюк В, Марченко С (2011). «Теоретический анализ телескопических колебаний в многостенных углеродных нанотрубках» (PDF) . Физика низких температур . 37 (4): 337–342. arXiv : 0903.2461 . Бибкод : 2011LTP....37..337Z . дои : 10.1063/1.3592692 . S2CID 51932307 . Архивировано (PDF) из оригинала 9 октября 2022 года.
- ^ Чернозатонский Л.А. (1992). «Соединители из углеродных нанотрубок и плоские тренажеры в джунглях». Буквы по физике А. 172 (3): 173–176. Бибкод : 1992PhLA..172..173C . дои : 10.1016/0375-9601(92)90978-у .
- ^ Менон М., Шривастава Д. (1 декабря 1997 г.). «Т-переходы» углеродных нанотрубок: наноразмерные контактные устройства металл-полупроводник-металл». Письма о физических отзывах . 79 (22): 4453–4456. Бибкод : 1997PhRvL..79.4453M . дои : 10.1103/physrevlett.79.4453 .
- ^ Ламбин П. (1996). «Атомная структура и электронные свойства изогнутых углеродных нанотрубок». Синтез. Метр. 77 (1–3): 249–1254. дои : 10.1016/0379-6779(96)80097-x .
- ^ Ма КЛ (2011). «Электронно-транспортные свойства соединений между углеродными нанотрубками и графеновыми нанолентами». Европейский физический журнал Б. 83 (4): 487–492. Бибкод : 2011EPJB...83..487M . дои : 10.1140/epjb/e2011-20313-9 . S2CID 119497542 .
- ^ Харрис П.Дж., Суарес-Мартинес I, Маркс Н.А. (декабрь 2016 г.). «Структура соединений между углеродными нанотрубками и графеновыми оболочками» (PDF) . Наномасштаб . 8 (45): 18849–18854. дои : 10.1039/c6nr06461b . ПМИД 27808332 . S2CID 42241359 . Архивировано (PDF) из оригинала 9 октября 2022 года.
- ^ Димитракакис Г.К., Тилианакис Э., Фрудакис Г.Е. (октябрь 2008 г.). «Столбчатый графен: новая трехмерная сетевая наноструктура для улучшенного хранения водорода». Нано-буквы . 8 (10): 3166–3170. Бибкод : 2008NanoL...8.3166D . дои : 10.1021/nl801417w . ПМИД 18800853 .
- ^ Перейти обратно: а б Лалвани Г., Квачала А.Т., Канакия С., Патель С.С., Джудекс С., Ситхараман Б. (март 2013 г.). «Изготовление и характеристика трехмерных макроскопических цельноуглеродных каркасов» . Карбон . 53 : 90–100. doi : 10.1016/j.carbon.2012.10.035 . ПМЦ 3578711 . ПМИД 23436939 .
- ^ Лалвани Г., Гопалан А., Д'Агати М., Шанкаран Дж.С., Джудекс С., Цинь YX и др. (октябрь 2015 г.). «Пористые трехмерные каркасы из углеродных нанотрубок для тканевой инженерии» . Журнал исследований биомедицинских материалов. Часть А. 103 (10): 3212–3225. дои : 10.1002/jbm.a.35449 . ПМЦ 4552611 . ПМИД 25788440 .
- ^ Нойс С.Г., Ванфлит Р.Р., Крейгхед Х.Г., Дэвис Р.К. (март 2019 г.). «Углеродные микрокантилеверы с большой площадью поверхности» . Наномасштабные достижения . 1 (3): 1148–1154. Бибкод : 2019NanoA...1.1148N . дои : 10.1039/C8NA00101D . ПМЦ 9418787 . ПМИД 36133213 .
- ^ Насибулин А.Г., Пихица П.В., Цзян Х., Браун Д.П., Крашенинников А.В., Анисимов А.С. и др. (март 2007 г.). «Новый гибридный угольный материал» . Природные нанотехнологии . 2 (3): 156–161. Бибкод : 2007NatNa...2..156N . дои : 10.1038/nnano . ПМИД 18654245 .
- ^ Смит Б.В., Монтиу М., Луцци Д.Е. (1998). «Инкапсулированный C-60 в углеродные нанотрубки». Природа . 396 (6709): 323–324. Бибкод : 1998Natur.396R.323S . дои : 10.1038/24521 . S2CID 30670931 .
- ^ Смит Б.В., Луцци Д.Е. (2000). «Механизм формирования фуллереновых стручков и коаксиальных трубок: путь к крупномасштабному синтезу». хим. Физ. Летт . 321 (1–2): 169–174. Бибкод : 2000CPL...321..169S . дои : 10.1016/S0009-2614(00)00307-9 .
- ^ Су Х, Годдард В.А., Чжао Ю (2006). «Динамическая сила трения в генераторе из углеродных стручков» (PDF) . Нанотехнологии . 17 (22): 5691–5695. arXiv : cond-mat/0611671 . Бибкод : 2006Nanot..17.5691S . дои : 10.1088/0957-4484/17/22/026 . S2CID 18165997 . Архивировано (PDF) из оригинала 9 октября 2022 года.
- ^ Ван М., Ли СМ (январь 2010 г.). «Генератор в углеродном стручке, управляемый внешним электрическим полем: исследование молекулярной динамики». Нанотехнологии . 21 (3): 035704. Бибкод : 2010Nanot..21c5704W . дои : 10.1088/0957-4484/21/3/035704 . ПМИД 19966399 . S2CID 12358310 .
- ^ Перейти обратно: а б Лю Л., Го Г.И., Джаянти К.С., Ву С.Ю. (май 2002 г.). «Колоссальные парамагнитные моменты в металлических углеродных наноторах» . Письма о физических отзывах . 88 (21): 217206. Бибкод : 2002PhRvL..88u7206L . doi : 10.1103/PhysRevLett.88.217206 . ПМИД 12059501 .
- ^ Хухтала М., Куронен А., Каски К. (2002). «Структуры углеродных нанотрубок: моделирование молекулярной динамики на реалистичном пределе» (PDF) . Компьютерная физика. Коммуникации . 146 (1): 30–37. Бибкод : 2002CoPhC.146...30H . дои : 10.1016/S0010-4655(02)00432-0 . Архивировано из оригинала (PDF) 27 июня 2008 года.
- ^ Паркер CB, Раут А.С., Браун Б., Стоунер БР, Гласс Дж.Т. (2012). «Трехмерные массивы графеновых углеродных нанотрубок». Дж. Матер. Рез . 7. 27 (7): 1046–1053. Бибкод : 2012JMatR..27.1046P . дои : 10.1557/jmr.2012.43 . S2CID 137964473 .
- ^ Стоунер Б.Р., Гласс Дж.Т. (2012). «Углеродные наноструктуры: морфологическая классификация для оптимизации плотности заряда». Алмаз и родственные материалы . 23 : 130–134. Бибкод : 2012DRM....23..130S . дои : 10.1016/j.diamond.2012.01.034 .
- ^ Лю Ц, Жэнь В., Чен З.Г., Инь Л., Ли Ф., Конг Х. и др. (2009). «Полупроводниковые свойства чашечных углеродных нанотрубок» (PDF) . Карбон . 47 (3): 731–736. Бибкод : 2009Carbo..47..731L . doi : 10.1016/j.carbon.2008.11.005 . Архивировано из оригинала (PDF) 9 января 2015 года.
- ^ Перейти обратно: а б Пэн Б., Локасио М., Заполь П., Ли С., Мильке С.Л., Шац Г.К. и др. (октябрь 2008 г.). «Измерения почти предельной прочности многостенных углеродных нанотрубок и улучшение сшивки, вызванной облучением». Природные нанотехнологии . 3 (10): 626–631. дои : 10.1038/nnano.2008.211 . ПМИД 18839003 .
- ^ Коллинз П.Г., Авурис П. (декабрь 2000 г.). «Нанотрубки для электроники». Научный американец . 283 (6): 62–69. Бибкод : 2000SciAm.283f..62C . doi : 10.1038/scientificamerican1200-62 . ПМИД 11103460 .
- ^ Перейти обратно: а б Филлетер Т., Бернал Р., Ли С., Эспиноза Х.Д. (июль 2011 г.). «Сверхвысокая прочность и жесткость в пучках сшитых иерархических углеродных нанотрубок». Продвинутые материалы . 23 (25): 2855–2860. Бибкод : 2011AdM....23.2855F . дои : 10.1002/adma.201100547 . ПМИД 21538593 . S2CID 6363504 .
- ^ Дженсен К., Микельсон В., Кис А., Зеттл А. (26 ноября 2007 г.). «Измерение силы изгиба и перегиба на отдельных многостенных углеродных нанотрубках». Физический обзор B . 76 (19): 195436. Бибкод : 2007PhRvB..76s5436J . дои : 10.1103/PhysRevB.76.195436 .
- ^ Около С., Рафик Р., Икбал С.С., Сахарудин М.С., Инам Ф. (июнь 2020 г.). «Материалы из полиэтилена высокой плотности, армированные углеродными нанотрубками, для обшивки морских объектов» . Молекулы . 25 (13): 2960. doi : 10,3390/molecules25132960 . ПМЦ 7412307 . ПМИД 32605124 .
- ^ Лэрд Э.А., Куэммет Ф., Стил Г.А., Гроув-Расмуссен К., Найгорд Дж., Фленсберг К. и др. (2015). «Квантовый транспорт в углеродных нанотрубках» . Обзоры современной физики . 87 (3): 703–764. arXiv : 1403.6113 . Бибкод : 2015РвМП...87..703Л . дои : 10.1103/RevModPhys.87.703 . S2CID 119208985 .
