Большой взрыв

Part of a series on |
Physical cosmology |
---|
![]() |
Большой взрыв — это физическая теория , описывающая, как Вселенная расширилась из начального состояния с высокой плотностью и температурой . [1] Впервые она была предложена в качестве физической теории в 1931 году римско-католическим священником и физиком Жоржем Леметром, когда он предположил, что Вселенная возникла из «первобытного атома». Различные космологические модели Большого взрыва объясняют эволюцию наблюдаемой Вселенной от самых ранних известных периодов до ее последующей крупномасштабной формы. [2] [3] [4] Эти модели предлагают всестороннее объяснение широкого спектра наблюдаемых явлений, включая обилие легких элементов , космическое микроволновое фоновое (CMB) излучение и крупномасштабную структуру . Однородность Вселенной, известная как проблема плоскостности , объясняется космической инфляцией : внезапным и очень быстрым расширением пространства в самые ранние моменты.
Crucially, these models are compatible with the Hubble–Lemaître law—the observation that the farther away a galaxy is, the faster it is moving away from Earth. Extrapolating this cosmic expansion backward in time using the known laws of physics, the models describe an increasingly concentrated cosmos preceded by a singularity in which space and time lose meaning (typically named "the Big Bang singularity").[5] Physics lacks a widely accepted theory of quantum gravity that can model the earliest conditions of the Big Bang. In 1964 the CMB was discovered, which convinced many cosmologists that the competing steady-state model of cosmic evolution was falsified, since the Big Bang models predict a uniform background radiation caused by high temperatures and densities in the distant past.[6] A wide range of empirical evidence strongly favors the Big Bang event, which is now essentially universally accepted.[7] Detailed measurements of the expansion rate of the universe place the Big Bang singularity at an estimated 13.787±0.020 billion years ago, which is considered the age of the universe.[8]
There remain aspects of the observed universe that are not yet adequately explained by the Big Bang models. After its initial expansion, the universe cooled sufficiently to allow the formation of subatomic particles, and later atoms. The unequal abundances of matter and antimatter that allowed this to occur is an unexplained effect known as baryon asymmetry. These primordial elements—mostly hydrogen, with some helium and lithium—later coalesced through gravity, forming early stars and galaxies. Astronomers observe the gravitational effects of an unknown dark matter surrounding galaxies. Most of the gravitational potential in the universe seems to be in this form, and the Big Bang models and various observations indicate that this excess gravitational potential is not created by baryonic matter, such as normal atoms. Measurements of the redshifts of supernovae indicate that the expansion of the universe is accelerating, an observation attributed to an unexplained phenomenon known as dark energy.[9]
Features of the models
The Big Bang models offer a comprehensive explanation for a broad range of observed phenomena, including the abundances of the light elements, the CMB, large-scale structure, and Hubble's law.[10] The models depend on two major assumptions: the universality of physical laws and the cosmological principle. The universality of physical laws is one of the underlying principles of the theory of relativity. The cosmological principle states that on large scales the universe is homogeneous and isotropic—appearing the same in all directions regardless of location.[11]
These ideas were initially taken as postulates, but later efforts were made to test each of them. For example, the first assumption has been tested by observations showing that the largest possible deviation of the fine-structure constant over much of the age of the universe is of order 10−5.[12] Also, general relativity has passed stringent tests on the scale of the Solar System and binary stars.[13][14][notes 1]
The large-scale universe appears isotropic as viewed from Earth. If it is indeed isotropic, the cosmological principle can be derived from the simpler Copernican principle, which states that there is no preferred (or special) observer or vantage point. To this end, the cosmological principle has been confirmed to a level of 10−5 via observations of the temperature of the CMB. At the scale of the CMB horizon, the universe has been measured to be homogeneous with an upper bound on the order of 10% inhomogeneity, as of 1995.[15]
Horizons
An important feature of the Big Bang spacetime is the presence of particle horizons. Since the universe has a finite age, and light travels at a finite speed, there may be events in the past whose light has not yet had time to reach earth. This places a limit or a past horizon on the most distant objects that can be observed. Conversely, because space is expanding, and more distant objects are receding ever more quickly, light emitted by us today may never "catch up" to very distant objects. This defines a future horizon, which limits the events in the future that we will be able to influence. The presence of either type of horizon depends on the details of the Friedmann–Lemaître–Robertson–Walker (FLRW) metric that describes the expansion of the universe.[16]
Our understanding of the universe back to very early times suggests that there is a past horizon, though in practice our view is also limited by the opacity of the universe at early times. So our view cannot extend further backward in time, though the horizon recedes in space. If the expansion of the universe continues to accelerate, there is a future horizon as well.[16]
Thermalization
Some processes in the early universe occurred too slowly, compared to the expansion rate of the universe, to reach approximate thermodynamic equilibrium. Others were fast enough to reach thermalization. The parameter usually used to find out whether a process in the very early universe has reached thermal equilibrium is the ratio between the rate of the process (usually rate of collisions between particles) and the Hubble parameter. The larger the ratio, the more time particles had to thermalize before they were too far away from each other.[17]
Timeline
According to the Big Bang models, the universe at the beginning was very hot and very compact, and since then it has been expanding and cooling.
Singularity
In the absence of a perfect cosmological principle, extrapolation of the expansion of the universe backwards in time using general relativity yields an infinite density and temperature at a finite time in the past.[18] This irregular behavior, known as the gravitational singularity, indicates that general relativity is not an adequate description of the laws of physics in this regime. Models based on general relativity alone cannot fully extrapolate toward the singularity.[5] In some proposals, such as the emergent Universe models, the singularity is replaced by another cosmological epoch. A different approach identifies the initial singularity as a singularity predicted by some models of the Big Bang theory to have existed before the Big Bang event.[19][clarification needed]
This primordial singularity is itself sometimes called "the Big Bang",[20] but the term can also refer to a more generic early hot, dense phase[21][notes 2] of the universe. In either case, "the Big Bang" as an event is also colloquially referred to as the "birth" of our universe since it represents the point in history where the universe can be verified to have entered into a regime where the laws of physics as we understand them (specifically general relativity and the Standard Model of particle physics) work. Based on measurements of the expansion using Type Ia supernovae and measurements of temperature fluctuations in the cosmic microwave background, the time that has passed since that event—known as the "age of the universe"—is 13.8 billion years.[22]
Despite being extremely dense at this time—far denser than is usually required to form a black hole—the universe did not re-collapse into a singularity. Commonly used calculations and limits for explaining gravitational collapse are usually based upon objects of relatively constant size, such as stars, and do not apply to rapidly expanding space such as the Big Bang. Since the early universe did not immediately collapse into a multitude of black holes, matter at that time must have been very evenly distributed with a negligible density gradient.[23]
Inflation and baryogenesis
The earliest phases of the Big Bang are subject to much speculation, given the lack of available data. In the most common models the universe was filled homogeneously and isotropically with a very high energy density and huge temperatures and pressures, and was very rapidly expanding and cooling. The period up to 10−43 seconds into the expansion, the Planck epoch, was a phase in which the four fundamental forces—the electromagnetic force, the strong nuclear force, the weak nuclear force, and the gravitational force, were unified as one.[24] In this stage, the characteristic scale length of the universe was the Planck length, 1.6×10−35 m, and consequently had a temperature of approximately 1032 degrees Celsius. Even the very concept of a particle breaks down in these conditions. A proper understanding of this period awaits the development of a theory of quantum gravity.[25][26] The Planck epoch was succeeded by the grand unification epoch beginning at 10−43 seconds, where gravitation separated from the other forces as the universe's temperature fell.[24]
At approximately 10−37 seconds into the expansion, a phase transition caused a cosmic inflation, during which the universe grew exponentially, unconstrained by the light speed invariance, and temperatures dropped by a factor of 100,000. This concept is motivated by the flatness problem, where the density of matter and energy is very close to the critical density needed to produce a flat universe. That is, the shape of the universe has no overall geometric curvature due to gravitational influence. Microscopic quantum fluctuations that occurred because of Heisenberg's uncertainty principle were "frozen in" by inflation, becoming amplified into the seeds that would later form the large-scale structure of the universe.[27] At a time around 10−36 seconds, the electroweak epoch begins when the strong nuclear force separates from the other forces, with only the electromagnetic force and weak nuclear force remaining unified.[28]
Inflation stopped locally at around 10−33 to 10−32 seconds, with the observable universe's volume having increased by a factor of at least 1078. Reheating followed as the inflaton field decayed, until the universe obtained the temperatures required for the production of a quark–gluon plasma as well as all other elementary particles.[29][30] Temperatures were so high that the random motions of particles were at relativistic speeds, and particle–antiparticle pairs of all kinds were being continuously created and destroyed in collisions.[1] At some point, an unknown reaction called baryogenesis violated the conservation of baryon number, leading to a very small excess of quarks and leptons over antiquarks and antileptons—of the order of one part in 30 million. This resulted in the predominance of matter over antimatter in the present universe.[31]
Cooling

The universe continued to decrease in density and fall in temperature, hence the typical energy of each particle was decreasing. Symmetry-breaking phase transitions put the fundamental forces of physics and the parameters of elementary particles into their present form, with the electromagnetic force and weak nuclear force separating at about 10−12 seconds.[28][32]
After about 10−11 seconds, the picture becomes less speculative, since particle energies drop to values that can be attained in particle accelerators. At about 10−6 seconds, quarks and gluons combined to form baryons such as protons and neutrons. The small excess of quarks over antiquarks led to a small excess of baryons over antibaryons. The temperature was no longer high enough to create either new proton–antiproton or neutron–antineutron pairs. A mass annihilation immediately followed, leaving just one in 108 of the original matter particles and none of their antiparticles.[33] A similar process happened at about 1 second for electrons and positrons. After these annihilations, the remaining protons, neutrons and electrons were no longer moving relativistically and the energy density of the universe was dominated by photons (with a minor contribution from neutrinos).
A few minutes into the expansion, when the temperature was about a billion kelvin and the density of matter in the universe was comparable to the current density of Earth's atmosphere, neutrons combined with protons to form the universe's deuterium and helium nuclei in a process called Big Bang nucleosynthesis (BBN).[34] Most protons remained uncombined as hydrogen nuclei.[35]
As the universe cooled, the rest energy density of matter came to gravitationally dominate that of the photon radiation. The recombination epoch began after about 379,000 years, when the electrons and nuclei combined into atoms (mostly hydrogen), which were able to emit radiation. This relic radiation, which continued through space largely unimpeded, is known as the cosmic microwave background.[35]
Structure formation

After the recombination epoch, the slightly denser regions of the uniformly distributed matter gravitationally attracted nearby matter and thus grew even denser, forming gas clouds, stars, galaxies, and the other astronomical structures observable today.[1] The details of this process depend on the amount and type of matter in the universe. The four possible types of matter are known as cold dark matter (CDM), warm dark matter, hot dark matter, and baryonic matter. The best measurements available, from the Wilkinson Microwave Anisotropy Probe (WMAP), show that the data is well-fit by a Lambda-CDM model in which dark matter is assumed to be cold. (Warm dark matter is ruled out by early reionization.)[37] This CDM is estimated to make up about 23% of the matter/energy of the universe, while baryonic matter makes up about 4.6%.[38]
In an "extended model" which includes hot dark matter in the form of neutrinos,[39] then the "physical baryon density" is estimated at 0.023. (This is different from the 'baryon density' expressed as a fraction of the total matter/energy density, which is about 0.046.) The corresponding cold dark matter density is about 0.11, and the corresponding neutrino density is estimated to be less than 0.0062.[38]
Cosmic acceleration
Independent lines of evidence from Type Ia supernovae and the CMB imply that the universe today is dominated by a mysterious form of energy known as dark energy, which appears to homogeneously permeate all of space. Observations suggest that 73% of the total energy density of the present day universe is in this form. When the universe was very young it was likely infused with dark energy, but with everything closer together, gravity predominated, braking the expansion. Eventually, after billions of years of expansion, the declining density of matter relative to the density of dark energy allowed the expansion of the universe to begin to accelerate.[9]
Dark energy in its simplest formulation is modeled by a cosmological constant term in Einstein field equations of general relativity, but its composition and mechanism are unknown. More generally, the details of its equation of state and relationship with the Standard Model of particle physics continue to be investigated both through observation and theory.[9]
All of this cosmic evolution after the inflationary epoch can be rigorously described and modeled by the lambda-CDM model of cosmology, which uses the independent frameworks of quantum mechanics and general relativity. There are no easily testable models that would describe the situation prior to approximately 10−15 seconds.[40] Understanding this earliest of eras in the history of the universe is one of the greatest unsolved problems in physics.
