Дрозофила меланогастер
Дрозофила меланогастер | |
---|---|
![]() | |
Плодовая мушка, питающаяся бананом | |
Научная классификация ![]() | |
Домен: | Эукариоты |
Королевство: | животное |
Тип: | Членистоногие |
Сорт: | Насекомое |
Заказ: | двукрылые |
Семья: | Дрозофилиды |
Род: | Дрозофила |
Подрод: | Софофора |
Species group: | melanogaster |
Species subgroup: | melanogaster |
Species complex: | melanogaster |
Species: | D. melanogaster |
Binomial name | |
Drosophila melanogaster | |
Synonyms[2] | |
List |

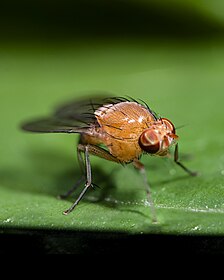
Drosophila melanogaster — вид мух ( насекомое отряда двукрылых ) семейства Drosophilidae . Этот вид часто называют плодовой мухой или малой плодовой мухой , реже — « уксусной мухой», « выжимочной мухой». [а] [5] или « банановая муха». [6] В дикой природе D. melanogaster привлекают гниющие фрукты и бродящие напитки, их часто можно встретить в садах, на кухнях и в пабах.
Начиная с предложения Чарльза В. Вудворта в 1901 году об использовании этого вида в качестве модельного организма , [7] [8] D. melanogaster продолжает широко использоваться для биологических исследований в области генетики , физиологии , микробного патогенеза и эволюции жизненного цикла . По состоянию на 2017 год шесть Нобелевских премий были присуждены дрозофилистам за работу по использованию этого насекомого. [9] [10]
Drosophila melanogaster обычно используется в исследованиях из-за ее быстрого жизненного цикла, относительно простой генетики (всего четыре пары хромосом ) и большого количества потомков на поколение. [11] Первоначально это был африканский вид, причем все неафриканские линии имели общее происхождение. [12] Его географический ареал включает все континенты, включая острова. [13] D. melanogaster — распространенный вредитель в домах, ресторанах и других местах, где подают еду. [14]
Flies belonging to the family Tephritidae are also called "fruit flies". This can cause confusion, especially in the Mediterranean, Australia, and South Africa, where the Mediterranean fruit fly Ceratitis capitata is an economic pest.
Etymology[edit]
The term "Drosophila", meaning "dew-loving", is a modern scientific Latin adaptation from Greek words δρόσος, drósos, "dew", and φιλία, philía, "lover". The term "melanogaster" meaning "black-belly", comes from Ancient Greek μέλας, mélas, “black”, and γᾰστήρ, gastḗr, "belly".
Physical appearance[edit]

Wild type fruit flies are yellow-brown, with brick-red eyes and transverse black rings across the abdomen. The black portions of the abdomen are the inspiration for the species name (melanogaster = "black-bellied"). The brick-red color of the eyes of the wild type fly are due to two pigments:[15] xanthommatin, which is brown and is derived from tryptophan, and drosopterins, which are red and are derived from guanosine triphosphate.[15] They exhibit sexual dimorphism; females are about 2.5 mm (0.10 in) long; males are slightly smaller with darker backs. Males are easily distinguished from females based on colour differences, with a distinct black patch at the abdomen, less noticeable in recently emerged flies, and the sex combs (a row of dark bristles on the tarsus of the first leg). Furthermore, males have a cluster of spiky hairs (claspers) surrounding the reproducing parts used to attach to the female during mating. Extensive images are found at FlyBase.[16]
Drosophila melanogaster can be distinguished from related species by the following combination of features: gena ~1/10 diameter of eye at greatest vertical height; wing hyaline and with costal index 2.4; male protarsus with a single row of ~12 setae forming a sex comb; male epandrial posterior lobe small and nearly triangular; female abdominal tergite 6 with dark band running to its ventral margin; female oviscapt small, pale, without dorsodistal depression and with 12-13 peg-like outer ovisensilla.[17][18]
Drosophila melanogaster flies can sense air currents with the hairs on their backs. Their eyes are sensitive to slight differences in light intensity and will instinctively fly away when a shadow or other movement is detected.[19]
Lifecycle and reproduction[edit]

Under optimal growth conditions at 25 °C (77 °F), the D. melanogaster lifespan is about 50 days from egg to death.[20] The developmental period for D. melanogaster varies with temperature, as with many ectothermic species. The shortest development time (egg to adult), 7 days, is achieved at 28 °C (82 °F).[21][22] Development times increase at higher temperatures (11 days at 30 °C or 86 °F) due to heat stress. Under ideal conditions, the development time at 25 °C (77 °F) is 8.5 days,[21][22][23] at 18 °C (64 °F) it takes 19 days[21][22] and at 12 °C (54 °F) it takes over 50 days.[21][22] Under crowded conditions, development time increases,[24] while the emerging flies are smaller.[24][25] Females lay some 400 eggs (embryos), about five at a time, into rotting fruit or other suitable material such as decaying mushrooms and sap fluxes. Drosophila melanogaster is a holometabolous insect, so it undergoes a full metamorphosis. Their life cycle is broken down into 4 stages: embryo, larva, pupa, adult.[26] The eggs, which are about 0.5 mm long, hatch after 12–15 hours (at 25 °C or 77 °F).[21][22] The resulting larvae grow for about 4 days (at 25 °C) while molting twice (into second- and third-instar larvae), at about 24 and 48 h after hatching.[21][22] During this time, they feed on the microorganisms that decompose the fruit, as well as on the sugar of the fruit itself. The mother puts feces on the egg sacs to establish the same microbial composition in the larvae's guts that has worked positively for herself.[27] Then the larvae encapsulate in the puparium and undergo a 4-day-long metamorphosis (at 25 °C), after which the adults eclose (emerge).[21][22]
Drosophila melanogaster, commonly known as the fruit fly, has been a significant model organism in embryonic development research. Many of its genes that regulate embryonic development and their mechanisms of action have been crucial in understanding the fundamental principles of embryonic development regulation in many multicellular organisms, including humans. Here are some important genes regulating embryonic development in Drosophila melanogaster and their modes of action:
Maternal genes: These genes are encoded in the female fruit fly and are present in the early stages of embryo development. They determine the embryo's main features and early development. For example, the gene called Bicoid regulates the formation of the embryo's anterior end, and its absence leads to an embryo lacking a head.
Zygotic genes: These genes are activated in later stages of embryo development when the fruit fly embryo begins to produce its own genetic products. For example, the hunchback gene regulates the formation of segments in the embryo.
Homeotic genes: This gene family regulates segmentation and axial patterning in development. They act as regulatory factors that determine cell fate in embryonic development. For example, the gene called Antennapedia regulates the formation of anterior limbs in the embryo.
Morphogens: These are molecules that form gradients in embryonic development and regulate cell fate depending on their position in the gradient. For example, the Hedgehog morphogen regulates the differentiation of segments and segment identity in the fruit fly embryo.
These genes and their modes of action form a complex regulatory network that guides the embryonic development of Drosophila melanogaster. They influence cell differentiation, segment formation, and axial patterning in the embryo, ultimately leading to the development of a fully formed adult fruit fly.
Males perform a sequence of five behavioral patterns to court females. First, males orient themselves while playing a courtship song by horizontally extending and vibrating their wings. Soon after, the male positions himself at the rear of the female's abdomen in a low posture to tap and lick the female genitalia. Finally, the male curls his abdomen and attempts copulation. Females can reject males by moving away, kicking, and extruding their ovipositor.[28] Copulation lasts around 15–20 minutes,[29] during which males transfer a few hundred, very long (1.76 mm) sperm cells in seminal fluid to the female.[30] Females store the sperm in a tubular receptacle and in two mushroom-shaped spermathecae; sperm from multiple matings compete for fertilization. A last male precedence is believed to exist; the last male to mate with a female sires about 80% of her offspring. This precedence was found to occur through both displacement and incapacitation.[31] The displacement is attributed to sperm handling by the female fly as multiple matings are conducted and is most significant during the first 1–2 days after copulation. Displacement from the seminal receptacle is more significant than displacement from the spermathecae.[31] Incapacitation of first male sperm by second male sperm becomes significant 2–7 days after copulation. The seminal fluid of the second male is believed to be responsible for this incapacitation mechanism (without removal of first male sperm) which takes effect before fertilization occurs.[31] The delay in effectiveness of the incapacitation mechanism is believed to be a protective mechanism that prevents a male fly from incapacitating his own sperm should he mate with the same female fly repetitively. Sensory neurons in the uterus of female D. melanogaster respond to a male protein, sex peptide, which is found in semen.[32] This protein makes the female reluctant to copulate for about 10 days after insemination. The signal pathway leading to this change in behavior has been determined. The signal is sent to a brain region that is a homolog of the hypothalamus and the hypothalamus then controls sexual behavior and desire.[32] Gonadotropic hormones in Drosophila maintain homeostasis and govern reproductive output via a cyclic interrelationship, not unlike the mammalian estrous cycle.[33] Sex peptide perturbs this homeostasis and dramatically shifts the endocrine state of the female by inciting juvenile hormone synthesis in the corpus allatum.[34]
D. melanogaster is often used for life extension studies, such as to identify genes purported to increase lifespan when mutated.[35] D. melanogaster is also used in studies of aging. Werner syndrome is a condition in humans characterized by accelerated aging. It is caused by mutations in the gene WRN that encodes a protein with essential roles in repair of DNA damage. Mutations in the D. melanogaster homolog of WRN also cause increased physiologic signs of aging, such as shorter lifespan, higher tumor incidence, muscle degeneration, reduced climbing ability, altered behavior and reduced locomotor activity.[36]
Meiosis[edit]
Meiotic recombination in D. melanogaster appears to be employed in repairing damage in germ-line DNA as indicated by the findings that meiotic recombination is induced by the DNA damaging agents ultraviolet light [37] and mitomycin C.[38]
Females[edit]

Females become receptive to courting males about 8–12 hours after emergence.[39] Specific neuron groups in females have been found to affect copulation behavior and mate choice. One such group in the abdominal nerve cord allows the female fly to pause her body movements to copulate.[32] Activation of these neurons induces the female to cease movement and orient herself towards the male to allow for mounting. If the group is inactivated, the female remains in motion and does not copulate. Various chemical signals such as male pheromones often are able to activate the group.[32]
Also, females exhibit mate choice copying. When virgin females are shown other females copulating with a certain type of male, they tend to copulate more with this type of male afterwards than naïve females (which have not observed the copulation of others). This behavior is sensitive to environmental conditions, and females copulate less in bad weather conditions.[40]
Males[edit]
This section needs additional citations for verification. (October 2015) |
D. melanogaster males exhibit a strong reproductive learning curve. That is, with sexual experience, these flies tend to modify their future mating behavior in multiple ways. These changes include increased selectivity for courting only intraspecifically, as well as decreased courtship times.
Sexually naïve D. melanogaster males are known to spend significant time courting interspecifically, such as with D. simulans flies. Naïve D. melanogaster will also attempt to court females that are not yet sexually mature, and other males. D. melanogaster males show little to no preference for D. melanogaster females over females of other species or even other male flies. However, after D. simulans or other flies incapable of copulation have rejected the males' advances, D. melanogaster males are much less likely to spend time courting nonspecifically in the future. This apparent learned behavior modification seems to be evolutionarily significant, as it allows the males to avoid investing energy into futile sexual encounters.[41]
In addition, males with previous sexual experience modify their courtship dance when attempting to mate with new females—the experienced males spend less time courting, so have lower mating latencies, meaning that they are able to reproduce more quickly. This decreased mating latency leads to a greater mating efficiency for experienced males over naïve males.[42] This modification also appears to have obvious evolutionary advantages, as increased mating efficiency is extremely important in the eyes of natural selection.
Polygamy[edit]
Both male and female D. melanogaster flies act polygamously (having multiple sexual partners at the same time).[43] In both males and females, polygamy results in a decrease in evening activity compared to virgin flies, more so in males than females.[43] Evening activity consists of those in which the flies participate other than mating and finding partners, such as finding food.[44] The reproductive success of males and females varies, because a female only needs to mate once to reach maximum fertility.[44] Mating with multiple partners provides no advantage over mating with one partner, so females exhibit no difference in evening activity between polygamous and monogamous individuals.[44] For males, however, mating with multiple partners increases their reproductive success by increasing the genetic diversity of their offspring.[44] This benefit of genetic diversity is an evolutionary advantage because it increases the chance that some of the offspring will have traits that increase their fitness in their environment.