- ^ Перейти обратно: а б Лу X, Чен Z (октябрь 2005 г.). «Искривленное пи-сопряжение, ароматичность и связанная с этим химия малых фуллеренов (<C60) и одностенных углеродных нанотрубок». Химические обзоры . 105 (10): 3643–3696. дои : 10.1021/cr030093d . ПМИД 16218563 .
- ^ Хон С., Мён С. (апрель 2007 г.). «Нанотрубная электроника: гибкий подход к мобильности». Природные нанотехнологии . 2 (4): 207–208. Бибкод : 2007NatNa...2..207H . дои : 10.1038/nnano.2007.89 . ПМИД 18654263 .
- ^ Василенко А., Винн Дж., Медейрос П.В., Моррис А.Дж., Слоан Дж., Куигли Д. (2017). «Инкапсулированные нанопровода: усиление электронного транспорта в углеродных нанотрубках». Физический обзор B . 95 (12): 121408. arXiv : 1611.04867 . Бибкод : 2017PhRvB..95l1408V . дои : 10.1103/PhysRevB.95.121408 . S2CID 59023024 .
- ^ Шарлье Дж.К., Блаз X, Рош С. (2007). «Электронные и транспортные свойства нанотрубок» (PDF) . Обзоры современной физики . 79 (2): 677–732. Бибкод : 2007РвМП...79..677С . дои : 10.1103/RevModPhys.79.677 .
- ^ Тан З.К., Чжан Л., Ван Н., Чжан XX, Вэнь Г.Х., Ли Г.Д. и др. (июнь 2001 г.). «Сверхпроводимость в одностенных углеродных нанотрубках с сопротивлением 4 ангстрема». Наука . 292 (5526): 2462–2465. Бибкод : 2001Sci...292.2462T . дои : 10.1126/science.1060470 . ПМИД 11431560 . S2CID 44987798 .
- ^ Такесуэ И., Харуяма Дж., Кобаяши Н., Чиаши С., Маруяма С., Сугай Т. и др. (февраль 2006 г.). «Сверхпроводимость в многостенных углеродных нанотрубках, полностью связанных концами». Письма о физических отзывах . 96 (5): 057001. arXiv : cond-mat/0509466 . Бибкод : 2006PhRvL..96e7001T . doi : 10.1103/PhysRevLett.96.057001 . ПМИД 16486971 . S2CID 119049151 .
- ^ Лорц Р., Чжан Ц., Ши В., Йе Дж.Т., Йе.Дж.Т., Цю С. и др. (май 2009 г.). «Сверхпроводящие характеристики композита углеродные нанотрубки-цеолит 4-А» . Труды Национальной академии наук Соединенных Штатов Америки . 106 (18): 7299–7303. дои : 10.1073/pnas.0813162106 . ПМЦ 2678622 . ПМИД 19369206 .
- ^ Бократ М. (1 марта 2006 г.). «Самое слабое звено». Физика природы . 2 (3): 155–156. дои : 10.1038/nphys252 . S2CID 125902065 .
- ^ Лю А.Т., Кунай Ю., Коттрилл А.Л., Каплан А., Чжан Г., Ким Х. и др. (июнь 2021 г.). «Электрохимия, индуцированная растворителем, на электрически асимметричной углеродной частице Януса» . Природные коммуникации . 12 (1): 3415. Бибкод : 2021NatCo..12.3415L . дои : 10.1038/s41467-021-23038-7 . ПМЦ 8184849 . ПМИД 34099639 . S2CID 235370395 .
- ^ Перейти обратно: а б Трафтон А (7 июня 2021 г.). «Инженеры MIT открыли совершенно новый способ производства электроэнергии» . СайТехДейли . Проверено 8 июня 2021 г.
- ^ Магнетизм на основе углерода: обзор магнетизма безметалловых соединений и материалов на основе углерода , Татьяна Макарова и Фернандо Паласио (ред.), Elsevier, 2006
- ^ Мисевич Дж.А., Мартель Р., Авурис П., Цанг Дж.К., Хайнце С., Терсофф Дж. (май 2003 г.). «Электрически индуцированная оптическая эмиссия полевого транзистора из углеродных нанотрубок». Наука . 300 (5620): 783–786. Бибкод : 2003Sci...300..783M . дои : 10.1126/science.1081294 . ПМИД 12730598 . S2CID 36336745 .
- ^ Чен Дж., Перебейнос В., Фрейтаг М., Цанг Дж., Фу Кью, Лю Дж. и др. (ноябрь 2005 г.). «Яркое инфракрасное излучение электрически индуцированных экситонов в углеродных нанотрубках». Наука . 310 (5751): 1171–1174. Бибкод : 2005Sci...310.1171C . дои : 10.1126/science.1119177 . ПМИД 16293757 . S2CID 21960183 .
- ^ Фрейтаг М., Мартин Ю., Мисевич Дж.А., Мартель Р., Авурис П. (2003). «Фотопроводимость одноуглеродных нанотрубок». Нано-буквы . 3 (8): 1067–1071. Бибкод : 2003NanoL...3.1067F . дои : 10.1021/nl034313e .
- ^ Иткис М.Э., Борондикс Ф., Ю.А., Хэддон Р.К. (апрель 2006 г.). «Болометрический инфракрасный фотоответ взвешенных пленок одностенных углеродных нанотрубок» . Наука . 312 (5772): 413–416. Бибкод : 2006Sci...312..413I . дои : 10.1126/science.1125695 . ПМИД 16627739 .
- ^ Стар А, Лу Ю, Брэдли К, Грюнер Г (2004). «Нанотрубные оптоэлектронные устройства памяти» . Нано-буквы . 4 (9): 1587–1591. Бибкод : 2004NanoL...4.1587S . дои : 10.1021/nl049337f .
- ^ Пол Черукури, Сергей М. Бачило, Сильвио Х. Литовский, Р. Брюс Вейсман (2004). «Ближняя инфракрасная флуоресцентная микроскопия одностенных углеродных нанотрубок в фагоцитирующих клетках». Журнал Американского химического общества . 126 (48): 15638–15639. дои : 10.1021/ja0466311 . ПМИД 15571374 .
- ^ Кевин Уэлшер, Сара П. Шерлок, Хунцзе Дай (2011). «Анатомическая визуализация глубоких тканей мышей с использованием флуорофоров из углеродных нанотрубок во втором ближнем инфракрасном окне» . Труды Национальной академии наук . 108 (22): 8943–8948. arXiv : 1105.3536 . Бибкод : 2011PNAS..108.8943W . дои : 10.1073/pnas.1014501108 . ПМК 3107273 . ПМИД 21576494 .
- ^ Пол В. Барон, Сынхён Байк, Дэниел А. Хеллер, Майкл С. Страно (2005). «Оптические сенсоры ближнего инфракрасного диапазона на основе одностенных углеродных нанотрубок». Природные материалы . 4 (1): 86–92. Бибкод : 2005NatMa...4...86B . дои : 10.1038/nmat1276 . ПМИД 15592477 . S2CID 43558342 .
- ^ Поп Э, Манн Д., Ван К., Гудсон К., Дай Х. (январь 2006 г.). «Теплопроводность отдельной одностенной углеродной нанотрубки при температуре выше комнатной». Нано-буквы . 6 (1): 96–100. arXiv : cond-mat/0512624 . Бибкод : 2006NanoL...6...96P . дои : 10.1021/nl052145f . ПМИД 16402794 . S2CID 14874373 .
- ^ Синха С., Барджами С., Яннаккьоне Г., Шваб А., Мюнх Г. (5 июня 2005 г.). «Внеосевые термические свойства пленок углеродных нанотрубок». Журнал исследований наночастиц . 7 (6): 651–657. Бибкод : 2005JNR.....7..651S . дои : 10.1007/s11051-005-8382-9 . S2CID 138479725 .
- ^ Козиол К.К., Янас Д., Браун Э., Хао Л. (1 апреля 2017 г.). «Термические свойства непрерывно формованных волокон из углеродных нанотрубок». Физика Э. 88 : 104–108. Бибкод : 2017PhyE...88..104K . дои : 10.1016/j.physe.2016.12.011 .
- ^ Куманек Б., Янас Д. (май 2019 г.). «Теплопроводность сетей углеродных нанотрубок: обзор» . Журнал материаловедения . 54 (10): 7397–7427. Бибкод : 2019JMatS..54.7397K . дои : 10.1007/s10853-019-03368-0 .
- ^ Тостенсон Э., Ли С., Чоу Т. (2005). «Нанокомпозиты в контексте». Композитные науки и технологии . 65 (3–4): 491–51. doi : 10.1016/j.compscitech.2004.11.003 .
- ^ Минго Н., Стюарт Д.А., Бройдо Д.А., Шривастава Д. (2008). «Передача фононов через дефекты в углеродных нанотрубках из первых принципов». Физ. Преподобный Б. 77 (3): 033418. Бибкод : 2008PhRvB..77c3418M . дои : 10.1103/PhysRevB.77.033418 . hdl : 1813/10898 .
- ^ Эндо М (октябрь 2004 г.). «Применение углеродных нанотрубок в двадцать первом веке». Философские труды Лондонского королевского общества. Серия А: Математические, физические и технические науки . 362 (1823): 2223–2238. дои : 10.1098/rsta.2004.1437 . ПМИД 15370479 . S2CID 20752554 .