Concept history
Etymology
English astronomer Fred Hoyle is credited with coining the term "Big Bang" during a talk for a March 1949 BBC Radio broadcast,[41] saying: "These theories were based on the hypothesis that all the matter in the universe was created in one big bang at a particular time in the remote past."[42][43] However, it did not catch on until the 1970s.[43]
It is popularly reported that Hoyle, who favored an alternative "steady-state" cosmological model, intended this to be pejorative,[44][45][46] but Hoyle explicitly denied this and said it was just a striking image meant to highlight the difference between the two models.[47][48][50] Helge Kragh writes that the evidence for the claim that it was meant as a pejorative is "unconvincing", and mentions a number of indications that it was not a pejorative.[43]
The term itself has been argued to be a misnomer because it evokes an explosion.[43][51] The argument is that whereas an explosion suggests expansion into a surrounding space, the Big Bang only describes the intrinsic expansion of the contents of the universe.[52][53] Another issue pointed out by Santhosh Mathew is that bang implies sound, which is not an important feature of the model.[45] An attempt to find a more suitable alternative was not successful.[43][46]
Development
The Big Bang models developed from observations of the structure of the universe and from theoretical considerations. In 1912, Vesto Slipher measured the first Doppler shift of a "spiral nebula" (spiral nebula is the obsolete term for spiral galaxies), and soon discovered that almost all such nebulae were receding from Earth. He did not grasp the cosmological implications of this fact, and indeed at the time it was highly controversial whether or not these nebulae were "island universes" outside our Milky Way.[56][57] Ten years later, Alexander Friedmann, a Russian cosmologist and mathematician, derived the Friedmann equations from the Einstein field equations, showing that the universe might be expanding in contrast to the static universe model advocated by Albert Einstein at that time.[58]
In 1924, American astronomer Edwin Hubble's measurement of the great distance to the nearest spiral nebulae showed that these systems were indeed other galaxies. Starting that same year, Hubble painstakingly developed a series of distance indicators, the forerunner of the cosmic distance ladder, using the 100-inch (2.5 m) Hooker telescope at Mount Wilson Observatory. This allowed him to estimate distances to galaxies whose redshifts had already been measured, mostly by Slipher. In 1929, Hubble discovered a correlation between distance and recessional velocity—now known as Hubble's law.[59][60]
Independently deriving Friedmann's equations in 1927, Georges Lemaître, a Belgian physicist and Roman Catholic priest, proposed that the recession of the nebulae was due to the expansion of the universe.[61] He inferred the relation that Hubble would later observe, given the cosmological principle.[9] In 1931, Lemaître went further and suggested that the evident expansion of the universe, if projected back in time, meant that the further in the past the smaller the universe was, until at some finite time in the past all the mass of the universe was concentrated into a single point, a "primeval atom" where and when the fabric of time and space came into existence.[62]
In the 1920s and 1930s, almost every major cosmologist preferred an eternal steady-state universe, and several complained that the beginning of time implied by the Big Bang imported religious concepts into physics; this objection was later repeated by supporters of the steady-state theory.[63] This perception was enhanced by the fact that the originator of the Big Bang concept, Lemaître, was a Roman Catholic priest.[64] Arthur Eddington agreed with Aristotle that the universe did not have a beginning in time, viz., that matter is eternal. A beginning in time was "repugnant" to him.[65][66] Lemaître, however, disagreed:
If the world has begun with a single quantum, the notions of space and time would altogether fail to have any meaning at the beginning; they would only begin to have a sensible meaning when the original quantum had been divided into a sufficient number of quanta. If this suggestion is correct, the beginning of the world happened a little before the beginning of space and time.[67]
During the 1930s, other ideas were proposed as non-standard cosmologies to explain Hubble's observations, including the Milne model,[68] the oscillatory universe (originally suggested by Friedmann, but advocated by Albert Einstein and Richard C. Tolman)[69] and Fritz Zwicky's tired light hypothesis.[70]
After World War II, two distinct possibilities emerged. One was Fred Hoyle's steady-state model, whereby new matter would be created as the universe seemed to expand. In this model the universe is roughly the same at any point in time.[71] The other was Lemaître's Big Bang theory, advocated and developed by George Gamow, who introduced BBN[72] and whose associates, Ralph Alpher and Robert Herman, predicted the CMB.[73] Ironically, it was Hoyle who coined the phrase that came to be applied to Lemaître's theory, referring to it as "this big bang idea" during a BBC Radio broadcast in March 1949.[48][43][notes 3] For a while, support was split between these two theories. Eventually, the observational evidence, most notably from radio source counts, began to favor Big Bang over steady state. The discovery and confirmation of the CMB in 1964 secured the Big Bang as the best theory of the origin and evolution of the universe.[74]
In 1968 and 1970, Roger Penrose, Stephen Hawking, and George F. R. Ellis published papers where they showed that mathematical singularities were an inevitable initial condition of relativistic models of the Big Bang.[75][76] Then, from the 1970s to the 1990s, cosmologists worked on characterizing the features of the Big Bang universe and resolving outstanding problems. In 1981, Alan Guth made a breakthrough in theoretical work on resolving certain outstanding theoretical problems in the Big Bang models with the introduction of an epoch of rapid expansion in the early universe he called "inflation".[77] Meanwhile, during these decades, two questions in observational cosmology that generated much discussion and disagreement were over the precise values of the Hubble Constant[78] and the matter-density of the universe (before the discovery of dark energy, thought to be the key predictor for the eventual fate of the universe).[79]
In the mid-1990s, observations of certain globular clusters appeared to indicate that they were about 15 billion years old, which conflicted with most then-current estimates of the age of the universe (and indeed with the age measured today). This issue was later resolved when new computer simulations, which included the effects of mass loss due to stellar winds, indicated a much younger age for globular clusters.[80]
Significant progress in Big Bang cosmology has been made since the late 1990s as a result of advances in telescope technology as well as the analysis of data from satellites such as the Cosmic Background Explorer (COBE),[81] the Hubble Space Telescope and WMAP.[82] Cosmologists now have fairly precise and accurate measurements of many of the parameters of the Big Bang model, and have made the unexpected discovery that the expansion of the universe appears to be accelerating.[83][84]
Observational evidence
"[The] big bang picture is too firmly grounded in data from every area to be proved invalid in its general features."
The earliest and most direct observational evidence of the validity of the theory are the expansion of the universe according to Hubble's law (as indicated by the redshifts of galaxies), discovery and measurement of the cosmic microwave background and the relative abundances of light elements produced by Big Bang nucleosynthesis (BBN). More recent evidence includes observations of galaxy formation and evolution, and the distribution of large-scale cosmic structures,[86] These are sometimes called the "four pillars" of the Big Bang models.[87]
Precise modern models of the Big Bang appeal to various exotic physical phenomena that have not been observed in terrestrial laboratory experiments or incorporated into the Standard Model of particle physics. Of these features, dark matter is currently the subject of most active laboratory investigations.[88] Remaining issues include the cuspy halo problem[89] and the dwarf galaxy problem[90] of cold dark matter. Dark energy is also an area of intense interest for scientists, but it is not clear whether direct detection of dark energy will be possible.[91] Inflation and baryogenesis remain more speculative features of current Big Bang models. Viable, quantitative explanations for such phenomena are still being sought. These are unsolved problems in physics.
Hubble's law and the expansion of the universe

Observations of distant galaxies and quasars show that these objects are redshifted: the light emitted from them has been shifted to longer wavelengths. This can be seen by taking a frequency spectrum of an object and matching the spectroscopic pattern of emission or absorption lines corresponding to atoms of the chemical elements interacting with the light. These redshifts are uniformly isotropic, distributed evenly among the observed objects in all directions. If the redshift is interpreted as a Doppler shift, the recessional velocity of the object can be calculated. For some galaxies, it is possible to estimate distances via the cosmic distance ladder. When the recessional velocities are plotted against these distances, a linear relationship known as Hubble's law is observed:[59]where
- is the recessional velocity of the galaxy or other distant object,
- is the proper distance to the object, and
- is Hubble's constant, measured to be 70.4+1.3
−1.4 km/s/Mpc by the WMAP.[38]
Hubble's law implies that the universe is uniformly expanding everywhere. This cosmic expansion was predicted from general relativity by Friedmann in 1922[58] and Lemaître in 1927,[61] well before Hubble made his 1929 analysis and observations, and it remains the cornerstone of the Big Bang model as developed by Friedmann, Lemaître, Robertson, and Walker.
The theory requires the relation to hold at all times, where is the proper distance, is the recessional velocity, and , , and vary as the universe expands (hence we write to denote the present-day Hubble "constant"). For distances much smaller than the size of the observable universe, the Hubble redshift can be thought of as the Doppler shift corresponding to the recession velocity . For distances comparable to the size of the observable universe, the attribution of the cosmological redshift becomes more ambiguous, although its interpretation as a kinematic Doppler shift remains the most natural one.[92]
An unexplained discrepancy with the determination of the Hubble constant is known as Hubble tension. Techniques based on observation of the CMB suggest a lower value of this constant compared to the quantity derived from measurements based on the cosmic distance ladder.[93]
Cosmic microwave background radiation

In 1964, Arno Penzias and Robert Wilson serendipitously discovered the cosmic background radiation, an omnidirectional signal in the microwave band.[74] Their discovery provided substantial confirmation of the big-bang predictions by Alpher, Herman and Gamow around 1950. Through the 1970s, the radiation was found to be approximately consistent with a blackbody spectrum in all directions; this spectrum has been redshifted by the expansion of the universe, and today corresponds to approximately 2.725 K. This tipped the balance of evidence in favor of the Big Bang model, and Penzias and Wilson were awarded the 1978 Nobel Prize in Physics.
The surface of last scattering corresponding to emission of the CMB occurs shortly after recombination, the epoch when neutral hydrogen becomes stable. Prior to this, the universe comprised a hot dense photon-baryon plasma sea where photons were quickly scattered from free charged particles. Peaking at around 372±14 kyr,[37] the mean free path for a photon becomes long enough to reach the present day and the universe becomes transparent.

In 1989, NASA launched COBE, which made two major advances: in 1990, high-precision spectrum measurements showed that the CMB frequency spectrum is an almost perfect blackbody with no deviations at a level of 1 part in 104, and measured a residual temperature of 2.726 K (more recent measurements have revised this figure down slightly to 2.7255 K); then in 1992, further COBE measurements discovered tiny fluctuations (anisotropies) in the CMB temperature across the sky, at a level of about one part in 105.[81] John C. Mather and George Smoot were awarded the 2006 Nobel Prize in Physics for their leadership in these results.
During the following decade, CMB anisotropies were further investigated by a large number of ground-based and balloon experiments. In 2000–2001, several experiments, most notably BOOMERanG, found the shape of the universe to be spatially almost flat by measuring the typical angular size (the size on the sky) of the anisotropies.[98][99][100]
In early 2003, the first results of the Wilkinson Microwave Anisotropy Probe were released, yielding what were at the time the most accurate values for some of the cosmological parameters. The results disproved several specific cosmic inflation models, but are consistent with the inflation theory in general.[82] The Planck space probe was launched in May 2009. Other ground and balloon-based cosmic microwave background experiments are ongoing.
Abundance of primordial elements

Using Big Bang models, it is possible to calculate the expected concentration of the isotopes helium-4 (4He), helium-3 (3He), deuterium (2H), and lithium-7 (7Li) in the universe as ratios to the amount of ordinary hydrogen.[34] The relative abundances depend on a single parameter, the ratio of photons to baryons. This value can be calculated independently from the detailed structure of CMB fluctuations. The ratios predicted (by mass, not by abundance) are about 0.25 for 4He:H, about 10−3 for 2H:H, about 10−4 for 3He:H, and about 10−9 for 7Li:H.[34]
The measured abundances all agree at least roughly with those predicted from a single value of the baryon-to-photon ratio. The agreement is excellent for deuterium, close but formally discrepant for 4He, and off by a factor of two for 7Li (this anomaly is known as the cosmological lithium problem); in the latter two cases, there are substantial systematic uncertainties. Nonetheless, the general consistency with abundances predicted by BBN is strong evidence for the Big Bang, as the theory is the only known explanation for the relative abundances of light elements, and it is virtually impossible to "tune" the Big Bang to produce much more or less than 20–30% helium.[101] Indeed, there is no obvious reason outside of the Big Bang that, for example, the young universe before star formation, as determined by studying matter supposedly free of stellar nucleosynthesis products, should have more helium than deuterium or more deuterium than 3He, and in constant ratios, too.[102]: 182–185
Galactic evolution and distribution
Detailed observations of the morphology and distribution of galaxies and quasars are in agreement with the current Big Bang models. A combination of observations and theory suggest that the first quasars and galaxies formed within a billion years after the Big Bang,[103] and since then, larger structures have been forming, such as galaxy clusters and superclusters.[104]
Populations of stars have been aging and evolving, so that distant galaxies (which are observed as they were in the early universe) appear very different from nearby galaxies (observed in a more recent state). Moreover, galaxies that formed relatively recently appear markedly different from galaxies formed at similar distances but shortly after the Big Bang. These observations are strong arguments against the steady-state model. Observations of star formation, galaxy and quasar distributions and larger structures, agree well with Big Bang simulations of the formation of structure in the universe, and are helping to complete details of the theory.[104][105]
Primordial gas clouds
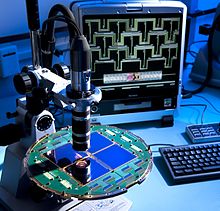
In 2011, astronomers found what they believe to be pristine clouds of primordial gas by analyzing absorption lines in the spectra of distant quasars. Before this discovery, all other astronomical objects have been observed to contain heavy elements that are formed in stars. Despite being sensitive to carbon, oxygen, and silicon, these three elements were not detected in these two clouds.[110][111] Since the clouds of gas have no detectable levels of heavy elements, they likely formed in the first few minutes after the Big Bang, during BBN.
Other lines of evidence
The age of the universe as estimated from the Hubble expansion and the CMB is now in agreement with other estimates using the ages of the oldest stars, both as measured by applying the theory of stellar evolution to globular clusters and through radiometric dating of individual Population II stars.[112] It is also in agreement with age estimates based on measurements of the expansion using Type Ia supernovae and measurements of temperature fluctuations in the cosmic microwave background.[22] The agreement of independent measurements of this age supports the Lambda-CDM (ΛCDM) model, since the model is used to relate some of the measurements to an age estimate, and all estimates turn agree. Still, some observations of objects from the relatively early universe (in particular quasar APM 08279+5255) raise concern as to whether these objects had enough time to form so early in the ΛCDM model.[113][114]
The prediction that the CMB temperature was higher in the past has been experimentally supported by observations of very low temperature absorption lines in gas clouds at high redshift.[115] This prediction also implies that the amplitude of the Sunyaev–Zel'dovich effect in clusters of galaxies does not depend directly on redshift. Observations have found this to be roughly true, but this effect depends on cluster properties that do change with cosmic time, making precise measurements difficult.[116][117]
Future observations
Future gravitational-wave observatories might be able to detect primordial gravitational waves, relics of the early universe, up to less than a second after the Big Bang.[118][119]
Problems and related issues in physics
As with any theory, a number of mysteries and problems have arisen as a result of the development of the Big Bang models. Some of these mysteries and problems have been resolved while others are still outstanding. Proposed solutions to some of the problems in the Big Bang model have revealed new mysteries of their own. For example, the horizon problem, the magnetic monopole problem, and the flatness problem are most commonly resolved with inflation theory, but the details of the inflationary universe are still left unresolved and many, including some founders of the theory, say it has been disproven.[120][121][122][123] What follows are a list of the mysterious aspects of the Big Bang concept still under intense investigation by cosmologists and astrophysicists.