The difference in evening activity between polygamous and monogamous male flies can be explained with courtship. For polygamous flies, their reproductive success increases by having offspring with multiple partners, and therefore they spend more time and energy on courting multiple females.[44] On the other hand, monogamous flies only court one female, and expend less energy doing so.[44] While it requires more energy for male flies to court multiple females, the overall reproductive benefits it produces has kept polygamy as the preferred sexual choice.[44]
The mechanism that affects courtship behavior in Drosophila is controlled by the oscillator neurons DN1s and LNDs.[45] Oscillation of the DN1 neurons was found to be effected by sociosexual interactions, and is connected to mating-related decrease of evening activity.[45]
Model organism in genetics[edit]
D. melanogaster remains one of the most studied organisms in biological research, particularly in genetics and developmental biology. It is also employed in studies of environmental mutagenesis.
History of use in genetic analysis[edit]

D. melanogaster was among the first organisms used for genetic analysis, and today it is one of the most widely used and genetically best-known of all eukaryotic organisms. All organisms use common genetic systems; therefore, comprehending processes such as transcription and replication in fruit flies helps in understanding these processes in other eukaryotes, including humans.[46]
Thomas Hunt Morgan began using fruit flies in experimental studies of heredity at Columbia University in 1910 in a laboratory known as the Fly Room. The Fly Room was cramped with eight desks, each occupied by students and their experiments. They started off experiments using milk bottles to rear the fruit flies and handheld lenses for observing their traits. The lenses were later replaced by microscopes, which enhanced their observations. Morgan and his students eventually elucidated many basic principles of heredity, including sex-linked inheritance, epistasis, multiple alleles, and gene mapping.[46]
D. melanogaster had historically been used in laboratories to study genetics and patterns of inheritance. However, D. melanogaster also has importance in environmental mutagenesis research, allowing researchers to study the effects of specific environmental mutagens.[47]
Reasons for use in laboratories[edit]

There are many reasons the fruit fly is a popular choice as a model organism:
- Its care and culture require little equipment, space, and expense even when using large cultures.
- It can be safely and readily anesthetized (usually with ether, carbon dioxide gas, by cooling, or with products such as FlyNap).
- Its morphology is easy to identify once anesthetized.
- It has a short generation time (about 10 days at room temperature), so several generations can be studied within a few weeks.
- It has a high fecundity (females lay up to 100 eggs per day, and perhaps 2000 in a lifetime).[11]
- Males and females are readily distinguished, and virgin females can be easily identified by their light-colored, translucent abdomen, facilitating genetic crossing.
- The mature larva has giant chromosomes in the salivary glands called polytene chromosomes, "puffs", which indicate regions of transcription, hence gene activity. The under-replication of rDNA occurs resulting in only 20% of DNA compared to the brain. Compare to the 47%, less rDNA in Sarcophaga barbata ovaries.
- It has only four pairs of chromosomes – three autosomes, and one pair of sex chromosomes.
- Males do not show meiotic recombination, facilitating genetic studies.
- Recessive lethal "balancer chromosomes" carrying visible genetic markers can be used to keep stocks of lethal alleles in a heterozygous state without recombination due to multiple inversions in the balancer.
- The development of this organism—from fertilized egg to mature adult—is well understood.
- Genetic transformation techniques have been available since 1987. One approach of inserting foreign genes into the Drosophila genome involves P elements. The transposable P elements, also known as transposons, are segments of bacterial DNA that are transferred into the fly genome. Transgenic flies have already contributed to many scientific advances, e.g., modeling such human diseases as Parkinson's, neoplasia, obesity, and diabetes.
- Its complete genome was sequenced and first published in 2000.[48]
- Sexual mosaics can be readily produced, providing an additional tool for studying the development and behavior of these flies.[49]
Genetic markers[edit]

Genetic markers are commonly used in Drosophila research, for example within balancer chromosomes or P-element inserts, and most phenotypes are easily identifiable either with the naked eye or under a microscope. In the list of a few common markers below, the allele symbol is followed by the name of the gene affected and a description of its phenotype. (Note: Recessive alleles are in lower case, while dominant alleles are capitalised.)
- Cy1: Curly; the wings curve away from the body, flight may be somewhat impaired
- e1: Ebony; black body and wings (heterozygotes are also visibly darker than wild type)
- Sb1: Stubble; bristles are shorter and thicker than wild type
- w1: White; eyes lack pigmentation and appear white
- bw: Brown; eye color determined by various pigments combined.
- y1: Yellow; body pigmentation and wings appear yellow, the fly analog of albinism
Classic genetic mutations[edit]
Drosophila genes are traditionally named after the phenotype they cause when mutated. For example, the absence of a particular gene in Drosophila will result in a mutant embryo that does not develop a heart. Scientists have thus called this gene tinman, named after the Oz character of the same name.[51] Likewise changes in the Shavenbaby gene cause the loss of dorsal cuticular hairs in Drosophila sechellia larvae.[52] This system of nomenclature results in a wider range of gene names than in other organisms.
- b: black- The black mutation was discovered in 1910 by Thomas Hunt Morgan.[53] The black mutation results in a darker colored body, wings, veins, and segments of the fruit fly's leg.[54] This occurs due to the fly's inability to create beta-alanine, a beta amino acid.[53] The phenotypic expression of this mutation varies based on the genotype of the individual; for example, whether the specimen is homozygotic or heterozygotic results in a darker or less dark appearance.[54] This genetic mutation is x-linked recessive.[55]
- bw: brown- The brown eye mutation results from inability to produce or synthesize pteridine (red) pigments, due to a point mutation on chromosome II.[56] When the mutation is homozygous, the pteridine pigments are unable to be synthesized because in the beginning of the pteridine pathway, a defective enzyme is being coded by homozygous recessive genes.[57][unreliable source?] In all, mutations in the pteridine pathway produces a darker eye color, hence the resulting color of the biochemical defect in the pteridine pathway being brown.
- m: miniature- One of the first records of the miniature mutation of wings was also made by Thomas Hunt Morgan in 1911. He described the wings as having a similar shape as the wild-type phenotype. However, their miniature designation refers to the lengths of their wings, which do not stretch beyond their body and, thus, are notably shorter than the wild-type length. He also noted its inheritance is connected to the sex of the fly and could be paired with the inheritance of other sex-determined traits such as white eyes.[58] The wings may also demonstrate other characteristics deviant from the wild-type wing, such as a duller and cloudier color.[59] Miniature wings are 1.5x shorter than wild-type but are believed to have the same number of cells. This is due to the lack of complete flattening by these cells, making the overall structure of the wing seem shorter in comparison. The pathway of wing expansion is regulated by a signal-receptor pathway, where the neurohormone bursicon interacts with its complementary G protein-coupled receptor; this receptor drives one of the G-protein subunits to signal further enzyme activity and results in development in the wing, such as apoptosis and growth.[60]
- se: sepia- The eye color of the sepia mutant is sepia, a reddish-brown color. In wild-type flies, ommochromes (brown) and drosopterins (red) give the eyes the typical red color.[61][62] The drosopterins are made via a pathway that involves a pyrimidodiazepine synthase,[63] which is encoded on chromosome 3L. The gene has a premature stop codon in sepia flies, so that the flies cannot produce the pyrimidodiazepine synthase and thus no red pigment, so that the eyes stay sepia.[61] The sepia allele is recessive and thus offspring from sepia flies and homozygous wild type flies, has red eyes. The sepia phenotype does not depend on the sex of the fly.[64]
- v: vermilion- The vermilion mutants cannot produce the brown ommochromes leaving the red drosopterins so that the eyes are vermilion colored (a radiant red) compared to a wild-type D. melanogaster. The vermilion mutation is sex-linked and recessive. The gene that is defect lies on the X chromosome.[65] The brown ommochromes are synthesised from kynurenine, which is made from tryptophane. Vermilion flies cannot convert tryptophane into kynurenine and thus cannot make ommochromes, either.[65] Vermilion mutants live longer than wild-type flies. This longer life span may be associated with the reduced amount of tryptophan converted to kynurenine in vermilion flies.[66]

- vg: vestigial- A spontaneous mutation, discovered in 1919 by Thomas Morgan and Calvin Bridges. Vestigial wings are those not fully developed and that have lost function. Since the discovery of the vestigial gene in Drosophila melanogaster, there have been many discoveries of the vestigial gene in other vertebrates and their functions within the vertebrates.[67] The vestigial gene is considered to be one of the most important genes for wing formation, but when it becomes over expressed the issue of ectopic wings begin to form.[68] The vestigial gene acts to regulate the expression of the wing imaginal discs in the embryo and acts with other genes to regulate the development of the wings. A mutated vestigial allele removes an essential sequence of the DNA required for correct development of the wings.[69]
- w: white- Drosophila melanogaster wild type typically expresses a brick red eye color. The white eye mutation in fruit flies is caused due to the absence of two pigments associated with red and brown eye colors; peridines (red) and ommochromes (brown).[62] In January 1910, Thomas Hunt Morgan first discovered the white gene and denoted it as w. The discovery of the white-eye mutation by Morgan brought about the beginnings of genetic experimentation and analysis of Drosophila melanogaster. Hunt eventually discovered that the gene followed a similar pattern of inheritance related to the meiotic segregation of the X chromosome. He discovered that the gene was located on the X chromosome with this information. This led to the discovery of sex-linked genes and also to the discovery of other mutations in Drosophila melanogaster.[70] The white-eye mutation leads to several disadvantages in flies, such as a reduced climbing ability, shortened life span, and lowered resistance to stress when compared to wild type flies.[71] Drosophila melanogaster has a series of mating behaviors that enable them to copulate within a given environment and therefore contribute to their fitness. After Morgan's discovery of the white-eye mutation being sex-linked, a study led by Sturtevant (1915) concluded that white-eyed males were less successful than wild-type males in terms of mating with females.[72] It was found that the greater the density in eye pigmentation, the greater the success in mating for the males of Drosophila melanogaster.[72]
- y: yellow- The yellow gene is a genetic mutation known as Dmel\y within the widely used data base called FlyBase. This mutation can be easily identified by the atypical yellow pigment observed in the cuticle of the adult flies and the mouth pieces of the larva.[73] The y mutation comprises the following phenotypic classes: the mutants that show a complete loss of pigmentation from the cuticle (y-type) and other mutants that show a mosaic pigment pattern with some regions of the cuticle (wild type, y2-type).[74] The role of the yellow gene is diverse and is responsible for changes in behaviour, sex-specific reproductive maturation and, epigenetic reprogramming.[75] The y gene is an ideal gene to study as it is visibly clear when an organism has this gene, making it easier to understand the passage of DNA to offspring.[75]

Genome[edit]
![]() D. melanogaster chromosomes to scale with megabase-pair references oriented as in the National Center for Biotechnology Information database, centimorgan distances are approximate and estimated from the locations of selected mapped loci. | |
NCBI genome ID | 47 |
---|---|
Ploidy | diploid |
Number of chromosomes | 8 |
Year of completion | 2015 |
The genome of D. melanogaster (sequenced in 2000, and curated at the FlyBase database[48]) contains four pairs of chromosomes – an X/Y pair, and three autosomes labeled 2, 3, and 4. The fourth chromosome is relatively very small and therefore often ignored, aside from its important eyeless gene. The D. melanogaster sequenced genome of 139.5 million base pairs has been annotated[76] and contains around 15,682 genes according to Ensemble release 73. More than 60% of the genome appears to be functional non-protein-coding DNA[77] involved in gene expression control. Determination of sex in Drosophila occurs by the X:A ratio of X chromosomes to autosomes, not because of the presence of a Y chromosome as in human sex determination. Although the Y chromosome is entirely heterochromatic, it contains at least 16 genes, many of which are thought to have male-related functions.[78]
There are three transferrin orthologs, all of which are dramatically divergent from those known in chordate models.[79]
Similarity to humans[edit]
A March 2000 study by National Human Genome Research Institute comparing the fruit fly and human genome estimated that about 60% of genes are conserved between the two species.[80] About 75% of known human disease genes have a recognizable match in the genome of fruit flies,[81] and 50% of fly protein sequences have mammalian homologs [citation needed]. An online database called Homophila is available to search for human disease gene homologues in flies and vice versa.[82]
Drosophila is being used as a genetic model for several human diseases including the neurodegenerative disorders Parkinson's, Huntington's, spinocerebellar ataxia and Alzheimer's disease.[83] The fly is also being used to study mechanisms underlying aging and oxidative stress, immunity, diabetes, and cancer, as well as drug abuse.[84][85][86]
Development[edit]
The life cycle of this insect has four stages: fertilized egg, larva, pupa, and adult.[13]
Embryogenesis in Drosophila has been extensively studied, as its small size, short generation time, and large brood size makes it ideal for genetic studies. It is also unique among model organisms in that cleavage occurs in a syncytium.