- ^ Чжоу Цз (январь 2003 г.). «Производство более чистых двустенных углеродных нанотрубок в системе плавающего катализатора». Карбон . 41 (13): 2607–2611. Бибкод : 2003Carbo..41.2607Z . дои : 10.1016/S0008-6223(03)00336-1 .
- ^ Перейти обратно: а б Николаев П. (апрель 2004 г.). «Газофазное производство одностенных углеродных нанотрубок из монооксида углерода: обзор процесса Hipco». Журнал нанонауки и нанотехнологий . 4 (4): 307–316. дои : 10.1166/jnn.2004.066 . ПМИД 15296221 .
- ^ Шульц М.Ю., Шанов В.Н., Юн Ю. (2009). Наномедицинский дизайн частиц, датчиков, двигателей, имплантатов, роботов и устройств . Артех Хаус. ISBN 978-1-59693-280-7 .
- ^ Такеучи К., Хаяши Т., Ким Я.А., Фудзисава К., Эндо М. (февраль 2014 г.). «Современное состояние науки и применение углеродных нанотрубок» . Наносистемы: физика, химия, математика . 5 (1): 15–24.
- ^ Брониковски М.Дж., Уиллис П.А., Кольбер Д.Т., Смит К.А., Смолли Р.Э. (июль 2001 г.). «Газофазное производство углеродных одностенных нанотрубок из монооксида углерода с помощью процесса HiPco: параметрическое исследование». Журнал вакуумной науки и технологий A: Вакуум, поверхности и пленки . 19 (4): 1800–1805. Бибкод : 2001JVSTA..19.1800B . дои : 10.1116/1.1380721 . S2CID 3846517 .
- ^ Иткис М.Э., Переа Д.Е., Нийоги С., Рикард С.М., Хамон М.А., Ху Х. и др. (1 марта 2003 г.). «Оценка чистоты свежеприготовленной сажи одностенных углеродных нанотрубок с использованием ближней ИК-спектроскопии в растворенной фазе». Нано-буквы . 3 (3): 309–314. Бибкод : 2003NanoL...3..309I . дои : 10.1021/nl025926e .
- ^ Ван Л., Пумера М. (октябрь 2014 г.). «Остаточные металлические примеси внутри углеродных нанотрубок играют доминирующую роль в предположительно «безметалловых» реакциях восстановления кислорода». Химические коммуникации . 50 (84): 12662–12664. дои : 10.1039/C4CC03271C . ПМИД 25204561 .
- ^ Эатемади А., Дараи Х., Каримханлу Х., Коухи М., Заргами Н., Акбарзаде А. и др. (13 августа 2014 г.). «Углеродные нанотрубки: свойства, синтез, очистка и медицинское применение» . Письма о наномасштабных исследованиях . 9 (1): 393. Бибкод : 2014NRL.....9..393E . дои : 10.1186/1556-276X-9-393 . ПМК 4141964 . ПМИД 25170330 .
- ^ Чен Ф., Ван Б., Чен Ю, Ли Л.Дж. (1 октября 2007 г.). «На пути к извлечению отдельных видов одностенных углеродных нанотрубок с использованием полимеров на основе флуорена» . Нано-буквы . 7 (10): 3013–3017. Бибкод : 2007NanoL...7.3013C . дои : 10.1021/nl071349o . ISSN 1530-6984 . ПМИД 17867716 .
- ^ Ниш А., Хван Дж.Ю., Дойг Дж., Николас Р.Дж. (октябрь 2007 г.). «Высокоселективное диспергирование одностенных углеродных нанотрубок с использованием ароматических полимеров» . Природные нанотехнологии . 2 (10): 640–646. Бибкод : 2007NatNa...2..640N . дои : 10.1038/nnano.2007.290 . ISSN 1748-3395 . ПМИД 18654390 .
- ^ Лемассон Ф.А., Странк Т., Герстель П., Хенрих Ф., Лебедкин С., Барнер-Коволлик С. и др. (2 февраля 2011 г.). «Селективное диспергирование одностенных углеродных нанотрубок со специфическими хиральными индексами поли(N-децил-2,7-карбазолом)» . Журнал Американского химического общества . 133 (4): 652–655. дои : 10.1021/ja105722u . ISSN 0002-7863 . ПМИД 21171609 . S2CID 23209007 .
- ^ Арнольд М.С., Ступп С.И., Херсам MC (1 апреля 2005 г.). «Обогащение одностенных углеродных нанотрубок по диаметру в градиенте плотности» . Нано-буквы . 5 (4): 713–718. Бибкод : 2005NanoL...5..713A . дои : 10.1021/nl050133o . ISSN 1530-6984 . ПМИД 15826114 .
- ^ Грин А.А., Hersam MC (17 мая 2011 г.). «Почти однохиральные одностенные углеродные нанотрубки, полученные с помощью ортогонального итеративного ультрацентрифугирования в градиенте плотности» . Продвинутые материалы . 23 (19): 2185–2190. дои : 10.1002/adma.201100034 . ISSN 0935-9648 . ПМИД 21472798 . S2CID 5375678 .
- ^ Флавель Б.С., Каппес М.М., Крупке Р., Хенрих Ф. (23 апреля 2013 г.). «Разделение одностенных углеродных нанотрубок с помощью эксклюзионной хроматографии с участием 1-додеканола» . АСУ Нано . 7 (4): 3557–3564. дои : 10.1021/nn4004956 . ISSN 1936-0851 . ПМИД 23540203 .
- ^ Хуан X, Маклин Р.С., Чжэн М. (1 октября 2005 г.). «Сортировка по длине с высоким разрешением и очистка углеродных нанотрубок, обернутых ДНК, методом эксклюзионной хроматографии» . Аналитическая химия . 77 (19): 6225–6228. дои : 10.1021/ac0508954 . ISSN 0003-2700 . ПМИД 16194082 .
- ^ Мур К.Э., Пфол М., Хенрих Ф., Чакрадханула В.С., Кубель С., Каппес М.М. и др. (22 июля 2014 г.). «Разделение двустенных углеродных нанотрубок методом эксклюзионной колоночной хроматографии» . АСУ Нано . 8 (7): 6756–6764. дои : 10.1021/nn500756a . ISSN 1936-0851 . ПМИД 24896840 .
- ^ Ао Г, Хрипин С.Ю., Чжэн М (23 июля 2014 г.). «ДНК-контролируемое разделение углеродных нанотрубок в полимерных водных двухфазных системах» . Журнал Американского химического общества . 136 (29): 10383–10392. дои : 10.1021/ja504078b . ISSN 0002-7863 . ПМИД 24976036 .
- ^ Фаган Дж.А., Хрипин С.Ю., Сильвера Батиста К.А., Симпсон Дж.Р., Хароз Э.Х., Хайт Уокер А.Р. и др. (май 2014 г.). «Выделение конкретных видов одностенных углеродных нанотрубок малого диаметра посредством водной двухфазной экстракции» . Продвинутые материалы . 26 (18): 2800–2804. Бибкод : 2014AdM....26.2800F . дои : 10.1002/adma.201304873 . ISSN 0935-9648 . ПМИД 24448916 . S2CID 205253171 .
- ^ Лю М., Мини Б., Ян Дж., Ли Ю., Чжэн М. (26 декабря 2019 г.). «На пути к полному разрешению гибридов ДНК/углеродных нанотрубок водными двухфазными системами» . Журнал Американского химического общества . 141 (51): 20177–20186. дои : 10.1021/jacs.9b09953 . ISSN 0002-7863 . ПМИД 31783712 . S2CID 208498347 .
- ^ Ли Х, Гордеев Г, Гаррити О, Райх С, Флавел БС (28 января 2019 г.). «Разделение одностенных углеродных нанотрубок малого диаметра в одну-три стадии с помощью водной двухфазной экстракции» . АСУ Нано . 13 (2): 2567–2578. дои : 10.1021/acsnano.8b09579 . ISSN 1936-0851 . ПМИД 30673278 . S2CID 59224819 .
- ^ Ян Ф, Ван М, Чжан Д, Ян Дж, Чжэн М, Ли Ю (11 марта 2020 г.). «Хиральные чистые углеродные нанотрубки: рост, сортировка и характеристика» . Химические обзоры . 120 (5): 2693–2758. doi : 10.1021/acs.chemrev.9b00835 . ISSN 0009-2665 . ПМИД 32039585 . S2CID 211071215 .
- ^ Янас Д. (21 декабря 2017 г.). «На пути к монохиральным углеродным нанотрубкам: обзор прогресса в сортировке одностенных углеродных нанотрубок» . Границы химии материалов . 2 (1): 36–63. дои : 10.1039/C7QM00427C . ISSN 2052-1537 .
- ^ Вэй Х, Ли С., Ван В., Чжан Икс, Чжоу В., Се С. и др. (май 2022 г.). «Последние достижения в разделении структур одностенных углеродных нанотрубок и их применение в оптике, электронике и оптоэлектронике» . Передовая наука . 9 (14): e2200054. дои : 10.1002/advs.202200054 . ISSN 2198-3844 . ПМЦ 9108629 . ПМИД 35293698 .
- ^ Чжэн М., Семке Э.Д. (1 мая 2007 г.). «Обогащение углеродных нанотрубок с одинарной хиральностью» . Журнал Американского химического общества . 129 (19): 6084–6085. дои : 10.1021/ja071577k . ISSN 0002-7863 . ПМИД 17458969 .