Baryon asymmetry
It is not yet understood why the universe has more matter than antimatter.[31] It is generally assumed that when the universe was young and very hot it was in statistical equilibrium and contained equal numbers of baryons and antibaryons. However, observations suggest that the universe, including its most distant parts, is made almost entirely of normal matter, rather than antimatter. A process called baryogenesis was hypothesized to account for the asymmetry. For baryogenesis to occur, the Sakharov conditions must be satisfied. These require that baryon number is not conserved, that C-symmetry and CP-symmetry are violated and that the universe depart from thermodynamic equilibrium.[124] All these conditions occur in the Standard Model, but the effects are not strong enough to explain the present baryon asymmetry.
Dark energy
Measurements of the redshift–magnitude relation for type Ia supernovae indicate that the expansion of the universe has been accelerating since the universe was about half its present age. To explain this acceleration, general relativity requires that much of the energy in the universe consists of a component with large negative pressure, dubbed "dark energy".[9]
Dark energy, though speculative, solves numerous problems. Measurements of the cosmic microwave background indicate that the universe is very nearly spatially flat, and therefore according to general relativity the universe must have almost exactly the critical density of mass/energy. But the mass density of the universe can be measured from its gravitational clustering, and is found to have only about 30% of the critical density.[9] Since theory suggests that dark energy does not cluster in the usual way it is the best explanation for the "missing" energy density. Dark energy also helps to explain two geometrical measures of the overall curvature of the universe, one using the frequency of gravitational lenses,[125] and the other using the characteristic pattern of the large-scale structure--baryon acoustic oscillations--as a cosmic ruler.[126][127]
Negative pressure is believed to be a property of vacuum energy, but the exact nature and existence of dark energy remains one of the great mysteries of the Big Bang. Results from the WMAP team in 2008 are in accordance with a universe that consists of 73% dark energy, 23% dark matter, 4.6% regular matter and less than 1% neutrinos.[38] According to theory, the energy density in matter decreases with the expansion of the universe, but the dark energy density remains constant (or nearly so) as the universe expands. Therefore, matter made up a larger fraction of the total energy of the universe in the past than it does today, but its fractional contribution will fall in the far future as dark energy becomes even more dominant.[citation needed]
The dark energy component of the universe has been explained by theorists using a variety of competing theories including Einstein's cosmological constant but also extending to more exotic forms of quintessence or other modified gravity schemes.[128] A cosmological constant problem, sometimes called the "most embarrassing problem in physics", results from the apparent discrepancy between the measured energy density of dark energy, and the one naively predicted from Planck units.[129]
Dark matter

During the 1970s and the 1980s, various observations showed that there is not sufficient visible matter in the universe to account for the apparent strength of gravitational forces within and between galaxies. This led to the idea that up to 90% of the matter in the universe is dark matter that does not emit light or interact with normal baryonic matter. In addition, the assumption that the universe is mostly normal matter led to predictions that were strongly inconsistent with observations. In particular, the universe today is far more lumpy and contains far less deuterium than can be accounted for without dark matter. While dark matter has always been controversial, it is inferred by various observations: the anisotropies in the CMB, galaxy cluster velocity dispersions, large-scale structure distributions, gravitational lensing studies, and X-ray measurements of galaxy clusters.[130]
Indirect evidence for dark matter comes from its gravitational influence on other matter, as no dark matter particles have been observed in laboratories. Many particle physics candidates for dark matter have been proposed, and several projects to detect them directly are underway.[131]
Additionally, there are outstanding problems associated with the currently favored cold dark matter model which include the dwarf galaxy problem[90] and the cuspy halo problem.[89] Alternative theories have been proposed that do not require a large amount of undetected matter, but instead modify the laws of gravity established by Newton and Einstein; yet no alternative theory has been as successful as the cold dark matter proposal in explaining all extant observations.[132]
Horizon problem
The horizon problem results from the premise that information cannot travel faster than light. In a universe of finite age this sets a limit—the particle horizon—on the separation of any two regions of space that are in causal contact.[133] The observed isotropy of the CMB is problematic in this regard: if the universe had been dominated by radiation or matter at all times up to the epoch of last scattering, the particle horizon at that time would correspond to about 2 degrees on the sky. There would then be no mechanism to cause wider regions to have the same temperature.[102]: 191–202
A resolution to this apparent inconsistency is offered by inflation theory in which a homogeneous and isotropic scalar energy field dominates the universe at some very early period (before baryogenesis). During inflation, the universe undergoes exponential expansion, and the particle horizon expands much more rapidly than previously assumed, so that regions presently on opposite sides of the observable universe are well inside each other's particle horizon. The observed isotropy of the CMB then follows from the fact that this larger region was in causal contact before the beginning of inflation.[27]: 180–186
Heisenberg's uncertainty principle predicts that during the inflationary phase there would be quantum thermal fluctuations, which would be magnified to a cosmic scale. These fluctuations served as the seeds for all the current structures in the universe.[102]: 207 Inflation predicts that the primordial fluctuations are nearly scale invariant and Gaussian, which has been confirmed by measurements of the CMB.[82]: sec 6
A related issue to the classic horizon problem arises because in most standard cosmological inflation models, inflation ceases well before electroweak symmetry breaking occurs, so inflation should not be able to prevent large-scale discontinuities in the electroweak vacuum since distant parts of the observable universe were causally separate when the electroweak epoch ended.[134]
Magnetic monopoles
The magnetic monopole objection was raised in the late 1970s. Grand unified theories (GUTs) predicted topological defects in space that would manifest as magnetic monopoles. These objects would be produced efficiently in the hot early universe, resulting in a density much higher than is consistent with observations, given that no monopoles have been found. This problem is resolved by cosmic inflation, which removes all point defects from the observable universe, in the same way that it drives the geometry to flatness.[133]
Flatness problem

The flatness problem (also known as the oldness problem) is an observational problem associated with a FLRW.[133] The universe may have positive, negative, or zero spatial curvature depending on its total energy density. Curvature is negative if its density is less than the critical density; positive if greater; and zero at the critical density, in which case space is said to be flat. Observations indicate the universe is consistent with being flat.[135][136]
The problem is that any small departure from the critical density grows with time, and yet the universe today remains very close to flat.[notes 4] Given that a natural timescale for departure from flatness might be the Planck time, 10−43 seconds,[1] the fact that the universe has reached neither a heat death nor a Big Crunch after billions of years requires an explanation. For instance, even at the relatively late age of a few minutes (the time of nucleosynthesis), the density of the universe must have been within one part in 1014 of its critical value, or it would not exist as it does today.[137]
Misconceptions
One of the common misconceptions about the Big Bang model is that it fully explains the origin of the universe. However, the Big Bang model does not describe how energy, time, and space were caused, but rather it describes the emergence of the present universe from an ultra-dense and high-temperature initial state.[138] It is misleading to visualize the Big Bang by comparing its size to everyday objects. When the size of the universe at Big Bang is described, it refers to the size of the observable universe, and not the entire universe.[139]
Another common misconception is that the Big Bang must be understood as the expansion of space and not in terms of the contents of space exploding apart. In fact, either description can be accurate. The expansion of space (implied by the FLRW metric) is only a mathematical convention, corresponding to a choice of coordinates on spacetime. There is no generally covariant sense in which space expands.[140]
The recession speeds associated with Hubble's law are not velocities in a relativistic sense (for example, they are not related to the spatial components of 4-velocities). Therefore, it is not remarkable that according to Hubble's law, galaxies farther than the Hubble distance recede faster than the speed of light. Such recession speeds do not correspond to faster-than-light travel.
Many popular accounts attribute the cosmological redshift to the expansion of space. This can be misleading because the expansion of space is only a coordinate choice. The most natural interpretation of the cosmological redshift is that it is a Doppler shift.[92]
Implications
Given current understanding, scientific extrapolations about the future of the universe are only possible for finite durations, albeit for much longer periods than the current age of the universe. Anything beyond that becomes increasingly speculative. Likewise, at present, a proper understanding of the origin of the universe can only be subject to conjecture.[141]
Pre–Big Bang cosmology
The Big Bang explains the evolution of the universe from a starting density and temperature that is well beyond humanity's capability to replicate, so extrapolations to the most extreme conditions and earliest times are necessarily more speculative. Lemaître called this initial state the "primeval atom" while Gamow called the material "ylem". How the initial state of the universe originated is still an open question, but the Big Bang model does constrain some of its characteristics. For example, if specific laws of nature were to come to existence in a random way, inflation models show, some combinations of these are far more probable,[142] partly explaining why our Universe is rather stable. Another possible explanation for the stability of the Universe could be a hypothetical multiverse, which assumes every possible universe to exist, and thinking species could only emerge in those stable enough.[143] A flat universe implies a balance between gravitational potential energy and other energy forms, requiring no additional energy to be created.[135][136]
Теория Большого взрыва, построенная на уравнениях классической общей теории относительности, указывает на сингулярность в начале космического времени, и такая бесконечная плотность энергии может быть физически невозможной. Однако физические теории общей теории относительности и квантовой механики в том виде, в котором они реализованы в настоящее время, неприменимы до эпохи Планка, и исправление этого положения потребует разработки правильной трактовки квантовой гравитации. [18] Некоторые методы квантовой гравитации, такие как уравнение Уиллера-ДеВитта , предполагают, что время само по себе может быть эмерджентным свойством . [144] Таким образом, физики могут прийти к выводу, что времени до Большого взрыва не существовало. [145] [146]
Хотя неизвестно, что могло предшествовать горячему плотному состоянию ранней Вселенной, как и почему оно возникло, и даже являются ли такие вопросы разумными, спекуляции на тему «космогонии» изобилуют.
Вот некоторые умозрительные предложения в этом отношении, каждое из которых влечет за собой непроверенные гипотезы:
- Простейшие модели, в которых Большой взрыв был вызван квантовыми флуктуациями . У этого сценария было очень мало шансов на реализацию, но, согласно тоталитарному принципу , даже самое невероятное событие в конечном итоге произойдет. С нашей точки зрения, это произошло мгновенно из-за отсутствия ощущения времени до Большого взрыва. [147] [148] [149] [150]
- Модели эмерджентной Вселенной , которые характеризуют вечную эпоху низкой активности до Большого взрыва, напоминающую древние идеи о космическом яйце и рождении мира из первичного хаоса .
- Модели, в которых все пространство-время конечно, включая условие отсутствия границ Хартла-Хокинга . В этих случаях Большой взрыв представляет собой предел времени, но без сингулярности. [151] В таком случае Вселенная самодостаточна. [152]
- Модели бранной космологии , в которых инфляция обусловлена движением бран в теории струн ; модель до Большого взрыва; экпиротическая модель, в которой Большой взрыв является результатом столкновения бран; и циклическая модель , вариант экпиротической модели, в которой столкновения происходят периодически. В последней модели Большому взрыву предшествовало Большое сжатие, и Вселенная циклически переходит от одного процесса к другому. [153] [154] [155] [156]
- Вечная инфляция , при которой универсальная инфляция заканчивается локально здесь и там случайным образом, причем каждая конечная точка ведет к образованию пузыря вселенной , расширяющегося после собственного Большого взрыва. [157] [158]
Предложения в последних двух категориях рассматривают Большой взрыв как событие либо в гораздо большей и древней вселенной , либо в мультивселенной .
Окончательная судьба вселенной
До наблюдения темной энергии космологи рассматривали два сценария будущего Вселенной. Если бы массовая плотность Вселенной была больше критической плотности, то Вселенная достигла бы максимального размера, а затем начала бы коллапсировать. Он снова станет плотнее и горячее, закончив состояние, подобное тому, в котором оно началось, — Большое Сжатие . [16]
Альтернативно, если бы плотность Вселенной была равна критической плотности или ниже ее, расширение замедлилось бы, но никогда не остановилось. Звездообразование прекратится с расходованием межзвездного газа в каждой галактике; звезды сгорят, оставив после себя белые карлики , нейтронные звезды и черные дыры. Столкновения между ними приведут к накоплению массы во все более и более крупных черных дырах. Средняя температура Вселенной очень постепенно асимптотически приблизится к абсолютному нулю — к Большому Заморозку . [159] Более того, если бы протоны были нестабильны , то барионная материя исчезла бы, оставив только излучение и черные дыры. В конце концов, черные дыры испарятся, испустив излучение Хокинга . Энтропия . Вселенной увеличится до такой степени, что из нее невозможно будет извлечь организованную форму энергии – сценарий, известный как тепловая смерть [160]
Современные наблюдения ускоряющегося расширения предполагают, что все большая и большая часть видимой в настоящее время Вселенной будет выходить за пределы нашего горизонта событий и терять контакт с нами. Конечный результат неизвестен. Модель Вселенной ΛCDM содержит темную энергию в виде космологической постоянной. Эта теория предполагает, что только гравитационно связанные системы, такие как галактики, останутся вместе, и они тоже будут подвержены тепловой смерти по мере расширения и охлаждения Вселенной. Другие объяснения темной энергии, называемые теориями фантомной энергии , предполагают, что в конечном итоге скопления галактик, звезды, планеты, атомы, ядра и сама материя будут разорваны на части постоянно увеличивающимся расширением в так называемом Большом Разрыве . [161]
Религиозно-философские интерпретации
Как описание происхождения Вселенной, Большой Взрыв имеет важное значение для религии и философии. [162] [163] В результате это стало одной из самых оживленных областей в дискурсе между наукой и религией . [164] Некоторые полагают, что Большой Взрыв подразумевает творца. [165] [166] в то время как другие утверждают, что космология Большого взрыва делает понятие творца излишним. [163] [167]
См. также
- Антропный принцип - Гипотеза о разумной жизни и Вселенной.