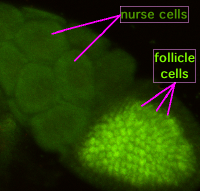
During oogenesis, cytoplasmic bridges called "ring canals" connect the forming oocyte to nurse cells. Nutrients and developmental control molecules move from the nurse cells into the oocyte. In the figure to the left, the forming oocyte can be seen to be covered by follicular support cells.
After fertilization of the oocyte, the early embryo (or syncytial embryo) undergoes rapid DNA replication and 13 nuclear divisions until about 5000 to 6000 nuclei accumulate in the unseparated cytoplasm of the embryo. By the end of the eighth division, most nuclei have migrated to the surface, surrounding the yolk sac (leaving behind only a few nuclei, which will become the yolk nuclei). After the 10th division, the pole cells form at the posterior end of the embryo, segregating the germ line from the syncytium. Finally, after the 13th division, cell membranes slowly invaginate, dividing the syncytium into individual somatic cells. Once this process is completed, gastrulation starts.[87]
Nuclear division in the early Drosophila embryo happens so quickly, no proper checkpoints exist, so mistakes may be made in division of the DNA. To get around this problem, the nuclei that have made a mistake detach from their centrosomes and fall into the centre of the embryo (yolk sac), which will not form part of the fly.
The gene network (transcriptional and protein interactions) governing the early development of the fruit fly embryo is one of the best understood gene networks to date, especially the patterning along the anteroposterior (AP) and dorsoventral (DV) axes (See under morphogenesis).[87]
The embryo undergoes well-characterized morphogenetic movements during gastrulation and early development, including germ-band extension, formation of several furrows, ventral invagination of the mesoderm, and posterior and anterior invagination of endoderm (gut), as well as extensive body segmentation until finally hatching from the surrounding cuticle into a first-instar larva.
During larval development, tissues known as imaginal discs grow inside the larva. Imaginal discs develop to form most structures of the adult body, such as the head, legs, wings, thorax, and genitalia. Cells of the imaginal disks are set aside during embryogenesis and continue to grow and divide during the larval stages—unlike most other cells of the larva, which have differentiated to perform specialized functions and grow without further cell division. At metamorphosis, the larva forms a pupa, inside which the larval tissues are reabsorbed and the imaginal tissues undergo extensive morphogenetic movements to form adult structures.
Developmental plasticity[edit]
Biotic and abiotic factors experienced during development will affect developmental resource allocation leading to phenotypic variation, also referred to as developmental plasticity.[88][89] As in all insects,[89] environmental factors can influence several aspects of development in Drosophila melanogaster.[90][91] Fruit flies reared under a hypoxia treatment experience decreased thorax length, while hyperoxia produces smaller flight muscles, suggesting negative developmental effects of extreme oxygen levels.[92] Circadian rhythms are also subject to developmental plasticity. Light conditions during development affect daily activity patterns in Drosophila melanogaster, where flies raised under constant dark or light are less active as adults than those raised under a 12-hour light/dark cycle.[93]
Temperature is one of the most pervasive factors influencing arthropod development. In Drosophila melanogaster temperature-induced developmental plasticity can be beneficial and/or detrimental.[94][95] Most often lower developmental temperatures reduce growth rates which influence many other physiological factors.[96] For example, development at 25 °C increases walking speed, thermal performance breadth, and territorial success, while development at 18 °C increases body mass, wing size, all of which are tied to fitness.[91][94] Moreover, developing at certain low temperatures produces proportionally large wings which improve flight and reproductive performance at similarly low temperatures (See acclimation).[97]
While certain effects of developmental temperature, like body size, are irreversible in ectotherms, others can be reversible.[89][98] When Drosophila melanogaster develop at cold temperatures they will have greater cold tolerance, but if cold-reared flies are maintained at warmer temperatures their cold tolerance decreases and heat tolerance increases over time.[98][99] Because insects typically only mate in a specific range of temperatures, their cold/heat tolerance is an important trait in maximizing reproductive output.[100]
While the traits described above are expected to manifest similarly across sexes, developmental temperature can also produce sex-specific effects in D. melanogaster adults.
- Females- Ovariole number is significantly affected by developmental temperature in D. melanogaster.[101] Egg size is also affected by developmental temperature, and exacerbated when both parents develop at warm temperatures (See Maternal effect).[94] Under stressful temperatures, these structures will develop to smaller ultimate sizes and decrease a female's reproductive output.[101][94] Early fecundity (total eggs laid in first 10 days post-eclosion) is maximized when reared at 25 °C (versus 17 °C and 29 °C) regardless of adult temperature.[102] Across a wide range of developmental temperatures, females tend to have greater heat tolerance than males.[103]
- Males- Stressful developmental temperatures will cause sterility in D. melanogaster males; although the upper temperature limit can be increased by maintaining strains at high temperatures (See acclimation).[95] Male sterility can be reversible if adults are returned to an optimal temperature after developing at stressful temperatures.[104] Male flies are smaller and more successful at defending food/oviposition sites when reared at 25 °C versus 18 °C; thus smaller males will have increased mating success and reproductive output.[91]
Sex determination[edit]
Drosophila flies have both X and Y chromosomes, as well as autosomes. Unlike humans, the Y chromosome does not confer maleness; rather, it encodes genes necessary for making sperm. Sex is instead determined by the ratio of X chromosomes to autosomes.[105] Furthermore, each cell "decides" whether to be male or female independently of the rest of the organism, resulting in the occasional occurrence of gynandromorphs.
X Chromosomes | Autosomes | Ratio of X:A | Sex |
---|---|---|---|
XXXX | AAAA | 1 | Normal Female |
XXX | AAA | 1 | Normal Female |
XXY | AA | 1 | Normal Female |
XXYY | AA | 1 | Normal Female |
XX | AA | 1 | Normal Female |
XY | AA | 0.50 | Normal Male |
X | AA | 0.50 | Normal Male (sterile) |
XXX | AA | 1.50 | Metafemale |
XXXX | AAA | 1.33 | Metafemale |
XX | AAA | 0.66 | Intersex |
X | AAA | 0.33 | Metamale |
Three major genes are involved in determination of Drosophila sex. These are sex-lethal, sisterless, and deadpan. Deadpan is an autosomal gene which inhibits sex-lethal, while sisterless is carried on the X chromosome and inhibits the action of deadpan. An AAX cell has twice as much deadpan as sisterless, so sex-lethal will be inhibited, creating a male. However, an AAXX cell will produce enough sisterless to inhibit the action of deadpan, allowing the sex-lethal gene to be transcribed to create a female.
Later, control by deadpan and sisterless disappears and what becomes important is the form of the sex-lethal gene. A secondary promoter causes transcription in both males and females. Analysis of the cDNA has shown that different forms are expressed in males and females. Sex-lethal has been shown to affect the splicing of its own mRNA. In males, the third exon is included which encodes a stop codon, causing a truncated form to be produced. In the female version, the presence of sex-lethal causes this exon to be missed out; the other seven amino acids are produced as a full peptide chain, again giving a difference between males and females.[106]
Presence or absence of functional sex-lethal proteins now go on to affect the transcription of another protein known as doublesex. In the absence of sex-lethal, doublesex will have the fourth exon removed and be translated up to and including exon 6 (DSX-M[ale]), while in its presence the fourth exon which encodes a stop codon will produce a truncated version of the protein (DSX-F[emale]). DSX-F causes transcription of Yolk proteins 1 and 2 in somatic cells, which will be pumped into the oocyte on its production.
Immunity[edit]
The D. melanogaster immune system can be divided into two responses: humoral and cell-mediated. The former is a systemic response mediated in large part through the toll and Imd pathways, which are parallel systems for detecting microbes. Other pathways including the stress response pathways JAK-STAT and P38, nutritional signalling via FOXO, and JNK cell death signalling are all involved in key physiological responses to infection. D. melanogaster has an organ called the "fat body", which is analogous to the human liver. The fat body is the primary secretory organ and produces key immune molecules upon infection, such as serine proteases and antimicrobial peptides (AMPs). AMPs are secreted into the hemolymph and bind infectious bacteria and fungi, killing them by forming pores in their cell walls or inhibiting intracellular processes. The cellular immune response instead refers to the direct activity of blood cells (hemocytes) in Drosophila, which are analogous to mammalian monocytes/macrophages. Hemocytes also possess a significant role in mediating humoral immune responses such as the melanization reaction.[107]
The immune response to infection can involve up to 2,423 genes, or 13.7% of the genome. Although the fly's transcriptional response to microbial challenge is highly specific to individual pathogens, Drosophila differentially expresses a core group of 252 genes upon infection with most bacteria. This core group of genes is associated with gene ontology categories such as antimicrobial response, stress response, secretion, neuron-like, reproduction, and metabolism among others.[108][109] Drosophila also possesses several immune mechanisms to both shape the microbiota and prevent excessive immune responses upon detection of microbial stimuli. For instance, secreted PGRPs with amidase activity scavenge and degrade immunostimulatory DAP-type PGN in order to block Imd activation.[110]
Unlike mammals, Drosophila have innate immunity but lack an adaptive immune response. However, the core elements of this innate immune response are conserved between humans and fruit flies. As a result, the fruit fly offers a useful model of innate immunity for disentangling genetic interactions of signalling and effector function, as flies do not have to contend with interference of adaptive immune mechanisms that could confuse results. Various genetic tools, protocols, and assays make Drosophila a classical model for studying the innate immune system,[111] which has even included immune research on the international space station.[112]
JAK-STAT signalling[edit]
Multiple elements of the Drosophila JAK-STAT signalling pathway bear direct homology to human JAK-STAT pathway genes. JAK-STAT signalling is induced upon various organismal stresses such as heat stress, dehydration, or infection. JAK-STAT induction leads to the production of a number of stress response proteins including Thioester-containing proteins (TEPs),[113] Turandots,[114] and the putative antimicrobial peptide Listericin.[115] The mechanisms through which many of these proteins act is still under investigation. For instance, the TEPs appear to promote phagocytosis of Gram-positive bacteria and the induction of the toll pathway. As a consequence, flies lacking TEPs are susceptible to infection by toll pathway challenges.[113]
The cellular response to infection[edit]
Circulating hemocytes are key regulators of infection. This has been demonstrated both through genetic tools to generate flies lacking hemocytes, or through injecting microglass beads or lipid droplets that saturate hemocyte ability to phagocytose a secondary infection.[116][117] Flies treated like this fail to phagocytose bacteria upon infection, and are correspondingly susceptible to infection.[118] These hemocytes derive from two waves of hematopoiesis, one occurring in the early embryo and one occurring during development from larva to adult.[119] However Drosophila hemocytes do not renew over the adult lifespan, and so the fly has a finite number of hemocytes that decrease over the course of its lifespan.[120] Hemocytes are also involved in regulating cell-cycle events and apoptosis of aberrant tissue (e.g. cancerous cells) by producing Eiger, a tumor necrosis factor signalling molecule that promotes JNK signalling and ultimately cell death and apoptosis.[121]
Behavioral genetics and neuroscience[edit]
In 1971, Ron Konopka and Seymour Benzer published "Clock mutants of Drosophila melanogaster", a paper describing the first mutations that affected an animal's behavior. Wild-type flies show an activity rhythm with a frequency of about a day (24 hours). They found mutants with faster and slower rhythms, as well as broken rhythms—flies that move and rest in random spurts. Work over the following 30 years has shown that these mutations (and others like them) affect a group of genes and their products that form a biochemical or biological clock. This clock is found in a wide range of fly cells, but the clock-bearing cells that control activity are several dozen neurons in the fly's central brain.