- ^ Лю Х, Нишиде Д, Танака Т, Катаура Х (10 мая 2011 г.). «Крупномасштабное однохиральное разделение одностенных углеродных нанотрубок с помощью простой гель-хроматографии» . Природные коммуникации . 2 (1): 309. Бибкод : 2011NatCo...2..309L . дои : 10.1038/ncomms1313 . ISSN 2041-1723 . ПМК 3113293 . ПМИД 21556063 .
- ^ Танака Т., Джин Х., Мията Ю., Фуджи С., Суга Х., Найто Ю. и др. (8 апреля 2009 г.). «Простое и масштабируемое разделение металлических и полупроводниковых углеродных нанотрубок на основе геля» . Нано-буквы . 9 (4): 1497–1500. Бибкод : 2009NanoL...9.1497T . дои : 10.1021/nl8034866 . ISSN 1530-6984 . ПМИД 19243112 .
- ^ Перейти обратно: а б с д Акерманн Дж., Меттерних Дж.Т., Гербертц С., Крусс С. (25 апреля 2022 г.). «Биосенсорство с помощью флуоресцентных углеродных нанотрубок» . Angewandte Chemie, международное издание . 61 (18): e202112372. дои : 10.1002/anie.202112372 . ISSN 1433-7851 . ПМЦ 9313876 . ПМИД 34978752 .
- ^ Нисслер Р., Курт Л., Ли Х., Спрейнат А., Кулеманн И., Флавел Б.С. и др. (27 апреля 2021 г.). «Обнаружение с помощью хирально чистых флуоресцентных углеродных нанотрубок ближнего инфракрасного диапазона» . Аналитическая химия . 93 (16): 6446–6455. дои : 10.1021/acs.analchem.1c00168 . ISSN 0003-2700 . ПМИД 33830740 .
- ^ Нисслер Р., Акерманн Дж., Ма К., Крусс С. (19 июля 2022 г.). «Перспективы флуоресцентных однохиральных биосенсоров на основе углеродных нанотрубок» . Аналитическая химия . 94 (28): 9941–9951. дои : 10.1021/acs.analchem.2c01321 . ISSN 0003-2700 . PMID 35786856 . S2CID 250283972 .
- ^ Перейти обратно: а б с д Акерманн Дж., Стегеманн Дж., Смола Т., Регер Э., Юнг С., Шмитц А. и др. (апрель 2023 г.). «Высокочувствительная визуализация флуоресцентных наносенсоров в ближнем инфракрасном диапазоне» . Маленький . 19 (14): e2206856. дои : 10.1002/smll.202206856 . ISSN 1613-6810 . ПМИД 36610045 .
- ^ Надим А., Киндопп А., Уилли И., Юбер Л., Жубер Дж., Люсенте С. и др. (26 июля 2023 г.). «Повышение внутриклеточных оптических характеристик и стабильности инженерных наноматериалов посредством водной двухфазной очистки» . Нано-буквы . 23 (14): 6588–6595. Бибкод : 2023NanoL..23.6588N . doi : 10.1021/acs.nanolett.3c01727 . ISSN 1530-6984 . ПМК 11068083 . ПМИД 37410951 . S2CID 259356687 .
- ^ Садри Р., Хоссейни М., Кази С.Н., Багери С., Зубир Н., Соланги К.Х. и др. (октябрь 2017 г.). «Биологический простой подход к приготовлению водных суспензий ковалентно-функционализированных углеродных нанотрубок и их потенциал в качестве теплоносителей». Журнал коллоидной и интерфейсной науки . 504 : 115–123. Бибкод : 2017JCIS..504..115S . дои : 10.1016/j.jcis.2017.03.051 . ПМИД 28531649 .
- ^ Саней С.Х., Долес Р., Экайтис Т. (2019). «Влияние микроструктуры нанокомпозита на стохастические упругие свойства: исследование методом конечных элементов». Журнал ASCE-ASME о рисках и неопределенности в инженерных системах, Часть B: Машиностроение . 5 (3): 030903. дои : 10.1115/1.4043410 . S2CID 140766023 .
- ^ Перейти обратно: а б Стефаниак А.Б. (2017). «Основные показатели и инструменты для определения характеристик инженерных наноматериалов». В Мэнсфилде Э., Кайзере Д.Л., Фудзите Д., Ван де Вурде М. (ред.). Метрология и стандартизация нанотехнологий . Вайли-ВЧ Верлаг. стр. 151–174. дои : 10.1002/9783527800308.ch8 . ISBN 978-3-527-80030-8 .
- ^ «ISO/TS 10868:2017 – Нанотехнологии – Характеристика одностенных углеродных нанотрубок с использованием абсорбционной спектроскопии ультрафиолетового, видимого и ближнего инфракрасного диапазона (УФ-Вид-БИК)» . Международная организация по стандартизации . Архивировано из оригинала 7 сентября 2017 года . Проверено 6 сентября 2017 г.
- ^ «ISO/TS 10797:2012 – Нанотехнологии – Характеристика одностенных углеродных нанотрубок с использованием просвечивающей электронной микроскопии» . Международная организация по стандартизации . Архивировано из оригинала 7 сентября 2017 года . Проверено 6 сентября 2017 г.
- ^ Перейти обратно: а б «ISO/TS 10798:2011 – Нанотехнологии – Характеристика одностенных углеродных нанотрубок с использованием сканирующей электронной микроскопии и энергодисперсионного рентгеновского спектрометрического анализа» . Международная организация по стандартизации . Архивировано из оригинала 7 сентября 2017 года . Проверено 6 сентября 2017 г.
- ^ Перейти обратно: а б Фэган Дж. (5 марта 2009 г.). «Эталонные материалы углеродных нанотрубок» . США Национальный институт стандартов и технологий . Проверено 6 сентября 2017 г.
- ^ «SRM 2483 - Одностенные углеродные нанотрубки (сырая сажа)» . Национальный институт стандартов и технологий США . Архивировано из оригинала 18 февраля 2013 года . Проверено 6 сентября 2017 г.
- ^ «SWCNT-1: Сертифицированный эталонный материал с одностенными углеродными нанотрубками - Национальный исследовательский совет Канады» . Канадский национальный исследовательский совет . 7 ноября 2014 года . Проверено 6 сентября 2017 г.
- ^ «RM 8281 - Одностенные углеродные нанотрубки (дисперсные, три популяции с разрешением по длине)» . Национальный институт стандартов и технологий США . Архивировано из оригинала 1 апреля 2015 года . Проверено 6 сентября 2017 г.
- ^ «ISO/TR 10929:2012 – Нанотехнологии – Характеристика образцов многостенных углеродных нанотрубок (МУНТ)» . Международная организация по стандартизации . Архивировано из оригинала 7 сентября 2017 года . Проверено 6 сентября 2017 г.
- ^ «ISO/TS 11888:2017 – Нанотехнологии – Характеристика многостенных углеродных нанотрубок – Мезоскопические факторы формы» . Международная организация по стандартизации . Архивировано из оригинала 7 сентября 2017 года . Проверено 6 сентября 2017 г.
- ^ Бекьярова Е., Дэвис М., Берч Т., Иткис М.Е., Чжао Б., Саншайн С. и др. (9 октября 2004 г.). «Химически функционализированные одностенные углеродные нанотрубки как датчики аммиака» (PDF) . Дж. Физ. хим. Б. 108 (51). Вашингтон, округ Колумбия: Публикации ACS: 19717–19720. дои : 10.1021/jp0471857 . ISSN 1520-5207 . S2CID 96173424 .
- ^ Стандо Г, Лукавски Д, Лисецки Ф, Янас Д (январь 2019 г.). «Внутренний гидрофильный характер сетей углеродных нанотрубок» . Прикладная наука о поверхности . 463 : 227–233. Бибкод : 2019ApSS..463..227S . дои : 10.1016/j.apsusc.2018.08.206 . S2CID 105024629 .
- ^ Саху П., Шреста Р.Г., Шреста Л.К., Хилл Дж.П., Такей Т., Арига К. (ноябрь 2016 г.). «Поверхностные окисленные углеродные нанотрубки, равномерно покрытые наночастицами никель-феррита». Журнал неорганических и металлоорганических полимеров и материалов . 26 (6): 1301–1308. дои : 10.1007/s10904-016-0365-z . S2CID 101287773 .
- ^ Саху П., Тан Дж.Б., Чжан З.М., Сингх С.К., Лу Т.Б. (7 марта 2018 г.). «Разработка структуры поверхности бинарных/тройных наночастиц феррита как высокоэффективных электрокатализаторов для реакции выделения кислорода». ChemCatChem . 10 (5): 1075–1083. дои : 10.1002/cctc.201701790 . S2CID 104164617 .
- ^ US 10000382 , Задерко А., Василий Ю.А., «Способ модификации поверхности углеродных материалов фторуглеродами и их производными», выдан 19 июня 2018 г. Архивировано 17 сентября 2018 г. на Wayback Machine.
- ^ «WO16072959 Способ модификации поверхности углеродных материалов фторуглеродами и их производными» . Patentscope.wipo.int . Проверено 17 сентября 2018 г.
- ^ Когнет Л., Цыбульски Д.А., Роша Дж.Д., Дойл К.Д., Тур Дж.М., Вейсман Р.Б. (8 июня 2007 г.). «Ступенчатое тушение экситонной флуоресценции в углеродных нанотрубках с помощью одномолекулярных реакций» . Наука . 316 (5830): 1465–1468. arXiv : 0707.3246 . Бибкод : 2007Sci...316.1465C . дои : 10.1126/science.1141316 . ISSN 0036-8075 . ПМИД 17556581 . S2CID 7476534 .