- Big Bounce – Модель происхождения Вселенной
- Большое сжатие – теоретический сценарий окончательной судьбы Вселенной.
- Холодный Большой Взрыв – Обозначение абсолютного нуля температуры в начале Вселенной.
- Космический календарь - метод визуализации хронологии Вселенной.
- Космогония - отрасль науки или теория происхождения Вселенной.
- Эврика: Поэма в прозе - длинное научно-популярное произведение американского писателя Эдгара Аллана По, предположение о Большом взрыве.
- Будущее расширяющейся Вселенной - Сценарий будущего, будет ли расширение Вселенной продолжаться вечно или нет.
- Тепловая смерть Вселенной – Возможная судьба Вселенной. Также известен как «Большой холод» и «Большое замораживание».
- Нестандартная космология - модели Вселенной, которые отклоняются от действующего на тот момент научного консенсуса.
- Форма Вселенной - Локальная и глобальная геометрия Вселенной.
- Стационарная модель - Модель Вселенной - альтернатива модели Большого взрыва, дискредитированной теории, отрицавшей Большой взрыв и утверждавшей, что Вселенная существовала всегда.
Примечания
- ^ Дополнительная информация и ссылки на тесты общей теории относительности приведены в статье « Тесты общей теории относительности» .
- ↑ Нет единого мнения о том, как долго длилась фаза Большого взрыва. Для одних писателей это обозначает лишь начальную сингулярность, для других — всю историю Вселенной. Обычно говорят, что по крайней мере первые несколько минут (в течение которых синтезируется гелий) произошли «во время Большого взрыва».
- ^ Обычно сообщается, что Хойл хотел, чтобы это было уничижительно. Однако позже Хойл это опроверг, заявив, что это было просто поразительное изображение, призванное подчеркнуть для радиослушателей разницу между двумя теориями. [47]
- ^ Строго говоря, темная энергия в форме космологической константы приводит Вселенную к плоскому состоянию; однако наша Вселенная оставалась почти плоской в течение нескольких миллиардов лет, прежде чем плотность темной энергии стала значительной.
Ссылки
- ^ Jump up to: а б с д Бридж, Марк (директор) (30 июля 2014 г.). Первая секунда Большого взрыва . Как работает Вселенная . Силвер-Спринг, Мэриленд. Научный канал .
- ^ Шелк 2009 , с. 208.
- ^ Сингх 2004 , с. 560. Книга ограничена 532 страницами. Запрошена правильная исходная страница.
- ^ Научная группа НАСА/WMAP (6 июня 2011 г.). «Космология: Исследование Вселенной» . Вселенная 101: Теория большого взрыва . Вашингтон, округ Колумбия: НАСА . Архивировано из оригинала 29 июня 2011 года . Проверено 18 декабря 2019 г.
Во втором разделе обсуждаются классические проверки теории Большого взрыва, которые делают ее настолько убедительной, как наиболее вероятное и точное описание нашей Вселенной.
- ^ Jump up to: а б Чоу 2008 , с. 211
- ^ Партридж 1995 , с. XVII
- ^ Краг 1996 , с. 319 : «В то же время наблюдения явно склонили чашу весов в пользу релятивистской теории большого взрыва...»
- ^ «Планк открывает почти идеальную Вселенную» . Макс-Планк-Гезельшафт. 21 марта 2013 года . Проверено 17 ноября 2020 г.
- ^ Jump up to: а б с д и ж Пиблз, PJE ; Ратра, Бхарат (22 апреля 2003 г.). «Космологическая постоянная и темная энергия». Обзоры современной физики . 75 (2): 559–606. arXiv : astro-ph/0207347 . Бибкод : 2003РвМП...75..559П . дои : 10.1103/RevModPhys.75.559 . ISSN 0034-6861 . S2CID 118961123 .
- ^ Райт, Эдвард Л. (24 мая 2013 г.). «Часто задаваемые вопросы по космологии: каковы доказательства Большого взрыва?» . Учебник по космологии Неда Райта . Лос-Анджелес: Отдел астрономии и астрофизики Калифорнийского университета, Лос-Анджелес . Архивировано из оригинала 20 июня 2013 года . Проверено 25 ноября 2019 г.
- ^ Фрэнсис, Чарльз (2018). Свет после тьмы I: Структуры неба . Трубадор Паблишинг ООО с. 199. ИСБН 9781785897122 .
- ^ Иванчик Александр Владимирович; Потехин Александр Юрьевич; Варшалович, Дмитрий А. (март 1999 г.). «Константа тонкой структуры: новый наблюдательный предел ее космологического изменения и некоторые теоретические последствия». Астрономия и астрофизика . 343 (2): 439–445. arXiv : astro-ph/9810166 . Бибкод : 1999A&A...343..439I .
- ^ Турышев, Слава Г. (ноябрь 2008 г.). «Экспериментальные проверки общей теории относительности». Ежегодный обзор ядерной науки и науки о элементарных частицах . 58 (1): 207–248. arXiv : 0806.1731 . Бибкод : 2008ARNPS..58..207T . дои : 10.1146/annurev.nucl.58.020807.111839 . S2CID 119199160 .
- ^ Исхак, Мустафа (декабрь 2019 г.). «Проверка общей теории относительности в космологии» . Живые обзоры в теории относительности . 22 (1): 204. arXiv : 1806.10122 . Бибкод : 2019LRR....22....1I . дои : 10.1007/s41114-018-0017-4 . ПМК 6299071 . ПМИД 30613193 . 1.
- ^ Гудман, Джереми (15 августа 1995 г.). «Пересмотр геоцентризма» (PDF) . Физический обзор D . 52 (4): 1821–1827. arXiv : astro-ph/9506068 . Бибкод : 1995ФРвД..52.1821Г . дои : 10.1103/PhysRevD.52.1821 . ПМИД 10019408 . S2CID 37979862 . Архивировано (PDF) из оригинала 2 мая 2019 года . Проверено 2 декабря 2019 г.
- ^ Jump up to: а б с Колб и Тернер 1988 , гл. 3
- ^ Энквист, К.; Сиркка, Дж. (сентябрь 1993 г.). «Химическое равновесие в газе КХД в ранней Вселенной». Буквы по физике Б. 314 (3–4): 298–302. arXiv : hep-ph/9304273 . Бибкод : 1993PhLB..314..298E . дои : 10.1016/0370-2693(93)91239-J . S2CID 119406262 .
- ^ Jump up to: а б Хокинг и Эллис, 1973 г.
- ^ Уолл, Майк (21 октября 2011 г.). «Большой взрыв: что на самом деле произошло при рождении нашей Вселенной?» . История и будущее космоса . Space.com . Проверено 7 сентября 2023 г.
- ^ Роос 2012 , с. 216: «Эта сингулярность называется Большим взрывом».
- ^ Дрис 1990 , стр. 223–224
- ^ Jump up to: а б Сотрудничество Планка (октябрь 2016 г.). « Результаты Planck 2015. XIII. Космологические параметры». Астрономия и астрофизика . 594 : Статья А13. arXiv : 1502.01589 . Бибкод : 2016A&A...594A..13P . дои : 10.1051/0004-6361/201525830 . S2CID 119262962 . (См. Таблицу 4 «Возраст/год», последний столбец).
- ^ Массер, Джордж (22 сентября 2003 г.). «Почему вся эта материя сразу не схлопнулась в черную дыру?» . Научный американец . Проверено 22 марта 2020 г.
- ^ Jump up to: а б Унру, В.Г.; Семенов, Г.В., ред. (1988). Ранняя Вселенная . Рейдель. ISBN 90-277-2619-1 . OCLC 905464231 .
- ^ Хоули, Джон Ф.; Холкомб, Кэтрин А. (7 июля 2005 г.). Основы современной космологии . ОУП Оксфорд. п. 355. ИСБН 9780198530961 .
- ^ «Краткая история Вселенной» . www.astro.ucla.edu . Проверено 28 апреля 2020 г.
- ^ Jump up to: а б Гут 1998 г.
- ^ Jump up to: а б «Модели Большого Взрыва возвращаются к планковскому времени» . гиперфизика.phy-astr.gsu.edu . Проверено 28 апреля 2020 г.
- ^ Шве, Филипп Ф.; Штейн, Бен П. (20 апреля 2005 г.). «Океан кварков» . Обновление новостей физики . Том. 728, нет. 1. Архивировано из оригинала 23 апреля 2005 года . Проверено 30 ноября 2019 г.
- ^ Хёг, Эрик (2014). «Астросоциология: Интервью о бесконечной Вселенной». Азиатский физический журнал . arXiv : 1408.4795 . Бибкод : 2014arXiv1408.4795H .
- ^ Jump up to: а б Колб и Тернер 1988 , гл. 6
- ^ Колб и Тернер 1988 , гл. 7
- ^ Вининк, январь (26 февраля 2009 г.). «Бариогенез» (PDF) . Томислав Прокопец. Архивировано (PDF) из оригинала 9 октября 2022 года.
- ^ Jump up to: а б с Колб и Тернер 1988 , гл. 4
- ^ Jump up to: а б Павлин 1999 , гл. 9
- ^ Клавин, Уитни; Дженкинс, Энн; Виллар, Рэй (7 января 2014 г.). «Команда НАСА Хаббл и Спитцер собираются исследовать далекие галактики» . Лаборатория реактивного движения . Вашингтон, округ Колумбия: НАСА . Архивировано из оригинала 3 сентября 2019 года . Проверено 8 января 2014 г.
- ^ Jump up to: а б Спергель, Дэвид Н .; Верде, Лисия ; Пейрис, Хиранья В .; и др. (сентябрь 2003 г.). «Наблюдения первокурсника Уилкинсона с помощью микроволнового зонда анизотропии (WMAP) : определение космологических параметров». Серия дополнений к астрофизическому журналу . 148 (1): 175–194. arXiv : astro-ph/0302209 . Бибкод : 2003ApJS..148..175S . дои : 10.1086/377226 . S2CID 10794058 .
- ^ Jump up to: а б с д Ярошик, Норман ; Беннетт, Чарльз Л .; Данкли, Джо ; и др. (февраль 2011 г.). «Семилетние наблюдения микроволнового зонда анизотропии Уилкинсона (WMAP) : карты неба, систематические ошибки и основные результаты» (PDF) . Серия дополнений к астрофизическому журналу . 192 (2): Статья 14. arXiv : 1001.4744 . Бибкод : 2011ApJS..192...14J . дои : 10.1088/0067-0049/192/2/14 . hdl : 2152/43001 . S2CID 46171526 . Архивировано (PDF) из оригинала 14 сентября 2019 года . Проверено 2 декабря 2019 г. (См. Таблицу 8.)
- ^ До свидания, Деннис (15 апреля 2020 г.). «Почему Большой Взрыв произвел нечто, а не ничего. Как материя получила преимущество над антиматерией в ранней Вселенной? Может быть, только может быть, нейтрино» . Нью-Йорк Таймс . Архивировано из оригинала 15 апреля 2020 года . Проверено 16 апреля 2020 г.
- ^ Мэнли 2011 , гл. 7: «Лучший бесплатный обед» [ нужна страница ] .
- ^ « Умер астроном «большого взрыва»» . Наука/Техн. Новости Би-би-си . Лондон: Би-би-си . 22 августа 2001 г. Архивировано из оригинала 3 сентября 2019 г. . Проверено 2 декабря 2019 г.
- ^ «Хойл на радио: Создание «Большого взрыва» » . Фред Хойл: Интернет-выставка . Кембридж: Колледж Святого Иоанна . Архивировано из оригинала 26 мая 2014 года . Проверено 2 декабря 2019 г.
- ^ Jump up to: а б с д и ж г Краг, Хельге (апрель 2013 г.). «Большой взрыв: этимология имени» . Астрономия и геофизика . 54 (2): 2,28–2,30. Бибкод : 2013A&G....54b2.28K . doi : 10.1093/astrogeo/att035 .
- ^ Мэттсон, Барбара (руководитель проекта) (8 декабря 2017 г.). «Хойл высмеивает теорию Вселенной «Большого взрыва»» . Cosmic Times (организуется Imagine the Universe!) . Гринбелт, Мэриленд: НАСА : Архив научных исследований по астрофизике высоких энергий . ОСЛК 227004453 . Архивировано из оригинала 10 марта 2018 года . Проверено 2 декабря 2019 г.
- ^ Jump up to: а б Мэтью, Сантош (2013). Очерки границ современной астрофизики и космологии . Springer Science & Business Media. п. 13. ISBN 978-3-319-01887-4 .
- ^ Jump up to: а б Тимоти Феррис пишет:«Термин «большой взрыв» был придуман с насмешливым намерением Фредом Хойлом, и его долговечность свидетельствует о творческих способностях и остроумии сэра Фреда. Действительно, этот термин выдержал международное соревнование, в котором трое судей — телевизионный научный репортер Хью Даунс, астроном Карл Саган и я просмотрели 13 099 заявок из 41 страны и пришли к выводу, что ни одна из них не была достаточно подходящей, чтобы заменить ее. Победитель не был объявлен, и, нравится нам это или нет, мы застряли в «большом взрыве». [54]
- ^ Jump up to: а б Кросуэлл 1995 , с. 113, глава 9
- ^ Jump up to: а б Миттон 2011 , с. 129 : «Чтобы создать картину в сознании слушателя, Хойл сравнил взрывную теорию происхождения Вселенной с «большим взрывом».
- ^ Краг, Хельге (2014). Хозяева Вселенной: Беседы с космологами прошлого . Издательство Оксфордского университета. п. 210н30. ISBN 978-0-19-103442-8 .