Since then, Benzer and others have used behavioral screens to isolate genes involved in vision, olfaction, audition, learning/memory, courtship, pain, and other processes, such as longevity.
Following the pioneering work of Alfred Henry Sturtevant[122] and others, Benzer and colleagues[49] used sexual mosaics to develop a novel fate mapping technique. This technique made it possible to assign a particular characteristic to a specific anatomical location. For example, this technique showed that male courtship behavior is controlled by the brain.[49] Mosaic fate mapping also provided the first indication of the existence of pheromones in this species.[123] Males distinguish between conspecific males and females and direct persistent courtship preferentially toward females thanks to a female-specific sex pheromone which is mostly produced by the female's tergites.
The first learning and memory mutants (dunce, rutabaga, etc.) were isolated by William "Chip" Quinn while in Benzer's lab, and were eventually shown to encode components of an intracellular signaling pathway involving cyclic AMP, protein kinase A, and a transcription factor known as CREB. These molecules were shown to be also involved in synaptic plasticity in Aplysia and mammals.[124]
The Nobel Prize in Physiology or Medicine for 2017 was awarded to Jeffrey C. Hall, Michael Rosbash, Michael W. Young for their works using fruit flies in understanding the "molecular mechanisms controlling the circadian rhythm".[125]
Male flies sing to the females during courtship using their wings to generate sound, and some of the genetics of sexual behavior have been characterized. In particular, the fruitless gene has several different splice forms, and male flies expressing female splice forms have female-like behavior and vice versa. The TRP channels nompC, nanchung, and inactive are expressed in sound-sensitive Johnston's organ neurons and participate in the transduction of sound.[126][127] Mutating the Genderblind gene, also known as CG6070, alters the sexual behavior of Drosophila, turning the flies bisexual.[128]
Flies use a modified version of Bloom filters to detect novelty of odors, with additional features including similarity of novel odor to that of previously experienced examples, and time elapsed since previous experience of the same odor.[129]
Aggression[edit]
As with most insects, aggressive behaviors between male flies commonly occur in the presence of courting a female and when competing for resources. Such behaviors often involve raising wings and legs towards the opponent and attacking with the whole body.[130] Thus, it often causes wing damage, which reduces their fitness by removing their ability to fly and mate.[131]
Acoustic communication[edit]
In order for aggression to occur, male flies produce sounds to communicate their intent. A 2017 study found that songs promoting aggression contain pulses occurring at longer intervals.[132] RNA sequencing from fly mutants displaying over-aggressive behaviors found more than 50 auditory-related genes (important for transient receptor potentials, Ca2+ signaling, and mechanoreceptor potentials) to be upregulated in the AB neurons located in Johnston's organ.[132] In addition, aggression levels were reduced when these genes were knocked out via RNA interference.[132] This signifies the major role of hearing as a sensory modality in communicating aggression.
Pheromone signaling[edit]
Other than hearing, another sensory modality that regulates aggression is pheromone signaling, which operates through either the olfactory system or the gustatory system depending on the pheromone.[133] An example is cVA, an anti-aphrodisiac pheromone used by males to mark females after copulation and to deter other males from mating.[134] This male-specific pheromone causes an increase in male-male aggression when detected by another male's gustatory system.[133] However, upon inserting a mutation that makes the flies irresponsive to cVA, no aggressive behaviors were seen.[135] This shows how there are multiple modalities for promoting aggression in flies.
Competition for food[edit]
Specifically, when competing for food, aggression occurs based on amount of food available and is independent of any social interactions between males.[136] Specifically, sucrose was found to stimulate gustatory receptor neurons, which was necessary to stimulate aggression.[136] However, once the amount of food becomes greater than a certain amount, the competition between males lowers.[136] This is possibly due to an over-abundance of food resources. On a larger scale, food was found to determine the boundaries of a territory since flies were observed to be more aggressive at the food's physical perimeter.
Effect of sleep deprivation[edit]
However, like most behaviors requiring arousal and wakefulness, aggression was found to be impaired via sleep deprivation. Specifically, this occurs through the impairment of Octopamine and dopamine signaling, which are important pathways for regulating arousal in insects.[137][138] Due to reduced aggression, sleep-deprived male flies were found to be disadvantaged at mating compared to normal flies.[138] However, when octopamine agonists were administered upon these sleep-deprived flies, aggression levels were seen to be increased and sexual fitness was subsequently restored.[138] Therefore, this finding implicates the importance of sleep in aggression between male flies.
Vision[edit]

The compound eye of the fruit fly contains 760 unit eyes or ommatidia, and are one of the most advanced among insects. Each ommatidium contains eight photoreceptor cells (R1-8), support cells, pigment cells, and a cornea. Wild-type flies have reddish pigment cells, which serve to absorb excess blue light so the fly is not blinded by ambient light. Eye color genes regulate cellular vesicular transport. The enzymes needed for pigment synthesis are then transported to the cell's pigment granule, which holds pigment precursor molecules.[62]
Each photoreceptor cell consists of two main sections, the cell body and the rhabdomere. The cell body contains the nucleus, while the 100-μm-long rhabdomere is made up of toothbrush-like stacks of membrane called microvilli. Each microvillus is 1–2 μm in length and about 60 nm in diameter.[139] The membrane of the rhabdomere is packed with about 100 million opsin molecules, the visual protein that absorbs light. The other visual proteins are also tightly packed into the microvilli, leaving little room for cytoplasm.
Opsins and spectral sensitivity[edit]


The genome of Drosophila encodes seven opsins,[141] five of those are expressed in the omatidia of the eye. The photoreceptor cells R1-R6 express the opsin Rh1,[142] which absorbs maximally blue light (around 480 nm),[143][144][145] however the R1-R6 cells cover a broader range of the spectrum than an opsin would allow due to a sensitising pigment[146][147] that adds two sensitivity maxima in the UV-range (355 and 370 nm).[145] The R7 cells come in two types with yellow and pale rhabdomeres (R7y and R7p).[148][149] The pale R7p cells express the opsin Rh3,[150][151] which maximally absorbs UV-light (345 nm).[152] The R7p cells are strictly paired with the R8p cells that express Rh5,[151] which maximally absorbs violet light (437 nm).[145] The other, the yellow R7y cells express a blue-absorbing screening pigment[148] and the opsin Rh4,[153] which maximally absorbs UV-light (375 nm).[152] The R7y cells are strictly paired with R8y cells that express Rh6,[154] which maximally absorbs UV-light (508 nm).[145] In a subset of omatidia both R7 and R8 cells express the opsin Rh3.[151]
However, these absorption maxima of the opsins where measured in white eyed flies without screening pigments (Rh3-Rh6),[152][145] or from the isolated opsin directly (Rh1).[143] Those pigments reduce the light that reaches the opsins depending on the wavelength. Thus in fully pigmented flies, the effective absorption maxima of opsins differs and thus also the sensitivity of their photoreceptor cells. With screening pigment, the opsin Rh3 is short wave shifted from 345 nm[b] to 330 nm and Rh4 from 375 nm to 355 nm. Whether screening pigment is present does not make a practical difference for the opsin Rh5 (435 nm and 437 nm), while the opsin R6 is long wave shifted by 92 nm from 508 nm to 600 nm.[140]
Additionally of the opsins of the eye, Drosophila has two more opsins: The ocelli express the opsin Rh2,[155][156] which maximally absorbs violet light (~420 nm).[156] And the opsin Rh7, which maximally absorbs UV-light (350 nm) with an unusually long wavelength tail up to 500 nm. The long tail disappears if a lysine at position 90 is replaced by glutamic acid. This mutant then absorbs maximally violet light (450 nm).[157] The opsin Rh7 entrains with cryptochrome the circadian rhythm of Drosophila to the day-night-cycle in the central pacemaker neurons.[158]
Each Drosophila opsin binds the carotenoid chromophore 11-cis-3-hydroxyretinal via a lysine.[159][160] This lysine is conserved in almost all opsins, only a few opsins have lost it during evolution.[161] Opsins without it are not light sensitive.[162][163][164] In particular, the Drosophila opsins Rh1, Rh4, and Rh7 function not only as photoreceptors, but also as chemoreceptors for aristolochic acid. These opsins still have the lysine like other opsins. However, if it is replaced by an arginine in Rh1, then Rh1 loses light sensitivity but still responds to aristolochic acid. Thus, the lysine is not needed for Rh1 to function as chemoreceptor.[163]
- Spectral sensitivities of Drosophila melanogaster opsins in white eyed flies. The sensitivities of Rh3–R6 are modelled with opsin templates and sensitivity estimates from Salcedo et al. (1999).[145] The opsin Rh1 (redrawn from Salcedo et al.[145]) has a characteristic shape as it is coupled to a UV-sensitising pigment.
- Normalized mean spectral sensitivity curves of Drosophila melanogaster opsins Rh1, Rh3, Rh4, Rh5, and Rh6 measured in their native photoreceptor cells in red eye flies with screening pigment. Each spectral curve is the average from six flies.
Phototransduction[edit]
As in vertebrate vision, visual transduction in invertebrates occurs via a G protein-coupled pathway. However, in vertebrates, the G protein is transducin, while the G protein in invertebrates is Gq (dgq in Drosophila). When rhodopsin (Rh) absorbs a photon of light its chromophore, 11-cis-3-hydroxyretinal, is isomerized to all-trans-3-hydroxyretinal. Rh undergoes a conformational change into its active form, metarhodopsin. Metarhodopsin activates Gq, which in turn activates a phospholipase Cβ (PLCβ) known as NorpA.[165]
PLCβ hydrolyzes phosphatidylinositol (4,5)-bisphosphate (PIP2), a phospholipid found in the cell membrane, into soluble inositol triphosphate (IP3) and diacylglycerol (DAG), which stays in the cell membrane. DAG, a derivative of DAG, or PIP2 depletion cause a calcium-selective ion channel known as transient receptor potential (TRP) to open and calcium and sodium flows into the cell.[166] IP3 is thought to bind to IP3 receptors in the subrhabdomeric cisternae, an extension of the endoplasmic reticulum, and cause release of calcium, but this process does not seem to be essential for normal vision.[165]
Кальций связывается с такими белками, как кальмодулин (CaM) и специфической для глаз протеинкиназой C (PKC), известной как InaC. Эти белки взаимодействуют с другими белками и, как было показано, необходимы для отключения светового ответа. Кроме того, белки, называемые аррестинами, связывают метародопсин и не позволяют ему активировать больше Gq. Натриево -кальциевый обменник , известный как CalX, выкачивает кальций из клетки. Он использует внутренний градиент натрия для экспорта кальция со стехиометрией 3 Na. + / 1 Калифорния ++ . [167]
TRP, InaC и PLC образуют сигнальный комплекс, связывая каркасный белок InaD. InaD содержит пять связывающих доменов, называемых белками домена PDZ , которые специфически связывают C-концы белков-мишеней. Разрушение комплекса мутациями либо в доменах PDZ, либо в целевых белках снижает эффективность передачи сигналов. Например, нарушение взаимодействия между InaC, протеинкиназой C и InaD приводит к задержке инактивации светового ответа .
В отличие от метародопсина позвоночных, метародопсин беспозвоночных может превращаться обратно в родопсин путем поглощения фотона оранжевого света (580 нм).
Около двух третей мозга дрозофилы отвечает за обработку зрительной информации. [168] Хотя пространственное разрешение их зрения значительно хуже, чем у людей, их временное разрешение примерно в 10 раз лучше.