- ^ Перейти обратно: а б Хеллер И., Янссенс А.М., Мянник Дж., Майнот Э.Д., Лемэй С.Г., Деккер С. (1 февраля 2008 г.). «Идентификация механизма биосенсорства с помощью транзисторов из углеродных нанотрубок» . Нано-буквы . 8 (2): 591–595. Бибкод : 2008NanoL...8..591H . дои : 10.1021/nl072996i . ISSN 1530-6984 . ПМИД 18162002 .
- ^ Чжан Дж., Богосян А.А., Барон П.В., Рвей А., Ким Дж.Х., Лин Д. и др. (26 января 2011 г.). «Обнаружение одиночных молекул оксида азота с помощью ДНК d(AT) 15, адсорбированной на флуоресцентных одностенных углеродных нанотрубках ближнего инфракрасного диапазона» . Журнал Американского химического общества . 133 (3): 567–581. дои : 10.1021/ja1084942 . ISSN 0002-7863 . ПМИД 21142158 .
- ^ Джин Х., Хеллер Д.А., Калбакова М., Ким Дж.Х., Чжан Дж., Богосян А.А. и др. (апрель 2010 г.). «Обнаружение передачи сигналов одиночных молекул H2O2 от рецептора эпидермального фактора роста с использованием флуоресцентных одностенных углеродных нанотрубок» . Природные нанотехнологии . 5 (4): 302–309. дои : 10.1038/nnano.2010.24 . ISSN 1748-3387 . ПМК 6438196 . ПМИД 20208549 .
- ^ Перейти обратно: а б с Крусс С., Салем Д.П., Вукович Л., Лима Б., Вандер Энде Э., Бойден Э.С. и др. (21 февраля 2017 г.). «Визуализация клеточного оттока дофамина с высоким разрешением с использованием флуоресцентной матрицы наносенсоров» . Труды Национальной академии наук . 114 (8): 1789–1794. Бибкод : 2017PNAS..114.1789K . дои : 10.1073/pnas.1613541114 . ISSN 0027-8424 . ПМЦ 5338365 . ПМИД 28179565 .
- ^ Перейти обратно: а б Ву Х, Нисслер Р., Моррис В., Херрманн Н., Ху П., Чон С.Дж. и др. (8 апреля 2020 г.). «Мониторинг здоровья растений с помощью флуоресцентных наносенсоров H 2 O 2 ближнего инфракрасного диапазона» . Нано-буквы . 20 (4): 2432–2442. Бибкод : 2020NanoL..20.2432W . дои : 10.1021/acs.nanolett.9b05159 . ISSN 1530-6984 . ПМИД 32097014 . S2CID 211524215 .
- ^ Перейти обратно: а б Лью Т.Т., Коман В.Б., Силмор К.С., Сео Дж.С., Гордийчук П., Квак С.Ю. и др. (15 апреля 2020 г.). «Обнаружение в режиме реального времени сигнальных волн H2O2, индуцированных раной, в растениях с помощью оптических наносенсоров» . Природные растения . 6 (4): 404–415. дои : 10.1038/s41477-020-0632-4 . ISSN 2055-0278 . ПМИД 32296141 . S2CID 215774820 .
- ^ Джин Х, Хеллер Д.А., Ким Дж.Х., Страно М.С. (10 декабря 2008 г.). «Стохастический анализ ступенчатых реакций тушения флуоресценции на одностенных углеродных нанотрубках: датчики одиночных молекул» . Нано-буквы . 8 (12): 4299–4304. Бибкод : 2008NanoL...8.4299J . дои : 10.1021/nl802010z . ISSN 1530-6984 . ПМИД 19367966 .
- ^ Хиральдо Дж.П., Лэндри М.П., Квак С.Ю., Джайн Р.М., Вонг М.Х., Айверсон Н.М. и др. (август 2015 г.). «Ратиометрический датчик, использующий однокиральные флуоресцентные углеродные нанотрубки ближнего инфракрасного диапазона: применение для мониторинга in vivo» . Маленький . 11 (32): 3973–3984. дои : 10.1002/smll.201403276 . ISSN 1613-6810 . ПМИД 25981520 . S2CID 44726670 .
- ^ Динарванд М., Нойберт Э., Мейер Д., Сельваджио Г., Манн Ф.А., Эрпенбек Л. и др. (11 сентября 2019 г.). «Визуализация высвобождения серотонина из клеток в ближнем инфракрасном диапазоне с помощью флуоресцентных наносенсоров» . Нано-буквы . 19 (9): 6604–6611. Бибкод : 2019NanoL..19.6604D . дои : 10.1021/acs.nanolett.9b02865 . ISSN 1530-6984 . ПМИД 31418577 . S2CID 201019834 .
- ^ Чон С., Ян Д., Бейен А.Г., Дель Бонис-О'Доннелл Дж.Т., Гест А.М., Наварро Н. и др. (6 декабря 2019 г.). «Высокопроизводительная эволюция наносенсоров серотонина ближнего инфракрасного диапазона» . Достижения науки . 5 (12): дааа3 Бибкод : 2019SciA.... 5.3771J дои : 10.1126/sciadv.aay3771 . ISSN 2375-2548 . ПМК 6920020 . ПМИД 31897432 .
- ^ Манохаран Г., Бёзель П., Тьен Дж., Хольтманспёттер М., Мейнгаст Л., Шмидт М. и др. (25 февраля 2023 г.). «Клик-функционализация силанизированных углеродных нанотрубок: от неорганических гетероструктур к биочувствительным наногибридам» . Молекулы . 28 (5): 2161. doi : 10,3390/molecules28052161 . ISSN 1420-3049 . ПМЦ 10004328 . ПМИД 36903408 .
- ^ Дель Бонис-О'Доннелл Дж.Т., Пиналс Р.Л., Чон С., Такрар А., Вулфингер Р.Д., Лэндри, член парламента (8 января 2019 г.). «Хемометрические подходы к разработке инфракрасных наносенсоров для изображения антрациклинов» . Биохимия . 58 (1): 54–64. doi : 10.1021/acs.biochem.8b00926 . ISSN 0006-2960 . ПМК 6411385 . ПМИД 30480442 .
- ^ Вонг М.Х., Хиральдо Дж.П., Квак С.Ю., Коман В.Б., Синклер Р., Лью Т.Т. и др. (февраль 2017 г.). «Обнаружение нитроароматических соединений и инфракрасная связь от растений дикого типа с использованием растительной нанобионики» . Природные материалы . 16 (2): 264–272. Бибкод : 2017NatMa..16..264W . дои : 10.1038/nmat4771 . ISSN 1476-1122 . ПМИД 27798623 .
- ^ Перейти обратно: а б Сюй X, Клемент П., Эклёф-Остерберг Дж., Келли-Локнейн Н., Мот-Поулсен К., Чавес Дж.Л. и др. (11 июля 2018 г.). «Реконфигурируемые мультиплексные сенсорные устройства из углеродных нанотрубок» . Нано-буквы . 18 (7): 4130–4135. Бибкод : 2018NanoL..18.4130X . дои : 10.1021/acs.nanolett.8b00856 . ISSN 1530-6984 . ПМИД 29923734 . S2CID 49310769 .
- ^ Йена П.В., Роксбери Д., Галасси Т.В., Аккари Л., Хорошко С.П., МАГАТЭ Д.Б. и др. (28 ноября 2017 г.). «Оптический репортер из углеродных нанотрубок отображает поток эндолизосомных липидов» . АСУ Нано . 11 (11): 10689–10703. дои : 10.1021/acsnano.7b04743 . ISSN 1936-0851 . ПМК 5707631 . ПМИД 28898055 .
- ^ Галасси Т.В., Йена П.В., Шах Дж., Ао Дж., Молитор Э., Брэм Ю. и др. (3 октября 2018 г.). «Оптический нанорепортер накопления эндолизосомных липидов демонстрирует устойчивое воздействие диеты на печеночные макрофаги in vivo» . Наука трансляционной медицины . 10 (461). doi : 10.1126/scitranslmed.aar2680 . ISSN 1946-6234 . ПМК 6543545 . ПМИД 30282694 .
- ^ Бискер Г., Донг Дж., Парк Х.Д., Айверсон Н.М., Ан Дж., Нельсон Дж.Т. и др. (8 января 2016 г.). «Молекулярное распознавание коронной фазы, нацеленное на белки» . Природные коммуникации . 7 (1): 10241. Бибкод : 2016NatCo...710241B . дои : 10.1038/ncomms10241 . ISSN 2041-1723 . ПМЦ 4729864 . ПМИД 26742890 .
- ^ Ким Дж., Кэмпбелл А.С., де Авила Б.Е., Ван Дж. (апрель 2019 г.). «Носимые биосенсоры для мониторинга здравоохранения» . Природная биотехнология . 37 (4): 389–406. дои : 10.1038/s41587-019-0045-y . ISSN 1087-0156 . ПМК 8183422 . ПМИД 30804534 .
- ^ Бароне П.В., Страно М.С. (11 декабря 2006 г.). «Обратимый контроль агрегации углеродных нанотрубок для датчика сродства к глюкозе» . Angewandte Chemie, международное издание . 45 (48): 8138–8141. дои : 10.1002/anie.200603138 . ISSN 1433-7851 . ПМИД 17099921 .