- ^ Хойл заявил:«Я постоянно стремился по радио – где у меня не было никаких наглядных средств, ничего, кроме устной речи – к визуальным изображениям. И это, казалось, был одним из способов отличить стационарный режим от взрывного Большого взрыва. И вот это было язык, который я использовал». [43] [49]
- ^ Калер, Джеймс Б. (2013). Маленькая книга звезд . Springer Science & Business Media. п. 3. ISBN 978-0-387-21621-8 .
- ^ Эмам, Моатаз (2021). Ковариантная физика: от классической механики к общей теории относительности и не только . Издательство Оксфордского университета. стр. 208–246. ISBN 978-0-19-886489-9 .
Термин «Большой взрыв» является неудачным. Это подразумевает «взрыв», а взрывы — это события, происходящие в космосе. Это неверно; этот термин описывает первый момент расширения самого пространства . Некоторые даже интерпретировали бы это как самое начало Вселенной, возникшей из «ничего». Трудно представить, что именно это было, но взрывом это точно не был.
- ^ Московиц, Клара (2010). «Был ли Большой взрыв на самом деле взрывом?» . Живая наука .
- ^
- Феррис, Тимоти (1998). Весь Шебанг: Отчет о состоянии Вселенной . Саймон и Шустер. п. 323н10. ISBN 978-0-684-83861-8 .
- Гейтер, Карл С.; Кавасос-Гейтер, Альма Э. (2012). Словарь научных цитат Гейтера (2-е изд.). Springer Science & Business Media. ISBN 978-1-4614-1114-7 . (цитирую Ферриса)
- ^ Московиц, Клара (25 сентября 2012 г.). «Телескоп Хаббл открывает самый дальний вид во Вселенную за всю историю» . Space.com . Нью-Йорк: Future plc . Архивировано из оригинала 12 октября 2019 года . Проверено 3 декабря 2019 г.
- ^ Слайфер, Весто М. (1913). «Лучевая скорость туманности Андромеды». Бюллетень обсерватории Лоуэлл . 1 (8): 56–57. Бибкод : 1913LowOB...2...56S .
- ^ Слайфер, Весто М. (январь 1915 г.). «Спектрографические наблюдения туманностей». Популярная астрономия . 23 : 21–24. Бибкод : 1915PA.....23...21S .
- ^ Jump up to: а б Фридман, Александр (декабрь 1922 г.). «О кривизне пространства». Журнал физики (на немецком языке). 10 (1): 377–386. Бибкод : 1922ZPhy...10..377F . дои : 10.1007/BF01332580 . S2CID 125190902 .
- Переведено на: Фридманн, Александр (декабрь 1999 г.). «О кривизне пространства». Общая теория относительности и гравитация . 31 (12): 1991–2000. Бибкод : 1999GReGr..31.1991F . дои : 10.1023/A:1026751225741 . S2CID 122950995 .
- ^ Jump up to: а б Хаббл, Эдвин (15 марта 1929 г.). «Связь между расстоянием и лучевой скоростью среди внегалактических туманностей» . Труды Национальной академии наук . 15 (3): 168–173. Бибкод : 1929PNAS...15..168H . дои : 10.1073/pnas.15.3.168 . ПМК 522427 . ПМИД 16577160 . Архивировано из оригинала 1 октября 2006 года . Проверено 28 ноября 2019 г. .
- ^ Кристиансон 1995
- ^ Jump up to: а б Леметр, Жорж (апрель 1927 г.). «Однородная Вселенная постоянной массы и увеличивающегося радиуса, учитывающая радиальную скорость внегалактических туманностей» . Анналы Брюссельского научного общества (на французском языке). 47 :49–59. Бибкод : 1927АССБ...47...49Л .
- Переведено на: Леметр, Жорж (март 1931 г.). «Однородная Вселенная постоянной массы и возрастающего радиуса, объясняющая лучевую скорость внегалактических туманностей» . Ежемесячные уведомления Королевского астрономического общества . 91 (5): 483–490. Бибкод : 1931MNRAS..91..483L . дои : 10.1093/mnras/91.5.483 .
- ^ Леметр, аббат Жорж (24 октября 1931 г.). «Вклад в дискуссию Британской ассоциации по эволюции Вселенной». Природа . 128 (3234): 704–706. Бибкод : 1931Natur.128..704L . дои : 10.1038/128704a0 . S2CID 4028196 .
- ^ Краг 1996
- ^ «Представлена теория большого взрыва – 1927 год» . Научная одиссея . Бостон, Массачусетс: WGBH Boston . 1998. Архивировано из оригинала 23 апреля 1999 года . Проверено 31 июля 2014 г.
- ^ Эддингтон, Артур С. (21 марта 1931 г.). «Конец света: с точки зрения математической физики». Природа . 127 (3203): 447–453. Бибкод : 1931Natur.127..447E . дои : 10.1038/127447a0 . S2CID 4140648 .
- ^ Аполлони, Симон (17 июня 2011 г.). « Отвратительно», «совсем не отвратительно»: как соответствующие эпистемические взгляды Жоржа Леметра и сэра Артура Эддингтона повлияли на то, как каждый из них подходил к идее начала Вселенной» . Научный журнал ИБСУ . 5 (1): 19–44.
- ^ Леметр, Жорж (9 мая 1931 г.). «Начало мира с точки зрения квантовой теории» . Природа . 127 (3210): 706. Бибкод : 1931Natur.127..706L . дои : 10.1038/127706b0 . ISSN 0028-0836 . S2CID 4089233 .
- ^ Милн 1935 г.
- ^ Толман 1934 г.
- ^ Цвики, Фриц (15 октября 1929 г.). «О красном смещении спектральных линий в межзвездном пространстве» . Труды Национальной академии наук . 15 (10): 773–779. Бибкод : 1929PNAS...15..773Z . дои : 10.1073/pnas.15.10.773 . ПМК 522555 . ПМИД 16577237 .
- ^ Хойл, Фред (октябрь 1948 г.). «Новая модель расширяющейся Вселенной» . Ежемесячные уведомления Королевского астрономического общества . 108 (5): 372–382. Бибкод : 1948MNRAS.108..372H . дои : 10.1093/mnras/108.5.372 .
- ^ Альфер, Ральф А .; Бете, Ганс ; Гамов, Георгий (1 апреля 1948 г.). «Происхождение химических элементов» . Физический обзор . 73 (7): 803–804. Бибкод : 1948PhRv...73..803A . дои : 10.1103/PhysRev.73.803 . ПМИД 18877094 .
- ^ Альфер, Ральф А .; Герман, Роберт (13 ноября 1948 г.). «Эволюция Вселенной». Природа . 162 (4124): 774–775. Бибкод : 1948Natur.162..774A . дои : 10.1038/162774b0 . S2CID 4113488 .
- ^ Jump up to: а б Пензиас, Арно А .; Уилсон, RW (июль 1965 г.). «Измерение избыточной температуры антенны на частоте 4080 МГц/с» . Астрофизический журнал . 142 : 419–421. Бибкод : 1965ApJ...142..419P . дои : 10.1086/148307 . Архивировано из оригинала 14 октября 2019 года . Проверено 5 декабря 2019 г.
- ^ Хокинг, Стивен В .; Эллис, Джордж Ф.Р. (апрель 1968 г.). «Космическое излучение черного тела и существование особенностей в нашей Вселенной». Астрофизический журнал . 152 : 25. Бибкод : 1968ApJ...152...25H . дои : 10.1086/149520 .
- ^ Хокинг, Стивен В .; Пенроуз, Роджер (27 января 1970 г.). «Особенности гравитационного коллапса и космологии». Труды Королевского общества A: Математические, физические и технические науки . 314 (1519): 529–548. Бибкод : 1970RSPSA.314..529H . дои : 10.1098/rspa.1970.0021 . S2CID 120208756 .
- ^ Гут, Алан (15 января 1981 г.). «Инфляционная вселенная: возможное решение проблем горизонта и плоскостности» . Физический обзор D . 23 (2): 347–356. Бибкод : 1981PhRvD..23..347G . дои : 10.1103/PhysRevD.23.347 .
- ^ Хухра, Джон П. (2008). «Постоянная Хаббла» . Наука . 256 (5055): 321–5. дои : 10.1126/science.256.5055.321 . ПМИД 17743107 . S2CID 206574821 . Архивировано из оригинала 30 сентября 2019 года . Проверено 5 декабря 2019 г.
- ^ Ливиус 2000 , с. 160
- ^ Наваби, Али Акбар; Риази, Нематолла (март 2003 г.). «Решена ли проблема возраста?». Журнал астрофизики и астрономии . 24 (1–2): 3–10. Бибкод : 2003JApA...24....3N . дои : 10.1007/BF03012187 . S2CID 123471347 .
- ^ Jump up to: а б Боггесс, Нэнси В.; Мэзер, Джон К .; Вайс, Райнер ; и др. (1 октября 1992 г.). «Миссия COBE: ее дизайн и реализация через два года после запуска» . Астрофизический журнал . 397 : 420–429. Бибкод : 1992ApJ...397..420B . дои : 10.1086/171797 .
- ^ Jump up to: а б с Спергель, Дэвид Н .; Бин, Рэйчел ; Доре, Оливье ; и др. (июнь 2007 г.). «Трехлетние наблюдения микроволновой анизотропии Уилкинсона (WMAP) : последствия для космологии». Серия дополнений к астрофизическому журналу . 170 (2): 377–408. arXiv : astro-ph/0603449 . Бибкод : 2007ApJS..170..377S . дои : 10.1086/513700 . S2CID 1386346 .
- ^ Рейсс, Адам Г.; Филиппенко Алексей Владимирович; Чаллис, Питер; Клоккьятти, Алехандро; Диркс, Алан; Гарнавич, Питер М.; Гиллиланд, Рон Л.; Хоган, Крейг Дж.; Джа, Саураб; Киршнер, Роберт П.; Лейбундгут, Б.; Филлипс, ММ; Рейсс, Дэвид; Шмидт, Брайан П.; Шоммер, Роберт А.; Смит, Р. Крис; Спиромилио, Дж.; Стаббс, Кристофер; Сунцев, Николай Б.; Тонри, Джон (1998). «Наблюдательные данные сверхновых об ускоряющейся Вселенной и космологической постоянной». Астрономический журнал . 116 (3): 1009–1038. arXiv : astro-ph/9805201 . Бибкод : 1998AJ....116.1009R . дои : 10.1086/300499 . S2CID 15640044 .
- ^ Перлмуттер, С.; Олдеринг, Г.; Гольдхабер, Г.; Кноп, РА; Ньюджент, П.; Кастро, П.Г.; Деустуа, С.; Фаббро, С.; Губар, А.; Грум, Делавэр; Хук, И.М.; Ким, АГ; Ким, МОЙ; Ли, Джей Си; Нуньес, Нью-Джерси; Боль, Р.; Пеннипакер, Чехия; Куимби, Р.; Лидман, К.; Эллис, РС; Ирвин, М.; МакМахон, Р.Г.; Руис-Лапуэнте, П.; Уолтон, Н.; Шефер, Б.; Бойл, Би Джей; Филиппенко А.В.; Мэтисон, Т.; Фрухтер, А.С.; Панагия, Н.; Ньюберг, HJM; Коуч, WJ (1999). «Измерения омеги и лямбды по 42 сверхновым с высоким красным смещением». Астрофизический журнал . 517 (2): 565–586. arXiv : astro-ph/9812133 . Бибкод : 1999ApJ...517..565P . дои : 10.1086/307221 . S2CID 118910636 .
- ^ Краусс 2012 , с. 118
- ^ Глэддерс, Майкл Д.; Да, Гонконг; Маджумдар, Субхабрата; и др. (20 января 2007 г.). «Космологические ограничения из исследования скоплений красной последовательности». Астрофизический журнал . 655 (1): 128–134. arXiv : astro-ph/0603588 . Бибкод : 2007ApJ...655..128G . дои : 10.1086/509909 . S2CID 10855653 .
- ^ Шеллард, Пол; и др., ред. (2012). «Четыре столпа стандартной космологии» . Пропагандистская деятельность . Кембридж, Великобритания: Центр теоретической космологии ; Кембриджский университет . Архивировано из оригинала 2 ноября 2013 года . Проверено 6 декабря 2019 г.
- С устаревшего сайта: Шеллард, Пол; и др., ред. (2006). «Четыре столпа стандартной космологии» . Кембриджская теория относительности и космология . Кембридж, Великобритания: Кембриджский университет. Архивировано из оригинала 28 января 1998 года . Проверено 6 декабря 2019 г.
- ^ Садуле, Бернар ; и др. «Прямые поиски темной материи» (PDF) . Astro2010: Десятилетний обзор астрономии и астрофизики (информационный документ). Вашингтон, округ Колумбия: Пресса национальных академий от имени Национального исследовательского совета Национальной академии наук . OCLC 850950122 . Архивировано из оригинала 13 апреля 2009 года . Проверено 8 декабря 2019 г.
- ^ Jump up to: а б Диманд, Юрг; Земп, Марсель; Мур, Бен; Стадель, Иоахим; Каролло, К. Марселла (декабрь 2005 г.). «Каспы в холодных гало темной материи» . Ежемесячные уведомления Королевского астрономического общества . 364 (2): 665–673. arXiv : astro-ph/0504215 . Бибкод : 2005MNRAS.364..665D . дои : 10.1111/j.1365-2966.2005.09601.x . S2CID 117769706 .
- ^ Jump up to: а б Буллок, Джеймс С. (2010). «Заметки о проблеме пропавших спутников». Мартинес-Дельгадо, Дэвид; Медиавилла, Эвенсио (ред.). Локальная групповая космология . стр. 95–122. arXiv : 1009.4505 . дои : 10.1017/CBO9781139152303.004 . ISBN 9781139152303 . S2CID 119270708 .
- ^ Кан, Роберт Н.; и др. (2009). «Информационный документ: Комплексная космическая миссия по использованию темной энергии» (PDF) . Astro2010: Десятилетний обзор астрономии и астрофизики, Science White Papers, вып. 35 (информационный документ). 2010 . Вашингтон, округ Колумбия: National Academies Press от имени Национального исследовательского совета Национальной академии наук : 35. Бибкод : 2009astro2010S..35B . OCLC 850950122 . Архивировано из оригинала 7 августа 2011 года . Проверено 8 декабря 2019 г.