Уход [ править ]
Известно, что дрозофилы демонстрируют предсказуемое поведение по уходу. Дрозофила последовательно начинает процедуру ухода, используя передние ноги для очистки глаз, затем головы и усиков. Используя задние лапы, дрозофилы приступают к уходу за брюшком, а затем за крыльями и грудной клеткой. На протяжении всей этой процедуры дрозофилы периодически трутся ногами, чтобы избавиться от лишней пыли и мусора, которые накапливаются в процессе ухода. [169]
Было показано, что уходовое поведение реализуется в иерархии подавления. Это означает, что поведение ухода, которое происходит в начале последовательности, предотвращает одновременное возникновение тех, которые следуют позже в последовательности, поскольку последовательность ухода состоит из взаимоисключающих действий. [170] [171] Эта иерархия не мешает дрозофиле вернуться к поведению по уходу, которое уже использовалось в последовательности ухода. [170] Считается, что порядок ухода за собой в иерархии подавления связан с приоритетом очистки определенной части тела. Например, глаза и усики, вероятно, создаются на ранних этапах процедуры ухода, чтобы предотвратить вмешательство мусора в работу органов чувств D. melanogaster . [170] [171]
Прогулка [ править ]

Как и многие другие шестиногие насекомые, дрозофила обычно ходит на треноге . [173] Это означает, что три ноги качаются вместе, а остальные три остаются неподвижными или в стойке. В частности, средняя нога движется синхронно с контралатеральными передними и задними ногами. Однако изменчивость конфигурации штатива существует в континууме, а это означает, что у мух нет явных переходов между разными походками. [174] При высоких скоростях ходьбы конфигурация ходьбы в основном представляет собой штатив (3 ноги в стойке), но при более медленных скоростях ходьбы мухи, скорее всего, будут иметь четыре (тетрапод) или пять ног в стойке (волна). [175] [176] Эти переходы могут помочь оптимизировать статическую стабильность . [177] Поскольку мухи настолько малы, силы инерции ничтожны по сравнению с силами упругости их мышц и суставов или силами вязкости окружающего воздуха. [178]
Полет [ править ]
Мухи летают, совершая прямые последовательности движений, чередующиеся с быстрыми поворотами, называемыми саккадами. [179] Во время этих поворотов муха способна повернуть на 90° менее чем за 50 миллисекунд. [179]
В характеристиках полета дрозофилы может преобладать вязкость воздуха , а не инерция тела мухи, но может произойти и противоположный случай, когда инерция является доминирующей силой. [179] Однако последующие работы показали, что, хотя вязкое воздействие на тело насекомого во время полета может быть незначительным, аэродинамические силы на самих крыльях фактически вызывают вязкое демпфирование поворотов плодовых мух. [180]
Коннектом [ править ]
Дрозофила — одно из немногих животных ( в том числе C. elegans подробные нейронные цепи ( коннектом ), у которых имеются ).
Для полноценного мозга мух существует коннектом высокого уровня на уровне отделов мозга и соединяющихся участков нейронов. [181] Версия этого доступна в Интернете. [182]
существуют подробные коннектомы на уровне схемы. Для пластинки [183] [184] и мозговое вещество [185] столбик, как в зрительной системе плодовой мушки, так и в альфа-доле тела гриба. [186]
В мае 2017 года в статье, опубликованной в bioRxiv, была представлена стопка изображений электронной микроскопии всего мозга взрослой женщины с синаптическим разрешением. Том доступен для разреженного отслеживания выбранных цепей. [187] [188] С тех пор было собрано несколько наборов данных, включая плотный коннектом половины центрального мозга дрозофилы в 2020 году. [189] [190] и плотный коннектом всей нервной цепочки взрослой женщины в 2021 году. [191] Обычно эти наборы данных получают путем разделения ткани (например, мозга) на тонкие срезы (порядка десяти или сотен нанометров). Затем каждый срез визуализируется с помощью электронного микроскопа, и эти изображения сшиваются и выравниваются вместе, чтобы создать объем трехмерного изображения. Ниже приведены методы, использованные при реконструкции и первоначальном анализе таких наборов данных. [192] Благодаря достижениям в области глубокого обучения автоматизированные методы сегментации изображений позволили провести крупномасштабную реконструкцию, обеспечивающую плотную реконструкцию всех нейритов в объеме. [193] Кроме того, разрешение электронной микроскопии выявляет ультраструктурные различия между нейронами, а также расположение отдельных синапсов, тем самым обеспечивая диаграмму соединений синаптических связей между всеми нейритами в данном наборе данных.
В 2023 году была опубликована полная карта мозга личинки дрозофилы на уровне синапсов и анализ его архитектуры. Личиночный мозг состоит из 3016 нейронов и 548 000 синаптических участков. [194] тогда как мозг взрослого человека имеет около 150 000 нейронов и 150 миллионов синапсов.
Заблуждения [ править ]
Дрозофилу иногда называют вредителем из-за ее склонности жить в населенных пунктах, где встречаются бродящие фрукты. Мухи могут собираться в домах, ресторанах, магазинах и других местах. [14] Название и поведение этого вида мух привели к ошибочному представлению о том, что он представляет угрозу биологической безопасности в Австралии и других странах. В то время как другие виды «плодовых мушек» действительно представляют риск, D. melanogaster привлекают уже гниющие фрукты, а не вызывают их гниение. [195] [196]
См. также [ править ]
- Испытания на животных на беспозвоночных
- Пищевое поведение насекомых (Измерение)
- Фруктовые мушки в космосе
- Генетически модифицированное насекомое
- Гинандроморфизм
- ген JETLAG
- Список баз данных дрозофилы
- Шпецле (ген)
- Время летит, как стрела; фруктовые мушки, как банан
- Трансгенезис
- Рыбка данио - еще один широко используемый модельный организм в научных исследованиях.
Примечания [ править ]
- ^ Несколько недавних публикаций предпочитают «уксусную мушку» как более точное описание, чем «дрозофила». [3] [4] [5]
- ^ Шарки и др. [140] в разделе результатов укажите максимум поглощения Rh3 как 334 нм. Однако во введении и в разделе «Материалы и методы» она указана как 345 нм. Для обоих значений они цитируют Фейлера и др., которые сообщили только о длине волны 345 нм. [152] Поэтому похоже это ошибка и там наверное тоже имели в виду 345 нм.
Ссылки [ править ]
- ^ Мейген Дж. В. (1830). Систематическое описание известных европейских двукрылых насекомых. (Том 6) (PDF) (на немецком языке). Шульц чудо-человек. Архивировано из оригинала (PDF) 9 февраля 2012 г.
- ^ «Дрозофила (Sophophora) melanogaster Meigen, 1830» . Каталог жизни . Вид 2000 г .: Лейден, Нидерланды . Проверено 2 мая 2024 г.
- ^ «Дрозофила | род насекомых» . Британская энциклопедия . Проверено 30 октября 2021 г.
- ^ «Уксусные мухи» . Расширение штата Пенсильвания . Проверено 30 октября 2021 г.
- ↑ Перейти обратно: Перейти обратно: а б Грин ММ (сентябрь 2002 г.). «Это действительно не плодовая мушка» . Генетика . 162 (1): 1–3. дои : 10.1093/генетика/162.1.1 . ПМЦ 1462251 . ПМИД 12242218 .
- ^ Шильтуизен, Менно (28 апреля 2015 г.). «Химический коктейль из спермы может захватить мозг партнера» . Обнаружить . Проверено 11 сентября 2023 г.
Даже в эякуляте скромной банановой мухи Drosophila melanogaster исследователи выявили не менее 133 различных видов белков.
- ^ Биография Т.Х. Моргана, получившая Нобелевскую премию, с упоминанием К.В. Вудворта
- ^ Холден Б. (1 января 2015 г.). Чарльз В. Вудворт: Замечательная жизнь первого энтомолога Калифорнийского университета (1-е изд.). Издательство Брайана Холдена. стр. 135–137. ISBN 978-0-9864105-3-6 .
- ^ «Нобелевские премии» . Хранитель . 7 октября 2017 г.
- ^ «FruitFly-ResearchGate» .
- ↑ Перейти обратно: Перейти обратно: а б Санг Дж. Х., Рив EC (23 июня 2001 г.). «Drosophila melanogaster: плодовая мушка» . Энциклопедия генетики . США: Fitzroy Dearborn Publishers, I.p. 157. ИСБН 978-1-884964-34-3 . Проверено 1 июля 2009 г.
- ^ Бодри Э., Вижинье Б., Вей М. (август 2004 г.). «Неафриканские популяции Drosophila melanogaster имеют уникальное происхождение» . Молекулярная биология и эволюция . 21 (8): 1482–91. дои : 10.1093/molbev/msh089 . ПМИД 15014160 .
- ↑ Перейти обратно: Перейти обратно: а б Марков Т.А. (июнь 2015 г.). «Тайная жизнь мух-дрозофил» . электронная жизнь . 4 . doi : 10.7554/eLife.06793 . ПМЦ 4454838 . ПМИД 26041333 .
- ↑ Перейти обратно: Перейти обратно: а б «Уксусные мухи, виды дрозофилы , семейство: Drosophilaidae » . Кафедра энтомологии Колледжа сельскохозяйственных наук Университета штата Пенсильвания. 2017 . Проверено 20 июля 2017 г.
- ↑ Перейти обратно: Перейти обратно: а б Юарт Г.Д., Хауэллс А.Дж. (1 января 1998 г.). «Транспортеры ABC, участвующие в транспортировке предшественников глазного пигмента у Drosophila melanogaster». Транспортеры ABC: биохимические, клеточные и молекулярные аспекты . Методы энзимологии. Том. 292. Академик Пресс. стр. 213–24. дои : 10.1016/S0076-6879(98)92017-1 . ISBN 978-0-12-182193-7 . ПМИД 9711556 .
- ^ «FlyBase: база данных генов и геномов дрозофилы» . Генетическое общество Америки. 2009. Архивировано из оригинала 15 августа 2009 года . Проверено 11 августа 2009 г.
- ^ Юзуки, Кевен; Тидон, Розана (2020). «Ключ для идентификации видов дрозофилид (Diptera, Drosophilidae), экзотических для Неотропического региона и встречающихся в Бразилии» . Revista Brasileira de Entomologia . 64 (1). doi : 10.1590/1806-9665-rbent-2019-100 . ISSN 1806-9665 . S2CID 211570766 .
- ^ Миллер, Мэн; Маршалл, ЮАР; Гримальди, Д.А. (2017). «Обзор видов дрозофил (Diptera: Drosophilidae) и родов дрозофилид северо-востока Северной Америки» . Канадский журнал идентификации членистоногих . 31 . дои : 10.3752/cjai.2017.31 .
- ^ «Дрозофила Меланогастер» . Сеть разнообразия животных. 2000. Архивировано из оригинала 30 ноября 2014 года . Проверено 11 августа 2009 г.
- ^ Линфорд, штат Нью-Джерси, Билгир С., Ро Джей, Плетчер С.Д. (январь 2013 г.). «Измерение продолжительности жизни Drosophila melanogaster» . Журнал визуализированных экспериментов (71). дои : 10.3791/50068 . ПМЦ 3582515 . ПМИД 23328955 .
- ↑ Перейти обратно: Перейти обратно: а б с д и ж г Эшбернер М. , Томпсон Дж. Н. (1978). «Лабораторная культура дрозофилы ». В Эшбернер М., Райт TRF (ред.). Генетика и биология дрозофилы . Том. 2А. Академическая пресса. 1–81.
- ↑ Перейти обратно: Перейти обратно: а б с д и ж г Эшбернер М. , Голич К.Г., Хоули Р.С. (2005). Дрозофила: Лабораторный справочник (2-е изд.). Лабораторный пресс Колд-Спринг-Харбор. стр. 162–4. ISBN 978-0-87969-706-8 .
- ^ в Блумингтоне дрозофил Центр запасов при Университете Индианы : Основные методы культивирования дрозофил. Архивировано 1 сентября 2006 г. в Wayback Machine.
- ↑ Перейти обратно: Перейти обратно: а б Чианг ХК, Ходсон AC (1950). «Аналитическое исследование роста популяции Drosophila melanogaster ». Экологические монографии . 20 (3): 173–206. Бибкод : 1950ЭкоМ...20..173С . дои : 10.2307/1948580 . JSTOR 1948580 .
- ^ Баккер К. (1961). «Анализ факторов, определяющих успех в борьбе за пищу личинок Drosophila melanogaster ». Архивы Néerlandaises de Zoologie . 14 (2): 200–281. дои : 10.1163/036551661X00061 . S2CID 85129022 .
- ^ Фернандес-Морено М.А., Фарр К.Л., Кагуни Л.С., Гаресс Р. (2007). «Drosophila melanogaster как модельная система для изучения митохондриальной биологии». Митохондрии . Методы молекулярной биологии. Том. 372. стр. 33–49. дои : 10.1007/978-1-59745-365-3_3 . ISBN 978-1-58829-667-2 . ПМК 4876951 . ПМИД 18314716 .