- ^ Перейти обратно: а б Зубков В., Ван Х., Шугерс Н., Венингер А., Глидер А., Каттанео С. и др. (2022). «Биоинженерия наносенсора глюкозооксидазы для непрерывного мониторинга глюкозы в ближнем инфракрасном диапазоне» . Наномасштабные достижения . 4 (11): 2420–2427. Бибкод : 2022NanoA...4.2420Z . дои : 10.1039/D2NA00092J . ISSN 2516-0230 . ПМК 9154020 . ПМИД 35746900 .
- ^ Харви Дж.Д., Йена П.В., Бейкер Х.А., Зерзе Г.Х., Уильямс Р.М., Галасси ТВ и др. (13 марта 2017 г.). «Репортер углеродных нанотрубок о событиях гибридизации микроРНК in vivo» . Природная биомедицинская инженерия . 1 (4). дои : 10.1038/s41551-017-0041 . ISSN 2157-846X . ПМК 5568023 . ПМИД 28845337 .
- ^ Харви Дж.Д., Бейкер Х.А., Ортис М.В., Кенцис А., Хеллер Д.А. (24 мая 2019 г.). «Обнаружение ВИЧ с помощью датчика РНК из углеродных нанотрубок» . Датчики СКУД . 4 (5): 1236–1244. doi : 10.1021/acsensors.9b00025 . ISSN 2379-3694 . ПМЦ 7556989 . ПМИД 31056899 .
- ^ Калмайер Н.Э., Абденнадер М.С., Агарвал С., Болдуин-Кордик Р., Хор Р.Л., Куистра А.С. и др. (23 марта 2021 г.). «Недорогие флуориметры ближнего инфракрасного диапазона: возможность перевода анализов на основе БИК в полевые условия» . Аналитическая химия . 93 (11): 4800–4808. дои : 10.1021/acs.analchem.0c03732 . ISSN 0003-2700 . ПМИД 33703890 . S2CID 232188200 .
- ^ Шумейко В., Палтиэль Ю., Бискер Г., Хаюка З., Шосеев О. (14 сентября 2020 г.). «Бумажный оптический биосенсор ближнего инфракрасного диапазона для количественного определения активности протеазы с использованием SWCNT, инкапсулированных в пептиды» . Датчики . 20 (18): 5247. Бибкод : 2020Senso..20.5247S . дои : 10.3390/s20185247 . ISSN 1424-8220 . ПМЦ 7570893 . ПМИД 32937986 .
- ^ Перейти обратно: а б с д и Нисслер Р., Бадер О., Домен М., Уолтер С.Г., Нолл С., Сельваджио Г. и др. (25 ноября 2020 г.). «Дистанционная ближняя инфракрасная идентификация возбудителей с помощью мультиплексированных наносенсоров» . Природные коммуникации . 11 (1): 5995. Бибкод : 2020NatCo..11.5995N . дои : 10.1038/s41467-020-19718-5 . ISSN 2041-1723 . ПМЦ 7689463 . ПМИД 33239609 .
- ^ Нисслер Р., Мюллер А.Т., Дорман Ф., Курт Л., Ли Х., Косио Э.Г. и др. (10 января 2022 г.). «Обнаружение и визуализация реакции растительных патогенов с помощью флуоресцентных полифенольных датчиков ближнего инфракрасного диапазона» . Angewandte Chemie, международное издание . 61 (2): e202108373. дои : 10.1002/anie.202108373 . ISSN 1433-7851 . ПМЦ 9298901 . ПМИД 34608727 .
- ^ Елизарова С., Шуайб А.А., Шаиб А., Хилл Б., Манн Ф., Броуз Н. и др. (31 мая 2022 г.). «Флуоресцентная наносенсорная краска обнаруживает высвобождение дофамина при варикозном расширении аксонов с высоким пространственно-временным разрешением» . Труды Национальной академии наук . 119 (22): e2202842119. Бибкод : 2022PNAS..11902842E . дои : 10.1073/pnas.2202842119 . ISSN 0027-8424 . ПМЦ 9295782 . ПМИД 35613050 .
- ^ Герстман Э., Хендлер-Ноймарк А., Вульф В., Бискер Г. (10 мая 2023 г.). «Мониторинг образования сгустков фибрина как части коагуляционного каскада с использованием флуоресцентных одностенных углеродных нанотрубок» . Прикладные материалы и интерфейсы ACS . 15 (18): 21866–21876. дои : 10.1021/acsami.3c00828 . ISSN 1944-8244 . ПМЦ 10176323 . ПМИД 37128896 .
- ^ Эрлих Р., Хендлер-Ноймарк А., Вульф В., Амир Д., Бискер Г. (июль 2021 г.). «Оптические наносенсоры для обратной связи в реальном времени о секреции инсулина β-клетками» . Маленький . 17 (30): e2101660. дои : 10.1002/smll.202101660 . ISSN 1613-6810 . ПМИД 34197026 .
- ^ Ким М., Чен С., Ван П., Малви Дж.Дж., Ян Ю., Вун С. и др. (17 марта 2022 г.). «Обнаружение рака яичников с помощью спектрального снятия отпечатков пальцев углеродных нанотрубок с квантовыми дефектами в сыворотке крови с помощью машинного обучения» . Природная биомедицинская инженерия . 6 (3): 267–275. дои : 10.1038/s41551-022-00860-y . ISSN 2157-846X . ПМЦ 9108893 . ПМИД 35301449 .
- ^ Меттерних Й.Т., Вартманн Й.А., Системих Л., Нисслер Р., Гербертц С., Крусс С. (12 июля 2023 г.). «Флуоресцентные биосенсоры ближнего инфракрасного диапазона на основе ковалентных якорей ДНК» . Журнал Американского химического общества . 145 (27): 14776–14783. дои : 10.1021/jacs.3c03336 . ISSN 0002-7863 . ПМИД 37367958 . S2CID 259261621 .
- ^ Акерманн Дж., Регер Э., Юнг С., Мор Дж., Гербертц С., Зайдль К. и др. (февраль 2024 г.). «Умные слайды для оптического мониторинга клеточных процессов» . Передовые функциональные материалы . 34 (6). дои : 10.1002/adfm.202309064 . ISSN 1616-301X .
- ^ «Умные нанотрубки – Разработка газового сенсора» . Умные нанотрубки . Проверено 9 февраля 2024 г.
- ^ "Добро пожаловать" . www.zymosense.com . Проверено 9 февраля 2024 г.
- ^ Клеман П., Акерманн Дж., Шахин-Сольмаз Н., Гербертц С., Боэро Г., Крусс С. и др. (ноябрь 2022 г.). «Сравнение электрических и оптических режимов трансдукции наносенсоров SWCNT, обернутых ДНК, для обратимого обнаружения нейромедиаторов» . Биосенсоры и биоэлектроника . 216 : 114642. дои : 10.1016/j.bios.2022.114642 . ПМИД 36055131 .
- ^ О'Коннелл М.Дж., Бачило С.М., Хаффман С.Б., Мур В.К., Страно М.С., Хароз Э.Х. и др. (26 июля 2002 г.). «Флуоресценция запрещенной зоны отдельных одностенных углеродных нанотрубок» . Наука . 297 (5581): 593–596. Бибкод : 2002Sci...297..593O . дои : 10.1126/science.1072631 . ISSN 0036-8075 . ПМИД 12142535 . S2CID 22623119 .
- ^ Сюй Б, Ву X, Ким М, Ван П, Ван Ю (28 января 2021 г.). «Электролюминесценция 4-нитроарильных органических центров окраски в полупроводниковых одностенных углеродных нанотрубках» . Журнал прикладной физики . 129 (4): 044305. Бибкод : 2021JAP...129d4305X . дои : 10.1063/5.0039047 . ISSN 0021-8979 .
- ^ Ли М.К., Риаз А., Ведерхак М., Финк К., Саха А., Дем С. и др. (23 августа 2022 г.). «Электролюминесценция одностенных углеродных нанотрубок с квантовыми дефектами» . АСУ Нано . 16 (8): 11742–11754. дои : 10.1021/acsnano.2c03083 . ISSN 1936-0851 . ОСТИ 1879407 . ПМИД 35732039 . S2CID 249956650 .
- ^ Хонг Джи, Антарис А.Л., Дай Х (10 января 2017 г.). «Флуорофоры ближнего инфракрасного диапазона для биомедицинской визуализации» . Природная биомедицинская инженерия . 1 (1). дои : 10.1038/s41551-016-0010 . ISSN 2157-846X . S2CID 78795936 .
- ^ Хун Джи, Диао С., Антарис А.Л., Дай Х. (14 октября 2015 г.). «Углеродные наноматериалы для биологической визуализации и наномедицинской терапии» . Химические обзоры . 115 (19): 10816–10906. doi : 10.1021/acs.chemrev.5b00008 . ISSN 0009-2665 . ПМИД 25997028 .
- ^ Донг Дж., Салем Д.П., Сунь Дж.Х., Страно М.С. (24 апреля 2018 г.). «Анализ мультиплексных массивов наносенсоров на основе флуоресцентных одностенных углеродных нанотрубок ближнего инфракрасного диапазона» . АСУ Нано . 12 (4): 3769–3779. дои : 10.1021/acsnano.8b00980 . ISSN 1936-0851 . ПМИД 29614219 .