- ^ Jump up to: а б Банн, EF; Хогг, Д.В. (2009). «Кинематическое происхождение космологического красного смещения». Американский журнал физики . 77 (8): 688–694. arXiv : 0808.1081 . Бибкод : 2009AmJPh..77..688B . дои : 10.1119/1.3129103 . S2CID 1365918 .
- ^ Ди Валентино, Элеонора; Мена, Ольга; Пан, Суприя; Визинелли, Лука; Ян, Вэйцян; Мельчиорри, Алессандро; Мота, Дэвид Ф.; Рисс, Адам Г.; Силк, Джозеф (2021). «В сфере напряженности Хаббла — обзор решений». Классическая и квантовая гравитация . 38 (15): 153001. arXiv : 2103.01183 . Бибкод : 2021CQGra..38o3001D . дои : 10.1088/1361-6382/ac086d . S2CID 232092525 .
- ^ Уайт, Мартин (1999). «Анизотропия реликтового излучения» (PDF) . В Арисаке — Кацуши; Берн, Цви (ред.). DPF 99: Материалы встречи в Лос-Анджелесе . Конференция Отдела частиц и полей 1999 г. (DPF '99) . Лос-Анджелес: Калифорнийский университет в Лос-Анджелесе от имени Американского физического общества . arXiv : astro-ph/9903232 . Бибкод : 1999dpf..conf.....W . OCLC 43669022 . Доклад № 9–10: Космический микроволновый фон. Архивировано (PDF) из оригинала 4 февраля 2017 г. Проверено 9 декабря 2019 г.
- ^ Беннетт, Чарльз Л .; Ларсон, Дэвин; Вейланд, Джанет Л.; и др. (октябрь 2013 г.). «Девятилетние наблюдения микроволнового зонда анизотропии Уилкинсона (WMAP) : окончательные карты и результаты». Серия дополнений к астрофизическому журналу . 208 (2): Статья 20. arXiv : 1212.5225 . Бибкод : 2013ApJS..208...20B . дои : 10.1088/0067-0049/208/2/20 . S2CID 119271232 .
- ^ Гэннон, Меган (21 декабря 2012 г.). «Раскрыта новая «детская картинка» Вселенной» . Space.com . Нью-Йорк: Future plc . Архивировано из оригинала 29 октября 2019 года . Проверено 9 декабря 2019 г.
- ^ Райт 2004 , с. 291
- ^ Мельчиорри, Алессандро; Аде, Питер А.Р.; де Бернардис, Паоло; и др. (20 июня 2000 г.). «Измерение ома в ходе североамериканского испытательного полета Бумеранга». Письма астрофизического журнала . 536 (2): L63–L66. arXiv : astro-ph/9911445 . Бибкод : 2000ApJ...536L..63M . дои : 10.1086/312744 . ПМИД 10859119 . S2CID 27518923 .
- ^ де Бернардис, Паоло; Аде, Питер А.Р.; Бок, Джеймс Дж.; и др. (27 апреля 2000 г.). «Плоская Вселенная на основе карт космического микроволнового фонового излучения высокого разрешения» (PDF) . Природа . 404 (6781): 955–959. arXiv : astro-ph/0004404 . Бибкод : 2000Natur.404..955D . дои : 10.1038/35010035 . hdl : 10044/1/60851 . ПМИД 10801117 . S2CID 4412370 . Архивировано (PDF) из оригинала 2 мая 2019 года . Проверено 10 декабря 2019 г.
- ^ Миллер, Андре Д.; Колдуэлл, Роберт Х.; Девлин, Марк Джозеф; и др. (10 октября 1999 г.). «Измерение углового спектра мощности космического микроволнового фона от l = 100 до 400». Письма астрофизического журнала . 524 (1): Л1–Л4. arXiv : astro-ph/9906421 . Бибкод : 1999ApJ...524L...1M . дои : 10.1086/312293 . S2CID 1924091 .
- ^ Стейгман, Гэри (февраль 2006 г.). «Первичный нуклеосинтез: успехи и проблемы». Международный журнал современной физики Э. 15 (1): 1–36. arXiv : astro-ph/0511534 . Бибкод : 2006IJMPE..15....1S . CiteSeerX 10.1.1.337.542 . дои : 10.1142/S0218301306004028 . S2CID 12188807 .
- ^ Jump up to: а б с Райден 2003 г.
- ^ О'Каллаган, Джонатан (6 декабря 2022 г.). «Астрономы борются с открытием JWST ранних галактик» . Научный американец . Проверено 13 февраля 2023 г.
- ^ Jump up to: а б Бертшингер, Эдмунд (2000). «Космологическая теория возмущений и формирование структур». arXiv : astro-ph/0101009 .
- ^ Берчингер, Эдмунд (сентябрь 1998 г.). «Моделирование структурообразования во Вселенной» (PDF) . Ежегодный обзор астрономии и астрофизики . 36 (1): 599–654. Бибкод : 1998ARA&A..36..599B . дои : 10.1146/annurev.astro.36.1.599 . S2CID 29015610 . Архивировано из оригинала (PDF) 9 марта 2019 года.
- ^ «Результаты и данные BICEP2, март 2014 г.» . Эксперименты BICEP и Keck Array CMB . Кембридж, Массачусетс: Исследовательские вычисления FAS , Гарвардский университет . 16 декабря 2014 г. [первоначально результаты были опубликованы 17 марта 2014 г.]. Архивировано из оригинала 18 марта 2014 года . Проверено 10 декабря 2019 г.
- ^ Клавин, Уитни (17 марта 2014 г.). «Технологии НАСА рассматривают рождение Вселенной» . Лаборатория реактивного движения . Вашингтон, округ Колумбия: НАСА . Архивировано из оригинала 10 октября 2019 года . Проверено 10 декабря 2019 г.
- ^ До свидания, Деннис (17 марта 2014 г.). «Космическая рябь раскрывает дымящийся пистолет Большого взрыва» . Космос и космос. Нью-Йорк Таймс . Нью-Йорк. ISSN 0362-4331 . Архивировано из оригинала 17 марта 2014 года . Проверено 11 декабря 2019 г. «Версия этой статьи появится в печати 18 марта 2014 года, раздел А, страница 1 нью-йоркского издания, с заголовком: «Космическая рябь раскрывает дымящийся пистолет Большого взрыва». Интернет-версия этой статьи первоначально называлась «Обнаружение волн в космических контрфорсах, знаменующих теорию Большого взрыва».
- ^ До свидания, Деннис (24 марта 2014 г.). «Рябь от Большого Взрыва» . Там. Нью-Йорк Таймс . Нью-Йорк. ISSN 0362-4331 . Архивировано из оригинала 25 марта 2014 года . Проверено 24 марта 2014 г. «Версия этой статьи появится в печати 25 марта 2014 года, раздел D, страница 1 нью-йоркского издания, с заголовком: Рябь от Большого взрыва».
- ^ Фумагалли, Микеле; О'Мира, Джон М.; Прочаска, Дж. Ксавье (2 декабря 2011 г.). «Обнаружение чистого газа через два миллиарда лет после Большого взрыва». Наука . 334 (6060): 1245–1249. arXiv : 1111.2334 . Бибкод : 2011Sci...334.1245F . дои : 10.1126/science.1213581 . ПМИД 22075722 . S2CID 2434386 .
- ^ Стивенс, Тим (10 ноября 2011 г.). «Астрономы обнаружили облака первичного газа из ранней Вселенной» . Новости Университета Санта-Крус . Санта-Крус, Калифорния: Калифорнийский университет, Санта-Крус . Архивировано из оригинала 14 ноября 2011 года . Проверено 11 декабря 2019 г.
- ^ Перли, Дэниел (21 февраля 2005 г.). «Определение возраста Вселенной, т о » . Беркли, Калифорния: Факультет астрономии Калифорнийского университета в Беркли . Архивировано из оригинала 11 сентября 2006 года . Проверено 11 декабря 2019 г.
- ^ Ян, Р.Дж., и Чжан, С.Н. (2010). Проблема возраста в модели ΛCDM. Ежемесячные уведомления Королевского астрономического общества, 407 (3), стр. 1835–1841.
- ^ Ю, Х., и Ван, ФЮ (2014). Решение проблемы космического возраста во вселенной $$ R_\mathrm {h}= ct $$. Европейский физический журнал C, 74 (10), 3090.
- ^ Шриананд, Рагунатан ; Нотердем, Паскье; Леду, Седрик; и др. (май 2008 г.). «Первое обнаружение CO в затухающей системе Лайман-α с высоким красным смещением» . Астрономия и астрофизика . 482 (3): L39–L42. arXiv : 0804.0116 . Бибкод : 2008A&A...482L..39S . дои : 10.1051/0004-6361:200809727 .
- ^ Августидис, Анастасиос; Луцци, Джемма; Мартинс, Карлос ЯП; и др. (14 февраля 2012 г.). «Ограничения на зависимость температуры и красного смещения реликтового излучения от SZ и измерений расстояний». Журнал космологии и физики астрочастиц . 2012 (2): Статья 013. arXiv : 1112.1862 . Бибкод : 2012JCAP...02..013A . CiteSeerX 10.1.1.758.6956 . дои : 10.1088/1475-7516/2012/02/013 . S2CID 119261969 .
- ^ Белушевич 2008 , стр. 16.
- ^ Гош, Паллаб (11 февраля 2016 г.). «Гравитационные волны Эйнштейна, «видимые» из черных дыр» . Наука и окружающая среда. Новости Би-би-си . Лондон: Би-би-си . Архивировано из оригинала 11 февраля 2016 года . Проверено 13 апреля 2017 г.
- ^ Биллингс, Ли (12 февраля 2016 г.). «Будущее гравитационно-волновой астрономии» . Научный американец . Архивировано из оригинала 13 февраля 2016 года . Проверено 13 апреля 2017 г.
- ^ Эрман, Джон ; Мостерин, Хесус (март 1999 г.). «Критический взгляд на инфляционную космологию». Философия науки . 66 (1): 1–49. дои : 10.1086/392675 . JSTOR 188736 . S2CID 120393154 .
- ^ Хокинг и Израиль 2010 , стр. 581–638, глава. 12: «Сингулярности и асимметрия времени» Роджера Пенроуза .
- ^ Пенроуз 1989
- ^ Стейнхардт, Пол Дж. (апрель 2011 г.). «Дебаты об инфляции: является ли теория, лежащая в основе современной космологии, глубоко ошибочной?» (PDF) . Научный американец . Том. 304, нет. 4. С. 36–43. doi : 10.1038/scientificamerican0411-36 . Архивировано (PDF) из оригинала 1 ноября 2019 г. Проверено 23 декабря 2019 г.
- ^ Sakharov, Andrei D. (10 January 1967). "Нарушение СР -инвариантности, С -асимметрия и барионная асимметрия Вселенной" [Violation of CP -invariance, C -asymmetry and baryon asymmetry of the Universe] (PDF) . Pis'ma v ZhETF (in Russian). 5 (1): 32–35. Archived (PDF) from the original on 28 July 2018.
- Переведено на: Сахаров, Андрей Дмитриевич (10 января 1967 г.). «Нарушение CP-инвариантности, С-асимметрии и барионной асимметрии Вселенной» (PDF) . Письма ЖЭТФ . 5 (1): 24–27. Архивировано (PDF) из оригинала 9 ноября 2019 года . Проверено 13 декабря 2019 г.
- Перепечатано в: Kolb & Turner 1988 , стр. 371–373.
- Переведено на: Сахаров, Андрей Дмитриевич (10 января 1967 г.). «Нарушение CP-инвариантности, С-асимметрии и барионной асимметрии Вселенной» (PDF) . Письма ЖЭТФ . 5 (1): 24–27. Архивировано (PDF) из оригинала 9 ноября 2019 года . Проверено 13 декабря 2019 г.
- ^ Вайнберг, Невин Н.; Камионковски, Марк (май 2003 г.). «Ограничение темной энергии обилием слабых гравитационных линз» . Ежемесячные уведомления Королевского астрономического общества . 341 (1): 251–262. arXiv : astro-ph/0210134 . Бибкод : 2003MNRAS.341..251W . дои : 10.1046/j.1365-8711.2003.06421.x . S2CID 1193946 .
- ^ Уайт, Мартин. «Барионные акустические колебания и темная энергия» .
- ^ Алам, Шадаб; и др. (апрель 2021 г.). «Завершенное расширенное спектроскопическое исследование барионных колебаний SDSS-IV: космологические последствия двух десятилетий спектроскопических исследований в обсерватории Апач-Пойнт». Физический обзор D . 103 (8): 083533. arXiv : 2007.08991 . Бибкод : 2021ФРвД.103х3533А . дои : 10.1103/PhysRevD.103.083533 .
- ^ Танабаши, М. 2018 , стр. 406–413 , гл. 27: «Темная энергия» (пересмотрено в сентябре 2017 г.), Дэвид Х. Вайнберг и Мартин Уайт.
- Olive 2014 , стр. 361–368 , гл. 26: «Темная энергия» (ноябрь 2013 г.) Майкла Дж. Мортонсона, Дэвида Х. Вайнберга и Мартина Уайта. Бибкод : 2014arXiv1401.0046M
- ^ Руг, Свенд Э.; Цинкернагель, Хенрик (декабрь 2002 г.). «Квантовый вакуум и проблема космологической постоянной». Исследования по истории и философии науки . Часть B. 33 (4): 663–705. arXiv : hep-th/0012253 . Бибкод : 2002ШПМП..33..663Р . дои : 10.1016/S1355-2198(02)00033-3 . S2CID 9007190 .