- ^ Блюм Дж. Э., Фишер К. Н., Майлз Дж., Хандельсман Дж. (ноябрь 2013 г.). «Частое пополнение поддерживает полезный микробиом Drosophila melanogaster» . мБио . 4 (6): e00860-13. дои : 10.1128/mBio.00860-13 . ПМЦ 3892787 . ПМИД 24194543 .
- ^ Кук Р., Коннолли К. (1973). «Реакция отторжения самок Drosophila melanogaster: их онтогенез, причинно-следственная связь и влияние на поведение ухаживающего самца». Поведение . 44 (1/2): 142–166. дои : 10.1163/156853973x00364 . JSTOR 4533484 . S2CID 85393769 .
- ^ Хоуот Б., Светец Н., Годой-Эррера Р., Фервер Дж.Ф. (июль 2010 г.). «Влияние лабораторной акклиматизации на изменение признаков, связанных с размножением Drosophila melanogaster» . Журнал экспериментальной биологии . 213 (Часть 13): 2322–31. дои : 10.1242/jeb.041566 . ПМИД 20543131 .
- ^ Гилберт С.Ф. (2006). «9: Оплодотворение дрозофилы» . Биология развития (8-е изд.). Синауэр Ассошиэйтс. ISBN 978-0-87893-250-4 . Архивировано из оригинала 7 февраля 2007 года.
- ↑ Перейти обратно: Перейти обратно: а б с Прайс К.С., Дайер К.А., Койн Дж.А. (июль 1999 г.). «Конкуренция сперматозоидов между самцами дрозофилы включает как смещение, так и выведение из строя». Природа . 400 (6743): 449–52. Бибкод : 1999Natur.400..449P . дои : 10.1038/22755 . ПМИД 10440373 . S2CID 4393369 .
- ↑ Перейти обратно: Перейти обратно: а б с д «Исследования плодовых мух могут показать, что происходит в мозгу самок во время ухаживания и спаривания» . Проверено 5 октября 2014 г.
- ^ Мейзельман М., Ли С.С., Тран Р.Т., Дай Х., Дин Ю., Ривера-Перес С. и др. (май 2017 г.). «Дрозофила меланогастер» . Труды Национальной академии наук Соединенных Штатов Америки . 114 (19): E3849–E3858. дои : 10.1073/pnas.1620760114 . ПМЦ 5441734 . ПМИД 28439025 .
- ^ Мошицкий П., Флейшманн И., Хаимов Н., Саудан П., Клаузер С., Кубли Э., Эпплбаум С.В. (1996). «Секс-пептид активирует биосинтез ювенильных гормонов у Drosophila melanogaster corpus allatum». Архив биохимии и физиологии насекомых . 32 (3–4): 363–74. doi : 10.1002/(SICI)1520-6327(1996)32:3/4<363::AID-ARCH9>3.0.CO;2-T . ПМИД 8756302 .
- ^ Карнс М.Ю., Кэмпбелл Т., Хуанг В., Батлер Д.Г., Карбон М.А., Дункан Л.Х. и др. (2015). «Геномная основа отсроченного старения у Drosophila melanogaster» . ПЛОС ОДИН . 10 (9): e0138569. Бибкод : 2015PLoSO..1038569C . дои : 10.1371/journal.pone.0138569 . ПМЦ 4574564 . ПМИД 26378456 .
- ^ Кэссиди Д., Эпини Д.Г., Саламе С., Чжоу Л.Т., Саломон Р.Н., Ширмер А.Е. и др. (ноябрь 2019 г.). «Доказательства преждевременного старения на модели синдрома Вернера у дрозофилы» . Экспериментальная геронтология . 127 : 110733. doi : 10.1016/j.exger.2019.110733 . ПМЦ 6935377 . ПМИД 31518666 .
- ^ Прюдоммо С., Пруст Дж. УФ-облучение клеток тополя эмбрионов Drosophila melanogaster. V. Исследование мейотической рекомбинации у самок с хромосомами разного строения. Мутат Рес. 23 апреля 1974 г. (1): 63-6. ПМИД 4209047
- ^ Шеве М.Дж., Сузуки Д.Т., Эразмус У. Генетические эффекты митомицина С у Drosophila melanogaster. II. Индуцированная мейотическая рекомбинация. Мутат Рес. Июль 1971 г.; 12 (3): 269–79. дои: 10.1016/0027-5107(71)90015-7. ПМИД 5563942
- ^ Питник С. (1996). «Инвестиции в семенники и стоимость получения длинных сперматозоидов у дрозофилы ». Американский натуралист . 148 : 57–80. дои : 10.1086/285911 . S2CID 83654824 .
- ^ Дагаефф А.С., Пошевиль А., Нобель С., Лойау А., Изабель Г., Данчин Э. (2016). «Копирование партнера дрозофилы коррелирует с атмосферным давлением в ситуации быстрого обучения» . Поведение животных . 121 : 163–174. дои : 10.1016/j.anbehav.2016.08.022 .
- ^ Дукас Р. (2004). «Самцы дрозофил учатся избегать межвидового ухаживания» . Поведенческая экология . 15 (4): 695–698. дои : 10.1093/beheco/arh068 .
- ^ Салим С., Рагглс П.Х., Эбботт В.К., Карни Дж.Е. (2014). «Сексуальный опыт улучшает поведение и успех самцов Drosophila melanogaster» . ПЛОС ОДИН . 9 (5): е96639. Бибкод : 2014PLoSO...996639S . дои : 10.1371/journal.pone.0096639 . ПМК 4013029 . ПМИД 24805129 .
- ↑ Перейти обратно: Перейти обратно: а б фон Хаартман Л. (1951). «Последовательная полигамия». Поведение . 3 (1): 256–273. дои : 10.1163/156853951x00296 .
- ↑ Перейти обратно: Перейти обратно: а б с д и ж г Вартак В.Р., Варма В., Шарма В.К. (февраль 2015 г.). «Влияние полигамии на ритм активности/отдыха самцов плодовых мух Drosophila melanogaster». Die Naturwissenschaften . 102 (1–2): 1252. Бибкод : 2015SciNa.102....3V . дои : 10.1007/s00114-014-1252-5 . ПМИД 25604736 . S2CID 7529509 .
- ↑ Перейти обратно: Перейти обратно: а б Бейтман Эй Джей (декабрь 1948 г.). «Внутриполовой отбор у дрозофилы» . Наследственность . 2 (Часть 3): 349–68. дои : 10.1038/hdy.1948.21 . ПМИД 18103134 .
- ↑ Перейти обратно: Перейти обратно: а б Пирс Б.А. (2004). Генетика: концептуальный подход (2-е изд.). У. Х. Фриман . ISBN 978-0-7167-8881-2 .
- ^ Килби Б.Дж., Макдональд DJ, Ауэрбах С., Собельс Ф.Х., Фогель Э.В. (июнь 1981 г.). «Использование Drosophila melanogaster в тестах на мутагены окружающей среды». Мутационные исследования . 85 (3): 141–6. дои : 10.1016/0165-1161(81)90029-7 . ПМИД 6790982 .
- ↑ Перейти обратно: Перейти обратно: а б Адамс, доктор медицинских наук; Цельникер SE ; Холт Р.А.; Эванс, Калифорния; Гокейн Дж.Д.; Аманатидес ПГ; и др. (март 2000 г.). «Последовательность генома Drosophila melanogaster». Наука . 287 (5461): 2185–95. Бибкод : 2000Sci...287.2185. . CiteSeerX 10.1.1.549.8639 . дои : 10.1126/science.287.5461.2185 . ПМИД 10731132 .
- ↑ Перейти обратно: Перейти обратно: а б с Хотта Ю., Бензер С. (декабрь 1972 г.). «Картирование поведения мозаики дрозофилы». Природа . 240 (5383): 527–35. Бибкод : 1972Natur.240..527H . дои : 10.1038/240527a0 . ПМИД 4568399 . S2CID 4181921 .
- ^ Мейерс С. (2018). «Использование новых технологий секвенирования ДНК для изучения геномных перестроек» . archive.ub.uni-heidelberg.de . дои : 10.11588/heidok.00024506 . Проверено 28 июня 2021 г.
- ^ Аспиазу Н., Фраш М. (июль 1993 г.). «Жестянщик и волынка: два гена гомеобокса, которые определяют судьбу клеток в дорсальной мезодерме дрозофилы» . Гены и развитие . 7 (7Б): 1325–40. дои : 10.1101/gad.7.7b.1325 . ПМИД 8101173 .
- ^ Стерн Д.Л., Франкель Н. (декабрь 2013 г.). «Структура и эволюция цис-регуляторных регионов: история бритого ребенка» . Философские труды Лондонского королевского общества. Серия Б, Биологические науки . 368 : 20130028.doi : (1632 ) 10.1098/rstb.2013.0028 . ПМЦ 3826501 . ПМИД 24218640 .
- ↑ Перейти обратно: Перейти обратно: а б Филлипс А.М., Смарт Р., Штраус Р., Брембс Б., Келли Л.Е. (май 2005 г.). «Загадка черной дрозофилы: молекулярная и поведенческая характеристика мутантного аллеля black1» (PDF) . Джин . 351 : 131–42. дои : 10.1016/j.gene.2005.03.013 . ПМИД 15878647 .
- ↑ Перейти обратно: Перейти обратно: а б «Отчет о генах FlyBase: Dmel\b» . www.flybase.org . Проверено 26 марта 2019 г.
- ^ Шеральд А.Ф. (сентябрь 1981 г.). «Межгенное подавление черной мутации Drosophila melanogaster». Молекулярная и общая генетика . 183 (1): 102–6. дои : 10.1007/bf00270146 . ПМИД 6799739 . S2CID 1210971 .
- ^ Шуп-младший (май 1966 г.). «Развитие пигментных гранул в глазах дикого типа и мутанта Drosophila melanogaster» . Журнал клеточной биологии . 29 (2): 223–49. дои : 10.1083/jcb.29.2.223 . ПМК 2106902 . ПМИД 5961338 .
- ^ «СПРАВОЧНЫЕ СТРАНИЦЫ ДЛЯ УЧИТЕЛЕЙ – ЛАБОРАТОРИЯ ПИГМЕНТОВ FLY EYE PIGMENTS» (PDF) . Архивировано из оригинала (PDF) 26 марта 2019 года . Проверено 26 марта 2019 г.
- ^ Морган Т.Х. (март 1911 г.). «Происхождение мутаций девяти крыльев у дрозофилы». Наука . 33 (848): 496–9. Бибкод : 1911Sci....33..496M . дои : 10.1126/science.33.848.496 . JSTOR 1638587 . ПМИД 17774436 .
- ^ «Отчет о генах FlyBase: Dmel\m» . www.flybase.org . Проверено 26 марта 2019 г.
- ^ Белоусов О.О., Катанаев В.Л., Демидов С.В., Козерецкая И.А. (март–апрель 2013 г.). «Подавление миниатюрного гена не в полной мере воспроизводит миниатюрные фенотипы потери функции в крыле Drosophila melanogaster». Цитология и генетика . 47 (2): 77–81. ПМИД 23745366 .
- ↑ Перейти обратно: Перейти обратно: а б Ким Дж., Су Х., Ким С., Ким К., Ан С., Йим Дж. (сентябрь 2006 г.). «Идентификация и характеристики структурного гена мутантной окраски глаз дрозофилы сепия, кодирующего PDA-синтазу, члена глутатион-S-трансфераз класса омега» . Биохимический журнал . 398 (3): 451–60. дои : 10.1042/BJ20060424 . ПМЦ 1559464 . ПМИД 16712527 .
- ↑ Перейти обратно: Перейти обратно: а б с Грант П., Мага Т., Лошаков А., Сингхал Р., Вали А., Нванкво Дж. и др. (октябрь 2016 г.). «Взгляд на торговлю генами: идентификация четырех мутаций цвета глаз у дрозофилы» . Г3 . 6 (10): 3185–3196. дои : 10.1534/g3.116.032508 . ПМК 5068940 . ПМИД 27558665 .