- ^ Салем Д.П., Гонг Х, Лю А.Т., Акомби К., Страно М.С. (7 января 2020 г.). «Иммобилизация и функция nIR-флуоресцентных датчиков из углеродных нанотрубок на бумажных подложках для манипуляций с жидкостью» . Аналитическая химия . 92 (1): 916–923. дои : 10.1021/acs.analchem.9b03756 . ISSN 0003-2700 . ПМИД 31829619 . S2CID 209340238 .
- ^ Роксбери Д., Йена П.В., Уильямс Р.М., Эньеди Б., Нитхаммер П., Марсет С. и др. (21 сентября 2015 г.). «Гиперспектральная микроскопия флуоресценции ближнего инфракрасного диапазона позволяет получать изображения 17-хиральных углеродных нанотрубок» . Научные отчеты . 5 (1): 14167. Бибкод : 2015НатСР...514167Р . дои : 10.1038/srep14167 . ISSN 2045-2322 . ПМЦ 4585673 . ПМИД 26387482 .
- ^ Вэй X, Танака Т, Акизуки Н, Мияучи Ю, Мацуда К, Офучи М и др. (19 мая 2016 г.). «Разделение однохиральности и оптические свойства (5,4) одностенных углеродных нанотрубок» . Журнал физической химии C. 120 (19): 10705–10710. дои : 10.1021/acs.jpcc.6b03257 . ISSN 1932-7447 .
- ^ Сельваджио Г., Чижик А., Нисслер Р., Кулеманн Л., Мейер Д., Вуонг Л. и др. (20 марта 2020 г.). «Расслоенные флуоресцентные силикатные нанолисты ближнего инфракрасного диапазона для (био)фотоники» . Природные коммуникации . 11 (1): 1495. Бибкод : 2020NatCo..11.1495S . дои : 10.1038/s41467-020-15299-5 . ISSN 2041-1723 . ПМК 7083911 . ПМИД 32198383 .
- ^ Системич Л., Галонска П., Стегеманн Дж., Акерманн Дж., Крусс С. (12 июня 2023 г.). «Прижизненная флуоресцентная визуализация биомолекул с помощью углеродных нанотрубок в ближнем инфракрасном диапазоне**» . Angewandte Chemie, международное издание . 62 (24): e202300682. дои : 10.1002/anie.202300682 . ISSN 1433-7851 . ПМИД 36891826 .
- ^ Крусс С., Хилмер А.Дж., Чжан Дж., Руэл Н.Ф., Му Б., Страно М.С. (декабрь 2013 г.). «Углеродные нанотрубки как оптические биомедицинские сенсоры» . Обзоры расширенной доставки лекарств . 65 (15): 1933–1950. дои : 10.1016/j.addr.2013.07.015 . ПМИД 23906934 .
- ^ Богосян А.А., Чжан Дж., Барон П.В., Руэл Н.Ф., Ким Дж.Х., Хеллер Д.А. и др. (18 июля 2011 г.). «Флуоресцентные датчики ближнего инфракрасного диапазона на основе одностенных углеродных нанотрубок для биологических наук» . ChemSusChem . 4 (7): 848–863. Бибкод : 2011ЧСЧ...4..848Б . дои : 10.1002/cssc.201100070 . ISSN 1864-5631 . ПМИД 21751417 .
- ^ Паньи Дж. (5 марта 2010 г.). «Амрой стремится стать нанолидером» . Европейские новости пластмасс. Архивировано из оригинала 10 июля 2011 года.
- ^ «Лента из углеродных нанотрубок остается липкой при экстремальных температурах» . Информационный бюллетень Nanowerk . Американское химическое общество. 10 июля 2019 г.
- ^ «Советы по нанотрубкам» . инструменты нанонауки. Архивировано из оригинала 27 октября 2011 года.
- ^ Нойс С.Г., Доэрти Дж.Л., Ченг З., Хан Х., Боуэн С., Франклин А.Д. (март 2019 г.). «Электронная стабильность транзисторов из углеродных нанотрубок при длительном напряжении смещения». Нано-буквы . 19 (3): 1460–1466. Бибкод : 2019NanoL..19.1460N . дои : 10.1021/acs.nanolett.8b03986 . ПМИД 30720283 . S2CID 73450707 .
- ^ «Публикации по применению углеродных нанотрубок, включая микропроизводство каркасов» . nano.byu.edu . 27 мая 2014 г.
- ^ Белкин А., Хублер А., Безрядин А. (февраль 2015 г.). «Самособирающиеся колеблющиеся наноструктуры и принцип производства максимальной энтропии» . Научные отчеты . 5 : 8323. Бибкод : 2015NatSR...5E8323B . дои : 10.1038/srep08323 . ПМК 4321171 . ПМИД 25662746 .
- ^ Тан К.В., Тан К.Х., Онг Ю.Т., Мохамед А.Р., Зейн Ш., Тан Ш. (сентябрь 2012 г.). «Энергетическое и экологическое применение углеродных нанотрубок». Письма по экологической химии . 10 (3): 265–273. Бибкод : 2012EnvCL..10..265T . дои : 10.1007/s10311-012-0356-4 . S2CID 95369378 .
- ^ Такер А. «Джек Андрака, вундеркинд от рака поджелудочной железы» . Смитсоновский журнал . Проверено 2 марта 2021 г.
- ^ [1] US 9329021 , DeLuca MJ, Felker CJ, Heider D, «Система и методы для использования при мониторинге конструкции», опубликовано 3 мая 2016 г.
- ^ «Пирахна УСВ построена с использованием нанопрепрега из углеродного волокна» . ReinforcedPlastics.com. 19 февраля 2009 г. Архивировано из оригинала 3 марта 2012 г.
- ^ ЛаПедус М (22 марта 2021 г.). «EUV-пелликулы наконец готовы» . Полупроводниковая техника . Проверено 13 ноября 2022 г. .
- ^ Занелло Л.П., Чжао Б., Ху Х., Хэддон Р.К. (март 2006 г.). «Пролиферация костных клеток на углеродных нанотрубках». Нано-буквы . 6 (3): 562–567. Бибкод : 2006NanoL...6..562Z . дои : 10.1021/nl051861e . ПМИД 16522063 .
- ^ «Острота и прочность легендарных мечей благодаря нанотрубкам, говорится в исследовании» . news.nationalgeographic.com . Архивировано из оригинала 18 ноября 2006 года.
- ^ Гуллапалли С., Вонг М.С. (2011). «Нанотехнологии: Путеводитель по нанообъектам» (PDF) . Химический технологический прогресс . 107 (5): 28–32. Архивировано из оригинала (PDF) 13 августа 2012 года . Проверено 24 ноября 2011 г.
- ^ Симонайт Т. «IBM ожидает, что компьютерные чипы на нанотрубных транзисторах будут готовы вскоре после 2020 года» . Обзор технологий Массачусетского технологического института .
- ^ Томас DJ (июнь 2018 г.). «Сверхтонкая графитизированная наноструктурированная пряжа из МУНТ для изготовления электропроводящей ткани». Международный журнал передовых производственных технологий . 96 (9–12): 3805–3808. дои : 10.1007/s00170-017-1320-z . S2CID 115751858 .
- ^ Сандерсон К. (2006). «Острейший разрез меча из нанотрубок» . Новости природы . дои : 10.1038/news061113-11 . S2CID 136774602 .
- ^ Рейболд М., Пауфлер П., Левин А.А., Кохманн В., Пецке Н., Мейер Д.С. (ноябрь 2006 г.). «Материалы: углеродные нанотрубки в древней дамасской сабле» . Природа . 444 (7117): 286. Бибкод : 2006Natur.444..286R . дои : 10.1038/444286a . ПМИД 17108950 . S2CID 4431079 .
- ^ Валенти Г., Бони А., Мельчионна М., Карньелло М., Наси Л., Бертони Г. и др. (декабрь 2016 г.). «Коаксиальные гетероструктуры, объединяющие диоксид палладия / титана с углеродными нанотрубками для эффективного электрокаталитического выделения водорода» . Природные коммуникации . 7 : 13549. Бибкод : 2016NatCo...713549V . дои : 10.1038/ncomms13549 . ПМК 5159813 . ПМИД 27941752 .
- ^ Перейти обратно: а б Лиениг Дж., Тиле М. (2018). «Снижение электромиграции в физическом дизайне». Основы проектирования интегральных схем с учетом электромиграции . Спрингер. стр. 138–140. дои : 10.1007/978-3-319-73558-0 . ISBN 978-3-319-73557-3 .
- ^ Деккер С. (май 1999 г.). «Углеродные нанотрубки как молекулярные квантовые провода». Физика сегодня . 52 (5): 22–28. Бибкод : 1999PhT....52e..22D . дои : 10.1063/1.882658 .
- ^ Мартель Р., Дерик В., Лавуа С., Аппенцеллер Дж., Чан К.К., Терсофф Дж. и др. (декабрь 2001 г.). «Амбиполярный электрический транспорт в полупроводниковых одностенных углеродных нанотрубках». Письма о физических отзывах . 87 (25): 256805. Бибкод : 2001PhRvL..87y6805M . doi : 10.1103/PhysRevLett.87.256805 . ПМИД 11736597 .
- ^ Сусантёко Р.А., Карам З., Алхури С., Мустафа И., Ву Ч., Альмхейри С. (2017). «Техника изготовления ленточного литья с использованием поверхностной инженерии для коммерциализации отдельных листов углеродных нанотрубок». Журнал химии материалов А. 5 (36): 19255–19266. дои : 10.1039/c7ta04999d .