- ^ Кил, Уильям К. (октябрь 2009 г.) [Последние изменения: февраль 2015 г.]. «Тёмная материя» . Конспекты лекций Билла Киля - Галактики и Вселенная . Архивировано из оригинала 3 мая 2019 года . Проверено 15 декабря 2019 г.
- ^ Танабаши, М. 2018 , стр. 396–405 , гл. 26: «Темная материя» (пересмотрено в сентябре 2017 г.) Мануэля Дреса и Жиля Жербье.
- Яо, В.-М. 2006 , стр. 233–237 , гл. 22: «Темная материя» (сентябрь 2003 г.) Мануэля Дреса и Жиля Жербье.
- ^ Додельсон, Скотт (31 декабря 2011 г.). «Настоящая проблема с MOND». Международный журнал современной физики Д. 20 (14): 2749–2753. arXiv : 1112.1320 . Бибкод : 2011IJMPD..20.2749D . дои : 10.1142/S0218271811020561 . S2CID 119194106 .
- ^ Jump up to: а б с Колб и Тернер 1988 , гл. 8
- ^ Пенроуз, 2007 г.
- ^ Jump up to: а б Филиппенко Алексей Владимирович ; Пасачофф, Джей М. (март – апрель 2002 г.). «Вселенная из ничего» . Меркурий . Том. 31, нет. 2. п. 15. Бибкод : 2002Mercu..31b..15F . Архивировано из оригинала 22 октября 2013 года . Проверено 10 марта 2010 г.
- ^ Jump up to: а б Лоуренс М. Краусс (спикер); Р. Элизабет Корнуэлл (продюсер) (21 октября 2009 г.). «Вселенная из ничего», Лоуренс Краусс, AAI, 2009 г. (видео). Вашингтон, округ Колумбия: Фонд разума и науки Ричарда Докинза . Архивировано из оригинала 23 ноября 2021 года . Проверено 17 октября 2011 г.
- ^ Хокинг и Израиль 2010 , стр. 504–517, глава. 9: «Космология большого взрыва — загадки и панацеи» Роберта Х. Дике и Филиппа Дж. Э. Пиблза .
- ^ «Краткие ответы на космические вопросы» . Форум Вселенной . Кембридж, Массачусетс: Гарвард-Смитсоновский центр астрофизики . Архивировано из оригинала 13 апреля 2016 года . Проверено 18 декабря 2019 г. Архивный сайт: «Роль Форума Вселенной как части Сети поддержки образования НАСА завершилась в сентябре 2009 года».
- ^ Дэвис, Тамара М .; Лайнуивер, Чарльз Х. (31 марта 2004 г.). «Расширяющаяся путаница: распространенные заблуждения о космологических горизонтах и сверхсветовом расширении Вселенной». Публикации Астрономического общества Австралии . 21 (1): 97–109. arXiv : astro-ph/0310808 . Бибкод : 2004PASA...21...97D . дои : 10.1071/as03040 . S2CID 13068122 .
- ^ Пикок, Дж. А. (2008). «Обличительная речь о расширении пространства». arXiv : 0809.4573 [ астроф-ф ].
- ^ Старобинский, Алексей (2000). «Будущее и происхождение нашей Вселенной: современный взгляд» . В Бурдюже В.; Хозин Г. (ред.). Будущее Вселенной и будущее нашей цивилизации . Материалы симпозиума, состоявшегося в Будапеште-Дебрецене, Венгрия, 2–6 июля 1999 г. Сингапур: World Scientific Publishing. п. 71. Бибкод : 2000fufc.conf...71S . дои : 10.1142/9789812793324_0008 . ISBN 9810242646 . S2CID 37813302 .
- ^ Хокинг 1988 , с. 69.
- ^ Кун, Роберт Лоуренс (23 декабря 2015 г.). «Противостояние Мультивселенной: что означало бы« бесконечные вселенные »» . Space.com . Проверено 7 января 2024 г.
- ^ Кэрролл и
- ^ Беккерс, Майк (16 февраля 2015 г.). « Квантовый трюк устраняет сингулярность Большого взрыва». Космология. Спектр науки (на немецком языке). Архивировано из оригинала 21 июля 2017 года . Проверено 19 декабря 2019 г. Гугл перевод
- Али, Ахмед Фараг ; Дас, Саурья (4 февраля 2015 г.). «Космология из квантового потенциала». Буквы по физике Б. 741 : 276–279. arXiv : 1404.3093v3 . Бибкод : 2015PhLB..741..276F . дои : 10.1016/j.physletb.2014.12.057 . S2CID 55463396 .
- Лашин, Эльсаед И. (7 марта 2016 г.). «О правильности космологии от квантового потенциала». Буквы по современной физике А. 31 (7): 1650044. arXiv : 1505.03070 . Бибкод : 2016МПЛА...3150044Л . дои : 10.1142/S0217732316500449 . S2CID 119220266 .
- Дас, Саурья; Раджат К., Бхадури (21 мая 2015 г.). «Темная материя и темная энергия из конденсата Бозе – Эйнштейна». Классическая и квантовая гравитация . 32 (10): 105003. arXiv : 1411.0753 . Бибкод : 2015CQGra..32j5003D . дои : 10.1088/0264-9381/32/10/105003 . S2CID 119247745 .
- Али, Ахмед Фараг ; Дас, Саурья (4 февраля 2015 г.). «Космология из квантового потенциала». Буквы по физике Б. 741 : 276–279. arXiv : 1404.3093v3 . Бибкод : 2015PhLB..741..276F . дои : 10.1016/j.physletb.2014.12.057 . S2CID 55463396 .
- ^ Хокинг, Стивен В. (1996). «Начало времен» . Стивен Хокинг (лекция). Лондон: Фонд Стивена Хокинга. Архивировано из оригинала 6 ноября 2019 года . Проверено 26 апреля 2017 г. .
- ^ Уолл, Майк (24 июня 2012 г.). «Большому взрыву не нужен был Бог, чтобы создать Вселенную, говорят исследователи» . Space.com .
- ^ Прощай, Деннис (22 мая 2001 г.). «До Большого взрыва было… что?» . Нью-Йорк Таймс . Архивировано из оригинала 27 февраля 2013 года.
- ^ Он, Дуншань; Гао, Дунфэн; Цай, Цин-юй (3 апреля 2014 г.). «Спонтанное создание Вселенной из ничего». Физический обзор D . 89 (8): 083510. arXiv : 1404.1207 . Бибкод : 2014PhRvD..89h3510H . дои : 10.1103/PhysRevD.89.083510 . S2CID 118371273 .
- ^ Линкольн, Майя; Вассер, Ави (1 декабря 2013 г.). «Спонтанное создание Вселенной Ex Nihilo» . Физика Темной Вселенной . 2 (4): 195–199. Бибкод : 2013PDU.....2..195L . дои : 10.1016/j.dark.2013.11.004 . ISSN 2212-6864 .
- ^ Хартл, Джеймс Х .; Хокинг, Стивен В. (15 декабря 1983 г.). «Волновая функция Вселенной». Физический обзор D . 28 (12): 2960–2975. Бибкод : 1983PhRvD..28.2960H . дои : 10.1103/PhysRevD.28.2960 . S2CID 121947045 .
- ^ Хокинг 1988 , с. 71.
- ^ Ланглуа, Дэвид (2003). «Брановая космология». Приложение «Прогресс теоретической физики» . 148 : 181–212. arXiv : hep-th/0209261 . Бибкод : 2002ПТПС.148..181Л . дои : 10.1143/PTPS.148.181 . S2CID 9751130 .
- ^ Гиббонс, Шеллард и Ранкин 2003 , стр. 801–838, гл. 43: «Инфляционная теория против экпиротического/циклического сценария», Андрей Линде . Бибкод : 2003ftpc.book..801L
- ^ Тан, Кер (8 мая 2006 г.). «Переработанная Вселенная: теория может раскрыть космическую тайну» . Space.com . Нью-Йорк: Future plc . Архивировано из оригинала 6 сентября 2019 года . Проверено 19 декабря 2019 г.
- ^ Кеннеди, Барбара К. (1 июля 2007 г.). «Что произошло до Большого взрыва?» . Новости и события . Юниверсити-Парк, Пенсильвания: Научный колледж Эберли , Университет штата Пенсильвания . Архивировано из оригинала 15 декабря 2019 года . Проверено 19 декабря 2019 г.
- Бойовальд, Мартин (август 2007 г.). «Что произошло до Большого взрыва?» . Физика природы . 3 (8): 523–525. Бибкод : 2007NatPh...3..523B . дои : 10.1038/nphys654 .
- ^ Линде, Андрей Д. (май 1986 г.). «Вечная хаотическая инфляция» . Буквы по современной физике А. 1 (2): 81–85. Бибкод : 1986МПЛА....1...81Л . дои : 10.1142/S0217732386000129 . S2CID 123472763 . Архивировано из оригинала 17 апреля 2019 года.
- ^ Линде, Андрей Д. (14 августа 1986 г.). «Вечно существующая самовоспроизводящаяся хаотическая инфляционная вселенная». Буквы по физике Б. 175 (4): 395–400. Бибкод : 1986PhLB..175..395L . дои : 10.1016/0370-2693(86)90611-8 .
- ^ Научная группа НАСА/WMAP (29 июня 2015 г.). «Какова окончательная судьба Вселенной?» . Вселенная 101: Теория большого взрыва . Вашингтон, округ Колумбия: НАСА . Архивировано из оригинала 15 октября 2019 года . Проверено 18 декабря 2019 г.
- ^ Адамс, Фред С .; Лафлин, Грегори (апрель 1997 г.). «Умирающая вселенная: долгосрочная судьба и эволюция астрофизических объектов». Обзоры современной физики . 69 (2): 337–372. arXiv : astro-ph/9701131 . Бибкод : 1997РвМП...69..337А . дои : 10.1103/RevModPhys.69.337 . S2CID 12173790 . .
- ^ Колдуэлл, Роберт Р .; Камионковски, Марк ; Вайнберг, Невин Н. (15 августа 2003 г.). «Фантомная энергия: темная энергия с w <-1 вызывает космический Судный день». Письма о физических отзывах . 91 (7): 071301. arXiv : astro-ph/0302506 . Бибкод : 2003PhRvL..91g1301C . doi : 10.1103/PhysRevLett.91.071301 . ПМИД 12935004 . S2CID 119498512 .
- ^ Харрис 2002 , с. 128
- ^ Jump up to: а б Кадр 2009 , стр. 137–141.
- ^ Харрисон 2010 , с. 9
- ^ Харрис 2002 , с. 129
- ^ Крейг, Уильям Лейн (декабрь 1999 г.). «Основной вопрос происхождения: Бог и начало Вселенной». Астрофизика и космическая наука (лекция). 269–270 (1–4): 721–738. Бибкод : 1999Ap&SS.269..721C . дои : 10.1023/A:1017083700096 . S2CID 117794135 .
- Блок и др. 2000 , стр. 723–740 дои : 10.1007/978-94-011-4114-7_85
- Крейг, Уильям Лейн . «Основной вопрос происхождения: Бог и начало Вселенной» . Научные труды: Существование Бога. Разумная вера . Даллас, Техас. Архивировано из оригинала 29 декабря 2019 года . Проверено 21 декабря 2019 г.
- ^ Хокинг 1988 , Введение: «...вселенная без края в пространстве, без начала и конца во времени, и Создателю нечего делать». — Карл Саган .
Библиография
- Белушевич, Радое (2008). Теория относительности, астрофизика и космология . Том 1. Вайнхайм: Wiley-VCH . ISBN 978-3-527-40764-4 . OCLC 876678499 .
- Блок, Дэвид Л.; Пуэрари, Иванио; Стоктон, Алан; и др., ред. (2000). На пути к новому тысячелетию в морфологии галактик: материалы международной конференции «На пути к новому тысячелетию в морфологии галактик: от z = 0 до Лайманского разлома», состоявшейся в конференц-центре Эском, Мидранд, Южная Африка, 13–18 сентября 1999 г. Дордрехт: Kluwer Academic Publishers . дои : 10.1007/978-94-011-4114-7 . ISBN 978-94-010-5801-8 . LCCN 00042415 . OCLC 851369444 . «Перепечатано из томов 269–270 по астрофизике и космической науке , № 1–4, 1999 г.».
- Блок, Дэвид Л. (2012). «Жорж Леметр и закон эпонимии Стиглера». В Холдере, Родни Д.; Миттон, Саймон (ред.). Затмение Хаббла: Леметр и цензура . Библиотека астрофизики и космических наук. Том. 395. Гейдельберг; Нью-Йорк: Спрингер . стр. 89–96. arXiv : 1106.3928v2 . Бибкод : 2012ASSL..395...89B . дои : 10.1007/978-3-642-32254-9_8 . ISBN 978-3-642-32253-2 . LCCN 2012956159 . OCLC 839779611 . S2CID 119205665 .
- Кэрролл, Шон М. (nd). «Почему есть что-то, а не ничего?». В Ноксе, Элеонора; Уилсон, Аластер (ред.). Рутледж-компаньон по философии физики . Лондон: Рутледж . arXiv : 1802.02231v2 . Бибкод : 2018arXiv180202231C .
- Чоу, Тай Л. (2008). Гравитация, черные дыры и очень ранняя Вселенная: введение в общую теорию относительности и космологию . Нью-Йорк: Спрингер . ISBN 978-0-387-73629-7 . LCCN 2007936678 . OCLC 798281050 .
- Кристиансон, Гейл Э. (1995). Эдвин Хаббл: Моряк туманностей . Нью-Йорк: Фаррар, Штраус и Жиру . ISBN 978-0-374-14660-3 . LCCN 94045995 . OCLC 461940674 .
- Кросвелл, Кен (1995). Алхимия небес: в поисках смысла Млечного Пути . Иллюстрации Филиппа Вана (1-е изд. Anchor Books). Нью-Йорк: Anchor Books . ISBN 978-0-385-47213-5 . LCCN 94030452 . OCLC 1100389944 .