- ^ Видеррехт Г.Дж., Браун Г.М. (1984). «Очистка и свойства ферментов Drosophila melanogaster, которые катализируют превращение трифосфата дигидронеоптерина в пиримидодиазепиновый предшественник дрозоптеринов» . Ж. Биол. Хим . 259 (22): 14121–7. дои : 10.1016/S0021-9258(18)89865-9 . ПМИД 6438092 .
- ^ «Особенности наследования у Drosophila Melanogaster» . Проверено 26 марта 2019 г.
- ↑ Перейти обратно: Перейти обратно: а б Грин ММ (апрель 1952 г.). «Мутантные изоаллели в локусе Vermilion у Drosophila Melanogaster» . Труды Национальной академии наук Соединенных Штатов Америки . 38 (4): 300–5. Бибкод : 1952ПНАС...38..300Г . дои : 10.1073/pnas.38.4.300 . ПМЦ 1063551 . ПМИД 16589094 .
- ^ Оксенкруг Г.Ф. (январь 2010 г.). «Увеличенная продолжительность жизни мутантов цвета глаз Drosophila melanogaster (белых и ярко-красных) с нарушением образования кинуренина» . Журнал нейронной передачи . 117 (1): 23–26. дои : 10.1007/s00702-009-0341-7 . ПМК 3013506 . ПМИД 19941150 .
- ^ Саймон Э., Фошо С., Зидер А., Тезе Н., Тибо П. (июль 2016 г.). «От рудиментарного к рудиментарному: раскрывшийся ген дрозофилы». Гены развития и эволюция . 226 (4): 297–315. дои : 10.1007/s00427-016-0546-3 . ПМИД 27116603 . S2CID 16651247 .
- ^ Томоясу Ю., Оде Т., Кларк-Хахтел С. (14 марта 2017 г.). «Какие серийные гомологи могут рассказать нам о происхождении крыльев насекомых» . F1000Исследования . 6 : 268. дои : 10.12688/f1000research.10285.1 . ПМК 5357031 . ПМИД 28357056 .
- ^ Уильямс Дж. А., Белл Дж. Б., Кэрролл С.Б. (декабрь 1991 г.). «Контроль над развитием крыльев и жужжальца дрозофилы с помощью продукта ядерного рудиментарного гена» . Гены и развитие . 5 (12Б): 2481–95. дои : 10.1101/gad.5.12b.2481 . ПМИД 1752439 .
- ^ Грин ММ (январь 2010 г.). «2010: Столетие генетики дрозофилы сквозь призму белого гена» . Генетика . 184 (1): 3–7. дои : 10.1534/genetics.109.110015 . ПМК 2815926 . ПМИД 20061564 .
- ^ Феррейро М.Дж., Перес С., Маркесано М., Руис С., Капути А., Агилера П. и др. (2018). «rosophila melanogaster White Mutant w1118 подвергается дегенерации сетчатки» . Границы в неврологии . 11 : 732. дои : 10.3389/fnins.2017.00732 . ПМЦ 5758589 . ПМИД 29354028 .
- ↑ Перейти обратно: Перейти обратно: а б Сяо С., Цю С., Робертсон Р.М. (август 2017 г.). «Белый ген контролирует успех совокупления у Drosophila melanogaster» . Научные отчеты . 7 (1): 7712. Бибкод : 2017NatSR...7.7712X . дои : 10.1038/s41598-017-08155-y . ПМЦ 5550479 . ПМИД 28794482 .
- ^ "Джин:Dmel\y" . Flybase.org . Консорциум FlyBase . Проверено 26 марта 2019 г.
- ^ Витткопп П.Дж., Тру-младший, Кэрролл С.Б. (апрель 2002 г.). «Взаимные функции белков желтого и черного дерева дрозофилы в развитии и эволюции пигментных рисунков». Разработка . 129 (8): 1849–58. дои : 10.1242/dev.129.8.1849 . ПМИД 11934851 .
- ↑ Перейти обратно: Перейти обратно: а б Биссманн Х (ноябрь 1985 г.). «Молекулярный анализ области желтого гена (y) Drosophila melanogaster» . Труды Национальной академии наук Соединенных Штатов Америки . 82 (21): 7369–73. Бибкод : 1985PNAS...82.7369B . дои : 10.1073/pnas.82.21.7369 . ПМЦ 391346 . ПМИД 3933004 .
- ^ «База данных геномов NCBI (Национальный центр биотехнологической информации)» . Проверено 30 ноября 2011 г.
- ^ Халлиган Д.Л., Кейтли П.Д. (июль 2006 г.). «Повсеместные селективные ограничения в геноме дрозофилы, выявленные путем межвидового сравнения всего генома» . Геномные исследования . 16 (7): 875–84. дои : 10.1101/гр.5022906 . ПМЦ 1484454 . ПМИД 16751341 .
- ^ Карвальо AB (декабрь 2002 г.). «Происхождение и эволюция Y-хромосомы дрозофилы». Текущее мнение в области генетики и развития . 12 (6): 664–8. дои : 10.1016/S0959-437X(02)00356-8 . ПМИД 12433579 .
- ^ Габальдон Т., Кунин Е.В. (май 2013 г.). «Функциональные и эволюционные последствия ортологии генов» . Обзоры природы. Генетика . 14 (5). Портфолио природы : 360–366. дои : 10.1038/nrg3456 . ПМЦ 5877793 . ПМИД 23552219 .
- ^ «Основы сравнительного геномного анализа» . Национальный институт исследования генома человека США. Декабрь 2002 года.
- ^ Рейтер Л.Т., Потоцкий Л., Чиен С., Грибсков М., Бир Э. (июнь 2001 г.). «Систематический анализ последовательностей генов, связанных с заболеваниями человека, у Drosophila melanogaster» . Геномные исследования . 11 (6): 1114–25. дои : 10.1101/гр.169101 . ПМК 311089 . ПМИД 11381037 .
- ^ Чиен С., Райтер Л.Т., Бир Э., Грибсков М. (январь 2002 г.). «Гомофила: гены болезней человека, родственные дрозофиле» . Исследования нуклеиновых кислот . 30 (1): 149–51. дои : 10.1093/нар/30.1.149 . ПМК 99119 . ПМИД 11752278 .
- ^ Джайсвал М., Сандовал Х., Чжан К., Баят В., Беллен Х.Дж. (2012). «Изучение механизмов, лежащих в основе нейродегенеративных заболеваний человека у дрозофилы» . Ежегодный обзор генетики . 46 : 371–96. doi : 10.1146/annurev-genet-110711-155456 . ПМЦ 3663445 . ПМИД 22974305 .
- ^ Пик Л (2017). Летающие модели болезней человека. Том 121 текущих тем биологии развития . Академическая пресса. ISBN 978-0-12-802905-3 .
- ^ Бушон Н., Сильверман Н., Черри С. (декабрь 2014 г.). «Иммунитет Drosophila melanogaster - от микробного распознавания до физиологии всего организма» . Обзоры природы. Иммунология . 14 (12): 796–810. дои : 10.1038/nri3763 . ПМК 6190593 . ПМИД 25421701 .
- ^ Каун К.Р., Девинени А.В., Хеберляйн У (июнь 2012 г.). «Drosophila melanogaster как модель изучения наркозависимости» . Генетика человека . 131 (6): 959–75. дои : 10.1007/s00439-012-1146-6 . ПМК 3351628 . ПМИД 22350798 .
- ↑ Перейти обратно: Перейти обратно: а б Вейгманн К., Клаппер Р., Штрассер Т., Рикерт С., Технау Г., Джекле Х. и др. (июнь 2003 г.). «FlyMove — новый взгляд на развитие дрозофилы». Тенденции в генетике . 19 (6): 310–1. дои : 10.1016/S0168-9525(03)00050-7 . ПМИД 12801722 .
- ^ Вест-Эберхард MJ (май 2005 г.). «Пластичность развития и происхождение видовых различий» . Труды Национальной академии наук Соединенных Штатов Америки . 102 (приложение 1): 6543–9. Бибкод : 2005PNAS..102.6543W . дои : 10.1073/pnas.0501844102 . ПМЦ 1131862 . ПМИД 15851679 .
- ↑ Перейти обратно: Перейти обратно: а б с Абрам П.К., Бойвен Дж., Муру Дж., Бродер Дж. (ноябрь 2017 г.). «Поведенческое воздействие температуры на экзотермических животных: объединение тепловой физиологии и поведенческой пластичности». Биологические обзоры Кембриджского философского общества . 92 (4): 1859–1876. дои : 10.1111/brv.12312 . ПМИД 28980433 . S2CID 9099834 .
- ^ Гиберт П., Хьюи Р.Б., Гилкрист Г.В. (январь 2001 г.). «Локомоторные характеристики Drosophila melanogaster: взаимодействие температуры развития и взрослой особи, возраста и географии» . Эволюция; Международный журнал органической эволюции . 55 (1): 205–9. дои : 10.1111/j.0014-3820.2001.tb01286.x . ПМИД 11263741 . S2CID 2991855 .
- ↑ Перейти обратно: Перейти обратно: а б с Замудио КР, Хьюи РБ, Крилл ВД (1995). «Больше не всегда лучше: размер тела, температура развития и родительская температура, а также территориальный успех самцов Drosophila melanogaster». Поведение животных . 49 (3): 671–677. дои : 10.1016/0003-3472(95)80200-2 . ISSN 0003-3472 . S2CID 9124942 .
- ^ Харрисон Дж. Ф., Уотерс Дж. С., Биддульф Т. А., Ковачевич А., Клок С. Дж., Соча Дж. Дж. (апрель 2018 г.). «Пластичность развития и стабильность сетей трахеи, снабжающих летательные мышцы дрозофилы, в ответ на повышение уровня кислорода» . Журнал физиологии насекомых . Границы дыхательной функции: внешние и внутренние ограничения газообмена насекомых. 106 (Часть 3): 189–198. Бибкод : 2018JInsP.106..189H . дои : 10.1016/j.jinsphys.2017.09.006 . ПМИД 28927826 .
- ^ Шиба В., Чандрашекаран М.К., Джоши А., Шарма В.К. (январь 2002 г.). «Пластичность развития ритма двигательной активности Drosophila melanogaster». Журнал физиологии насекомых . 48 (1): 25–32. Бибкод : 2002JInsP..48...25S . дои : 10.1016/S0022-1910(01)00139-1 . ПМИД 12770129 .
- ↑ Перейти обратно: Перейти обратно: а б с д Крилл В.Д., Хьюи Р.Б., Гилкрист Г.В. (июнь 1996 г.). «Влияние температуры внутри и между поколениями на морфологию и физиологию Drosophila Melanogaster». Эволюция; Международный журнал органической эволюции . 50 (3): 1205–1218. дои : 10.2307/2410661 . JSTOR 2410661 . ПМИД 28565273 .
- ↑ Перейти обратно: Перейти обратно: а б Дэвид Дж.Р., Арарипе Л.О., Чакир М., Легут Х., Лемос Б., Петави Г. и др. (июль 2005 г.). «Мужская стерильность при экстремальных температурах: важный, но игнорируемый феномен для понимания климатической адаптации дрозофилы» . Журнал эволюционной биологии . 18 (4): 838–46. дои : 10.1111/j.1420-9101.2005.00914.x . ПМИД 16033555 . S2CID 23847613 .
- ^ Френч В., Фист М., Куропатка Л. (ноябрь 1998 г.). «Размер тела и размер клеток у дрозофилы: реакция развития на температуру». Журнал физиологии насекомых . 44 (11): 1081–1089. Бибкод : 1998JInsP..44.1081F . дои : 10.1016/S0022-1910(98)00061-4 . ПМИД 12770407 .
- ^ Фрейзер М.Р., Харрисон Дж.Ф., Кирктон С.Д., Робертс С.П. (июль 2008 г.). «Выращивание в холоде улучшает способность дрозофилы к холодному полету за счет изменений в морфологии крыльев» . Журнал экспериментальной биологии . 211 (Часть 13): 2116–22. дои : 10.1242/jeb.019422 . ПМИД 18552301 .