- ^ Карам З., Сусантёко Р.А., Альхаммади А., Мустафа И., Ву Ч., Альмхейри С. (июнь 2018 г.). «Разработка метода поверхностного литья на ленту для изготовления отдельно стоящих листов углеродных нанотрубок, содержащих наночастицы Fe 2 O 3 , для гибких батарей». Передовые инженерные материалы . 20 (6): 1701019. doi : 10.1002/adem.201701019 . S2CID 139283096 .
- ^ Бехабту Н., Янг К.С., Центалович Д.Е., Кляйнерман О., Ван Х., Ма А.В. и др. (январь 2013 г.). «Прочные, легкие, многофункциональные волокна из углеродных нанотрубок со сверхвысокой проводимостью». Наука . 339 (6116): 182–186. Бибкод : 2013Sci...339..182B . дои : 10.1126/science.1228061 . hdl : 1911/70792 . ПМИД 23307737 . S2CID 10843825 .
- ^ Пиро Л., Араужо Ф.А., Буи Т.Н., Отто М.Дж., Исси Дж.П. (26 августа 2015 г.). «Двумерный квантовый транспорт в волокнах из углеродных нанотрубок с высокой проводимостью». Физический обзор B . 92 (8): 085428. Бибкод : 2015PhRvB..92h5428P . doi : 10.1103/PhysRevB.92.085428 .
- ^ Лю Ф., Вагтервельд Р.М., Геббен Б., Отто М.Дж., Бишевель П.М., Хамелерс Х.В. (ноябрь 2014 г.). «Няжи из углеродных нанотрубок как прочные гибкие проводящие емкостные электроды» . Коллоидные и интерфейсные научные коммуникации . 3 :9–12. дои : 10.1016/j.colcom.2015.02.001 .
- ^ Пирхёнен Дж., Монтонен Дж., Линд П., Вотерен Дж., Отто М. (28 февраля 2015 г.). «Замена меди новыми углеродными наноматериалами в обмотках электрических машин». Международное обозрение электротехники . 10 (1): 12. CiteSeerX 10.1.1.1005.8294 . дои : 10.15866/iree.v10i1.5253 .
- ^ Пряжа из углеродных нанотрубок вращает электродвигатели в LUT . Ютуб
- ^ Фрейзер К., Кодали В., Янамала Н., Берч М.Э., Сина Л., Касуччио Г. и др. (декабрь 2020 г.). «Физико-химическая характеристика и генотоксичность широкого класса углеродных нанотрубок и нановолокон, используемых или производимых на предприятиях США» . Токсикология частиц и волокон . 17 (1): 62. дои : 10.1186/s12989-020-00392-w . ПМЦ 7720492 . PMID 33287860 .
- ^ Фрейзер К., Хаббс А., Янамала Н., Мерсер Р.Р., Стюкл Т.А., Дженсен Дж. и др. (декабрь 2021 г.). «Гистопатология широкого класса углеродных нанотрубок и нановолокон, используемых или производимых на предприятиях США, на мышиной модели» . Токсикология частиц и волокон . 18 (1): 47. дои : 10.1186/s12989-021-00440-z . ПМЦ 8686255 . ПМИД 34923995 .
- ^ Барбарино М., Джордано А. (март 2021 г.). «Оценка канцерогенности углеродных нанотрубок в дыхательной системе» . Раки . 13 (6): 1318. doi : 10.3390/cancers13061318 . ПМЦ 7998467 . ПМИД 33804168 .
- ^ «CDC — Нумерованные публикации NIOSH: текущие разведывательные бюллетени (CIB) — отсортированы по дате, в порядке убывания без номеров публикаций» . www.cdc.gov . Проверено 9 ноября 2022 г.
- ^ Ховард Дж. (апрель 2013 г.). «Профессиональное воздействие углеродных нанотрубок и нановолокон». Текущий разведывательный бюллетень . № 65. Публикация DHHS (NIOSH). дои : 10.26616/NIOSHPUB2013145 .
- ^ «Профессиональное воздействие углеродных нанотрубок и нановолокон» . Текущий разведывательный бюллетень . № 65. 14 июля 2020 г. doi : 10.26616/NIOSHPUB2013145 .
- ^ Бишоп Л., Сина Л., Орандл М., Янамала Н., Дам М.М., Берч М.Э. и др. (26 сентября 2017 г.). «Оценка токсичности in vivo профессиональных компонентов жизненного цикла углеродных нанотрубок для определения контекста потенциальных последствий для здоровья» . АСУ Нано . 11 (9): 8849–8863. дои : 10.1021/acsnano.7b03038 . ISSN 1936-0851 . ПМИД 28759202 .
- ^ «ЭКА ХИМ» . chem.echa.europa.eu . Проверено 10 июня 2024 г.
- ^ «Завершена регистрация REACH для одностенных углеродных нанотрубок» . pcimag.com . PCI Маг. 16 октября 2016 г. Архивировано из оригинала 24 ноября 2016 г. . Проверено 24 ноября 2016 г.
- ^ Перейти обратно: а б с Пасиос Пухадо М (2012). Углеродные нанотрубки как платформы для биосенсоров с электрохимическим и электронным преобразованием (Диссертация). Спрингеровские тезисы. Спрингер Гейдельберг. стр. XX, 208. doi : 10.1007/978-3-642-31421-6 . hdl : 10803/84001 . ISBN 978-3-642-31421-6 . S2CID 199491391 .
- ^ Перейти обратно: а б Монтиу М., Кузнецов В.Л. (август 2006 г.). «Кому следует отдать должное за открытие углеродных нанотрубок?» (PDF) . Карбон . 44 (9): 1621–1623. Бибкод : 2006Carbo..44.1621M . doi : 10.1016/j.carbon.2006.03.019 . Архивировано (PDF) из оригинала 9 октября 2022 года.
- ^ Radushkevich LV (1952). О Структуре Углерода, Образующегося При Термическом Разложении Окиси Углерода На Железном Контакте [О структуре углерода, образующегося при термическом разложении оксида углерода на контакте с железом] (PDF) . Журнал Физической Химии [ Журнал Физической Химии ] (на русском языке). 26 : 88–95. Архивировано из оригинала (PDF) 5 марта 2016 года . Проверено 5 апреля 2012 г.
- ^ Перейти обратно: а б с д и Эклунд ПК (2007). Отчет группы WTEC по итоговому отчету «Международная оценка исследований и разработок в области производства и применения углеродных нанотрубок» (PDF) (Отчет). Всемирный центр оценки технологий (WTEC). Архивировано из оригинала (PDF) 11 марта 2017 года . Проверено 5 августа 2015 г.
- ^ Оберлин А., Эндо М., Кояма Т. (март 1976 г.). «Нитечатый рост углерода за счет разложения бензола» (PDF) . Журнал роста кристаллов . 32 (3): 335–349. Бибкод : 1976JCrGr..32..335O . дои : 10.1016/0022-0248(76)90115-9 . Архивировано (PDF) из оригинала 9 октября 2022 года.
- ^ JP 1982-58,966 , Кояма Т., Эндо М.Т., «Способ производства углеродных волокон с помощью парофазного процесса», выпущен в 1983 г.
- ^ Абрахамсон Дж., Уайлс П.Г., Роудс Б.Л. (январь 1999 г.). «Структура углеродных волокон, обнаруженных на анодах угольной дуги». Карбон . 37 (11): 1873–1874. Бибкод : 1999Carbo..37.1873A . дои : 10.1016/S0008-6223(99)00199-2 .
- ^ Пропал без вести (1982). "Отсутствующий". Известия Академии наук СССР Металлы [ Известия Академии наук СССР. Металлы ] (на русском языке). 3 : 12–17. [ нужна полная цитата ]
- ^ US 4663230 , Tennent HG, «Углеродные фибриллы, способ их получения и содержащие их композиции», выдан 5 мая 1987 г.
- ^ Кречмер В., Лэмб Л.Д., Фостиропулос К.Х., Хаффман Д.Р. (1990). «Твердый C60: новая форма углерода». Природа . 347 (6291): 354–358. Бибкод : 1990Natur.347..354K . дои : 10.1038/347354a0 . S2CID 4359360 .
- ^ Кокарнесваран М., Сельварадж П., Ашокан Т., Перумал С., Селлаппан П., Муруган К.Д. и др. (ноябрь 2020 г.). «Открытие углеродных нанотрубок в керамике шестого века до нашей эры из Килади, Индия » Научные отчеты 10 (1): Бибкод : 2020NatSR..1019786K 19786. дои : 10.1038/s41598-020-76720-z . ПМЦ 7666134 . ПМИД 33188244 .
Внешние ссылки
[ редактировать ]
- Наноуглерод: от графена до бакиболлов . Интерактивные 3D-модели циклогексана, бензола, графена, графита, хиральных и нехиральных нанотрубок, а также бакиболлов C60 — WeCanFigureThisOut.org.
- C 60 и углеродные нанотрубки - короткое видео, объясняющее, как можно сделать нанотрубки из листов модифицированного графита, а также три различных типа нанотрубок, которые образуются.
- Учебный модуль по зонной структуре углеродных нанотрубок и нанолент
- Подборка бесплатных статей об углеродных нанотрубках.
- Демонстрационный проект WOLFRAM: Электронная зонная структура одностенной углеродной нанотрубки методом зонного сворачивания
- Демонстрационный проект WOLFRAM: электронная структура одностенной углеродной нанотрубки в представлении Ванье с сильной связью