- д'Инверно, Рэй (1992). Знакомство с теорией относительности Эйнштейна . Оксфорд, Великобритания; Нью-Йорк: Кларендон Пресс ; Издательство Оксфордского университета . ISBN 978-0-19-859686-8 . LCCN 91024894 . OCLC 554124256 .
- Дрис, Уильям Б. (1990). За пределами Большого взрыва: квантовая космология и Бог . Ла Саль, Иллинойс: Издательская компания Open Court . ISBN 978-0-8126-9118-4 . LCCN 90038498 . OCLC 1088758264 .
- Фаррелл, Джон (2005). День без вчерашнего дня: Лемэтр, Эйнштейн и рождение современной космологии . Нью-Йорк: Thunder's Mouth Press . ISBN 978-1-56025-660-1 . LCCN 2006272995 . OCLC 61672162 .
- Фрейм, Том (2009). Потеря моей религии: неверие в Австралии . Сидней: UNSW Press. ISBN 978-1-921410-19-2 . OCLC 782015652 .
- Гиббонс, Гэри В .; Шеллард, EPS; Рэнкин, Стюарт Джон, ред. (2003). Будущее теоретической физики и космологии: празднование 60-летия Стивена Хокинга . Кембридж, Великобритания; Нью-Йорк: Издательство Кембриджского университета . ISBN 978-0-521-82081-3 . LCCN 2002041704 . OCLC 1088190774 .
- Гут, Алан Х. (1998) [первоначально опубликовано в 1997 году]. Инфляционная Вселенная: поиск новой теории космического происхождения . Предисловие Алана Лайтмана . Лондон: Винтажные книги . ISBN 978-0-09-995950-2 . LCCN 96046117 . OCLC 919672203 .
- Харрис, Джеймс Ф. (2002). Аналитическая философия религии . Справочник по современной философии религии. Том. 3. Дордрехт: Kluwer Academic Publishers . ISBN 978-1-4020-0530-5 . LCCN 2002071095 . OCLC 237734029 .
- Харрисон, Питер , изд. (2010). Кембриджский справочник по науке и религии . Кембриджские спутники религии. Кембридж, Великобритания; Нью-Йорк: Издательство Кембриджского университета . ISBN 978-0-521-71251-4 . LCCN 2010016793 . OCLC 972341489 .
- Хокинг, Стивен В .; Эллис, Джордж Ф.Р. (1973). Крупномасштабная структура пространства-времени . Кембридж, Великобритания: Издательство Кембриджского университета . ISBN 978-0-521-20016-5 . LCCN 72093671 . OCLC 1120809270 .
- Хокинг, Стивен В. (1988). Краткая история времени: от Большого взрыва до черных дыр . Введение Карла Сагана ; иллюстрации Рона Миллера. Нью-Йорк: Издательская группа Bantam Dell . ISBN 978-0-553-10953-5 . LCCN 87033333 . OCLC 39256652 .
- Хокинг, Стивен В .; Израиль, Вернер , ред. (2010) [Первоначально опубликовано в 1979 году]. Общая теория относительности: обзор столетия Эйнштейна . Кембридж, Великобритания: Издательство Кембриджского университета . ISBN 978-0-521-13798-0 . LCCN 78062112 . OCLC 759923541 .
- Колб, Эдвард ; Тернер, Майкл , ред. (1988). Ранняя Вселенная . Границы в физике. Том. 70. Редвуд-Сити, Калифорния: Аддисон-Уэсли . ISBN 978-0-201-11604-5 . LCCN 87037440 . OCLC 488800074 .
- Краг, Хельге (1996). Космология и полемика: историческое развитие двух теорий Вселенной . Принстон, Нью-Джерси: Издательство Принстонского университета . ISBN 978-0-691-02623-7 . LCCN 96005612 . OCLC 906709898 .
- Краусс, Лоуренс М. (2012). Вселенная из ничего: почему существует нечто, а не ничто . Послесловие Ричарда Докинза (1-е издание Free Press в твердом переплете). Нью-Йорк: Свободная пресса . ISBN 978-1-4516-2445-8 . LCCN 2011032519 . OCLC 709673181 .
- Ливио, Марио (2000). Ускоряющаяся Вселенная: бесконечное расширение, космологическая константа и красота космоса (исполнение аудиокниги Тома Паркса, Brilliance Audio ). Предисловие Аллана Сэндиджа . Нью-Йорк: Джон Уайли и сыновья . ISBN 978-0-471-32969-5 . LCCN 99022278 . OCLC 226086793 .
- Мэнли, Стивен Л. (2011). Брэндон, Джоди (ред.). Видения Мультивселенной . Помптон-Плейнс, Нью-Джерси : Книги с новыми страницами . ISBN 978-1-60163-720-8 . LCCN 2010052741 . ОСЛК 609531953 .
- Мартинес-Дельгадо, Дэвид, изд. (2013). Локальная групповая космология . Кембридж, Великобритания: Издательство Кембриджского университета . ISBN 978-1-107-02380-2 . LCCN 2013012345 . ОСЛК 875920635 . «Лекции, прочитанные на XX Зимней школе астрофизики Канарских островов, проходившей на Тенерифе, Испания, 17–18 ноября 2008 г.».
- Милн, Эдвард Артур (1935). Относительность, гравитация и структура мира . Международная серия монографий по физике. Оксфорд, Великобритания; Лондон: Кларендон Пресс ; Издательство Оксфордского университета . LCCN 35019093 . OCLC 1319934 .
- Миттон, Саймон (2011). Фред Хойл: Жизнь в науке . Кембридж, Великобритания; Нью-Йорк: Издательство Кембриджского университета . ISBN 978-0-521-18947-7 . LCCN 2011293530 . OCLC 774201415 .
- Олив, Калифорния; и др. ( Группа данных о частицах ) (2014). «Обзор физики элементарных частиц» (PDF) . Китайская физика C . 38 (9): 1–708. arXiv : 1412.1408 . Бибкод : 2014ChPhC..38i0001O . дои : 10.1088/1674-1137/38/9/090001 . ПМИД 10020536 . S2CID 118395784 . Архивировано (PDF) из оригинала 30 января 2017 года . Проверено 13 декабря 2019 г.
- Партридж, Р. Брюс (1995). 3K: Космическое микроволновое фоновое излучение . Кембриджская серия по астрофизике. Том. 25 (Иллюстрированное изд.). Кембридж, Великобритания: Издательство Кембриджского университета . ISBN 978-0-521-35808-8 . LCCN 94014980 . OCLC 1123849709 .
- Пикок, Джон А. (1999). Космологическая физика . Кембриджская серия по астрофизике. Кембридж, Великобритания; Нью-Йорк: Издательство Кембриджского университета . ISBN 978-0-521-42270-3 . LCCN 98029460 . OCLC 60157380 .
- Пенроуз, Роджер (1989). «Трудности с инфляционной космологией». В Фенивесе, Эрвин Дж. (ред.). Четырнадцатый Техасский симпозиум по релятивистской астрофизике . Анналы Нью-Йоркской академии наук . Том. 571. Нью-Йорк: Нью-Йоркская академия наук . стр. 249–264. Бибкод : 1989NYASA.571..249P . дои : 10.1111/j.1749-6632.1989.tb50513.x . ISBN 978-0-89766-526-1 . ISSN 0077-8923 . LCCN 89014030 . OCLC 318253659 . S2CID 122383812 . «Симпозиум проходил в Далласе, штат Техас, 11-16 декабря 1988 года».
- Пенроуз, Роджер (2007) [Первоначально опубликовано: Лондон: Джонатан Кейп , 2004]. Дорога к реальности (1-е изд. Винтажных книг). Нью-Йорк: Винтажные книги . ISBN 978-0-679-77631-4 . LCCN 2008274126 . OCLC 920157277 . Издание книги 2004 года доступно в Интернет-архиве . Проверено 20 декабря 2019 г.
- Роос, Мэттс (2012) [первоначально глава опубликована в 2008 году]. «Расширение Вселенной – Стандартная модель Большого взрыва» . В Энгволде, Оддбьёрн ; Стабель, Рольф; Черни, Божена; Латтанцио, Джон (ред.). Астрономия и астрофизика . Энциклопедия систем жизнеобеспечения . Том. II. Рэмси, остров Мэн: ЮНЕСКО в партнерстве с Eolss Publishers Co. Ltd. arXiv : 0802.2005 . Бибкод : 2008arXiv0802.2005R . ISBN 978-1-84826-823-4 . OCLC 691095693 .
- Райден, Барбара Сью (2003). Введение в космологию . Сан-Франциско: Аддисон-Уэсли . ISBN 978-0-8053-8912-8 . LCCN 2002013176 . OCLC 1087978842 .
- Силк, Джозеф (2009). Горизонты космологии: исследование видимых и невидимых миров . Серия Темплтона «Наука и религия». Коншохокен, Пенсильвания: Templeton Press . ISBN 978-1-59947-341-3 . LCCN 2009010014 . OCLC 818734366 .
- Сингх, Саймон (2004). Большой взрыв: происхождение Вселенной (1-е изд. в США). Нью-Йорк: Четвертая власть . Бибкод : 2004biba.book.....S . ISBN 978-0-00-716220-8 . LCCN 2004056306 . OCLC 475508230 .
- Танабаши, М.; и др. ( Группа данных о частицах ) (2018). «Обзор физики элементарных частиц» . Физический обзор D . 98 (3): 1–708. Бибкод : 2018PhRvD..98c0001T . doi : 10.1103/PhysRevD.98.030001 . hdl : 10044/1/68623 . ПМИД 10020536 .
- Толман, Ричард К. (1934). Теория относительности, термодинамика и космология . Международная серия монографий по физике. Оксфорд, Великобритания; Лондон: Кларендон Пресс ; Издательство Оксфордского университета . ISBN 978-0-486-65383-9 . ЛЦН 34032023 . OCLC 919976 .
- Вульфсон, Майкл (2013). Время, пространство, звезды и человек: история большого взрыва (2-е изд.). Лондон: Издательство Имперского колледжа . ISBN 978-1-84816-933-3 . LCCN 2013371163 . OCLC 835115510 .
- Райт, Эдвард Л. (2004). «Теоретический обзор анизотропии космического микроволнового фона». В Фридмане, Венди Л. (ред.). Измерение и моделирование Вселенной . Серия по астрофизике Обсерваторий Карнеги. Том. 2. Кембридж, Великобритания: Издательство Кембриджского университета . п. 291. arXiv : astro-ph/0305591 . Бибкод : 2004mmu..symp..291W . ISBN 978-0-521-75576-4 . LCCN 2005277053 . OCLC 937330165 .
- Яо, В.-М.; и др. ( Группа данных о частицах ) (2006). «Обзор физики элементарных частиц» (PDF) . Журнал физики G: Ядерная физика и физика элементарных частиц . 33 (1): 1–1232. Бибкод : 2006JPhG...33....1Y . дои : 10.1088/0954-3899/33/1/001 . S2CID 117958297 . Архивировано (PDF) из оригинала 12 февраля 2017 года . Проверено 16 декабря 2019 г.
Дальнейшее чтение
- Альфер, Ральф А .; Герман, Роберт (август 1988 г.). «Размышления о ранних работах по космологии «Большого взрыва»». Физика сегодня . 41 (8): 24–34. Бибкод : 1988PhT....41h..24A . дои : 10.1063/1.881126 .
- Барроу, Джон Д. (1994). Происхождение Вселенной . Магистр наук. Лондон: Вайденфельд и Николсон . ISBN 978-0-297-81497-9 . LCCN 94006343 . OCLC 490957073 .
- Дэвис, Пол (1992). Разум Бога: научная основа рационального мира . Нью-Йорк: Саймон и Шустер . ISBN 978-0-671-71069-9 . LCCN 91028606 . OCLC 59940452 .
- Лайнвивер, Чарльз Х.; Дэвис, Тамара М. (март 2005 г.). «Заблуждения о Большом взрыве» (PDF) . Научный американец . Том. 292, нет. 3. С. 36–45. Архивировано (PDF) из оригинала 9 октября 2019 года . Проверено 23 декабря 2019 г.
- Мэзер, Джон К .; Бослоу, Джон (1996). Самый первый свет: правдивая внутренняя история научного путешествия обратно к заре Вселенной (1-е изд.). Нью-Йорк: Основные книги . ISBN 978-0-465-01575-7 . LCCN 96010781 . OCLC 34357391 .
- Риордан, Майкл ; Зайц, Уильям А. (май 2006 г.). «Первые несколько микросекунд» (PDF) . Научный американец . Том. 294, нет. 5. С. 34–41. Бибкод : 2006SciAm.294e..34R . doi : 10.1038/scientificamerican0506-34a . Архивировано (PDF) из оригинала 30 ноября 2014 г.
- Сингх, Саймон (2005) [первое издание в США опубликовано в 2004 г.]. Большой взрыв: происхождение Вселенной (Harper Perennial; иллюстрированное издание). Нью-Йорк, Нью-Йорк: Многолетник Харпер . ISBN 978-0007162215 .
- Вайнберг, Стивен (1993) [первоначально опубликовано в 1977 году]. Первые три минуты: современный взгляд на происхождение Вселенной (обновленное издание). Нью-Йорк: Основные книги . ISBN 978-0-465-02437-7 . LCCN 93232406 . OCLC 488469247 . Первое издание доступно в Интернет-архиве . Проверено 23 декабря 2019 г.
Внешние ссылки
- Однажды во Вселенной. Архивировано 22 июня 2020 года в Wayback Machine - проект, финансируемый STFC, объясняющий историю Вселенной доступным для понимания языком.
- «Космология большого взрыва» - НАСА / WMAP научная группа
- «Большой взрыв» - Наука НАСА
- «Большой взрыв, большое замешательство» - модель большого взрыва с анимированной графикой Йоханнеса Кельмана.
- Космология в Керли
- «Проблема с «большим взрывом»» – ряд недавних статей иллюстрирует давнюю путаницу по поводу этого знаменитого термина. Сабина Хоссенфельде