- ↑ Перейти обратно: Перейти обратно: а б Слотсбо С., Шу М.Ф., Кристенсен Т.Н., Лешке В., Сёренсен Дж.Г. (сентябрь 2016 г.). «Обратимость развития тепло- и холодо-пластичности асимметрична и имеет долгосрочные последствия для термической толерантности взрослых» . Журнал экспериментальной биологии . 219 (Часть 17): 2726–32. дои : 10.1242/jeb.143750 . ПМИД 27353229 .
- ^ Гилкрист Г.В., Хьюи Р.Б. (январь 2001 г.). «Влияние родительской температуры и температуры развития на термическую зависимость приспособленности Drosophila melanogaster» . Эволюция; Международный журнал органической эволюции . 55 (1): 209–14. дои : 10.1111/j.0014-3820.2001.tb01287.x . ПМИД 11263742 . S2CID 1329035 .
- ^ Остин Си Джей, Меринг Эй Джей (май 2013 г.). «Оптимальный температурный диапазон пластикового вида Drosophila simulans» . Журнал экологии животных . 82 (3): 663–72. Бибкод : 2013JAnEc..82..663A . дои : 10.1111/1365-2656.12041 . ПМИД 23360477 .
- ↑ Перейти обратно: Перейти обратно: а б Ходин Дж., Риддифорд Л.М. (октябрь 2000 г.). «Различные механизмы лежат в основе фенотипической пластичности и межвидовой изменчивости репродуктивных признаков у дрозофилид (Insecta: Diptera)» . Эволюция; Международный журнал органической эволюции . 54 (5): 1638–53. дои : 10.1111/j.0014-3820.2000.tb00708.x . ПМИД 11108591 . S2CID 6875815 .
- ^ Клепсатель П., Гириш Т.Н., Дирксен Х., Галикова М. (май 2019 г.). «Дрозофила максимизируется при оптимальной температуре развития» . Журнал экспериментальной биологии . 222 (Часть 10): jeb202184. дои : 10.1242/jeb.202184 . ПМИД 31064855 .
- ^ Шу М.Ф., Кристенсен Т.Н., Педерсен А., Карлссон Б.Г., Лешке В., Мальмендал А. (февраль 2017 г.). «Метаболическая и функциональная характеристика влияния температуры развития Drosophila melanogaster» . Американский журнал физиологии. Регуляторная, интегративная и сравнительная физиология . 312 (2): Р211–Р222. дои : 10.1152/ajpregu.00268.2016 . ПМЦ 5336569 . ПМИД 27927623 .
- ^ Кохет Ю., Дэвид Дж. (январь 1978 г.). «Контроль репродуктивного потенциала взрослых с помощью преимагинальных тепловых условий: исследование на Drosophila melanogaster». Экология . 36 (3): 295–306. Бибкод : 1978Oecol..36..295C . дои : 10.1007/BF00348055 . ПМИД 28309916 . S2CID 12465060 .
- ^ Rideout EJ, Нарсайя М.С., Гревал СС (декабрь 2015 г.). «Ген-трансформатор определения пола регулирует различия между мужчинами и женщинами в размерах тела дрозофилы» . ПЛОС Генетика . 11 (12): e1005683. дои : 10.1371/journal.pgen.1005683 . ПМК 4692505 . ПМИД 26710087 .
- ^ Гилберт С.Ф. (2000). Биология развития (6-е изд.). Сандерленд (Массачусетс): Sinauer Associates; 2000. ISBN 978-0-87893-243-6 .
- ^ Леметр Б., Хоффманн Дж. (2007). «Защита хозяина Drosophila melanogaster» (PDF) . Ежегодный обзор иммунологии . 25 : 697–743. doi : 10.1146/annurev.immunol.25.022106.141615 . ПМИД 17201680 .
- ^ Троха К., Им Дж. Х., Рева Дж., Лаззаро Б. П., Бушон Н. (февраль 2018 г.). «Сравнительная транскриптомика показывает, что CrebA является новым регулятором толерантности к инфекциям у D. melanogaster» . ПЛОС Патогены . 14 (2): e1006847. дои : 10.1371/journal.ppat.1006847 . ПМЦ 5812652 . ПМИД 29394281 .
- ^ Де Грегорио Э., Спеллман П.Т., Цзоу П., Рубин Г.М., Леметр Б. (июнь 2002 г.). «Пути Toll и Imd являются основными регуляторами иммунного ответа у дрозофилы» . Журнал ЭМБО . 21 (11): 2568–79. дои : 10.1093/emboj/21.11.2568 . ПМК 126042 . ПМИД 12032070 .
- ^ Паредес Х.К., Уэлчман Д.П., Пойдевин М., Леметр Б. (ноябрь 2011 г.). «Негативная регуляция амидазой PGRP формирует антибактериальный ответ дрозофилы и защищает муху от безобидной инфекции» (PDF) . Иммунитет . 35 (5): 770–9. doi : 10.1016/j.immuni.2011.09.018 . ПМИД 22118526 .
- ^ Троха К., Пучон Н. (сентябрь 2019 г.). «Методы изучения врожденного иммунитета Drosophila melanogaster». Междисциплинарные обзоры Wiley. Биология развития . 8 (5): е344. дои : 10.1002/wdev.344 . ПМИД 30993906 . S2CID 119527642 .
- ^ Гилберт Р., Торрес М., Клеменс Р., Хэйтли С., Хосамани Р., Уэйд В., Бхаттачарья С. (февраль 2020 г.). «Модель инфекции Drosophila melanogaster» . npj Микрогравитация . 6 (1): 4. дои : 10.1038/s41526-019-0091-2 . ПМК 7000411 . ПМИД 32047838 .
- ↑ Перейти обратно: Перейти обратно: а б Досталова А., Роммелер С., Пуйдевен М., Леметр Б. (сентябрь 2017 г.). «Тиоэфирсодержащие белки регулируют путь Toll и играют роль в защите дрозофилы от микробных патогенов и паразитоидных ос» . БМК Биология . 15 (1): 79. дои : 10.1186/s12915-017-0408-0 . ПМЦ 5584532 . ПМИД 28874153 .
- ^ Шринивасан Н., Гордон О., Аренс С., Франц А., Деддуш С., Чакраварти П. и др. (ноябрь 2016 г.). «Дрозофила меланогастер» . электронная жизнь . 5 . doi : 10.7554/eLife.19662 . ПМК 5138034 . ПМИД 27871362 .
- ^ Гото А., Яно Т., Терашима Дж., Ивашита С., Осима Ю., Курата С. (май 2010 г.). «Совместная регуляция индукции нового антибактериального листерицина с помощью белка распознавания пептидогликана LE и пути JAK-STAT» . Журнал биологической химии . 285 (21): 15731–8. дои : 10.1074/jbc.M109.082115 . ПМЦ 2871439 . ПМИД 20348097 .
- ^ Ван Л., Кунатидис И., Лигоксигакис П. (январь 2014 г.). «Дрозофила как модель для изучения роли клеток крови в воспалении, врожденном иммунитете и раке» . Границы клеточной и инфекционной микробиологии . 3 : 113. дои : 10.3389/fcimb.2013.00113 . ПМЦ 3885817 . ПМИД 24409421 .
- ^ Нейен С., Бретчер А.Дж., Бинггели О., Леметр Б. (июнь 2014 г.). «Методы изучения иммунитета дрозофилы» (PDF) . Методы . 68 (1): 116–28. дои : 10.1016/j.ymeth.2014.02.023 . ПМИД 24631888 .
- ^ Хашимото Ю., Табути Ю., Сакураи К., Куцуна М., Курокава К., Авасаки Т. и др. (декабрь 2009 г.). «Идентификация липотейхоевой кислоты как лиганда дрейпера при фагоцитозе Staphylococcus aureus гемоцитами дрозофилы» . Журнал иммунологии . 183 (11): 7451–60. doi : 10.4049/jimmunol.0901032 . ПМИД 19890048 .
- ^ Хольц А., Боссингер Б., Штрассер Т., Яннинг В., Клэппер Р. (октябрь 2003 г.). «Два происхождения гемоцитов у дрозофилы» . Разработка . 130 (20): 4955–62. дои : 10.1242/dev.00702 . ПМИД 12930778 .
- ^ Санчес Бош П., Махиджани К., Хербосо Л., Голд К.С., Багинский Р., Вудкок К.Дж. и др. (декабрь 2019 г.). «Взрослые дрозофилы лишены кроветворения, но полагаются на резервуар клеток крови в респираторном эпителии для передачи сигналов инфекции в окружающие ткани» . Развивающая клетка . 51 (6): 787–803.e5. дои : 10.1016/j.devcel.2019.10.017 . ПМЦ 7263735 . ПМИД 31735669 .
- ^ Парви Дж.П., Ю.Ю., Досталова А., Кондо С., Куркан А., Буле П. и др. (июль 2019 г.). «Дрозофила» . электронная жизнь . 8 : e45061. doi : 10.7554/eLife.45061 . ПМК 6667213 . ПМИД 31358113 .
- ^ Стертевант А.Х. (1929). «Бордовый мутантный тип Drosophila simulans: исследование элиминации хромосом и клеточного происхождения». Журнал научной зоологии . 135 : 323–356.
- ^ Ниссани М (май 1975 г.). «Новый поведенческий биоанализ для анализа полового влечения и феромонов у насекомых» . Журнал экспериментальной зоологии . 192 (2): 271–5. Бибкод : 1975JEZ...192..271N . дои : 10.1002/jez.1401920217 . ПМИД 805823 .
- ^ Хан Ф.А. (2011). Основы биотехнологии . ЦРК Пресс. п. 213. ИСБН 978-1-4398-2009-4 .
- ^ «Нобелевская премия по физиологии и медицине 2017 года присуждена совместно Джеффри К. Холлу, Майклу Росбашу и Майклу У. Янгу за открытие молекулярных механизмов, контролирующих циркадный ритм» . Нобелевская премия.org. 2 октября 2017 г. Проверено 5 октября 2017 г.
- ^ Ленерт Б.П., Бейкер А.Е., Годри К., Чианг А.С., Уилсон Р.И. (январь 2013 г.). «Особые роли каналов TRP в слуховой трансдукции и усилении у дрозофилы» . Нейрон . 77 (1): 115–28. дои : 10.1016/j.neuron.2012.11.030 . ПМЦ 3811118 . ПМИД 23312520 .
- ^ Чжан В., Ян З., Ян Л.И., Ян Ю.Н. (август 2013 г.). «Звуковой ответ, опосредованный каналами TRP NOMPC, NANCHUNG и INACTIVE в хордотональных органах личинок дрозофилы» . Труды Национальной академии наук Соединенных Штатов Америки . 110 (33): 13612–7. Бибкод : 2013PNAS..11013612Z . дои : 10.1073/pnas.1312477110 . ПМЦ 3746866 . ПМИД 23898199 .
- ^ "Гомосексуализм включается и выключается у плодовых мушек"
- ^ Дасгупта С., Шихан Т.С., Стивенс К.Ф., Навлаха С. (декабрь 2018 г.). «Нейронная структура данных для обнаружения новизны» . Труды Национальной академии наук Соединенных Штатов Америки . 115 (51): 13093–13098. Бибкод : 2018PNAS..11513093D . дои : 10.1073/pnas.1814448115 . ПМК 6304992 . ПМИД 30509984 .
- ^ Звартс Л., Верстивен М., Каллаертс П. (1 января 2012 г.). «Генетика и нейробиология агрессии у дрозофилы» . Летать . 6 (1): 35–48. дои : 10.4161/fly.19249 . ПМЦ 3365836 . ПМИД 22513455 .
- ^ Дэвис С.М., Томас А.Л., Лю Л., Кэмпбелл И.М., Дирик Х.А. (январь 2018 г.). «Дрозофила использует экран для повреждения крыльев» . Генетика . 208 (1): 273–282. doi : 10.1534/genetics.117.300292 . ПМК 5753862 . ПМИД 29109180 .
- ↑ Перейти обратно: Перейти обратно: а б с Верстевен М., Ванден Брук Л., Гертен Б., Цвартс Л., Декрекер Л., Билен М. и др. (февраль 2017 г.). «Агрессия дрозофилы» . Труды Национальной академии наук Соединенных Штатов Америки . 114 (8): 1958–1963. дои : 10.1073/pnas.1605946114 . ПМЦ 5338383 . ПМИД 28115690 .
- ↑ Перейти обратно: Перейти обратно: а б Сенгупта С., Смит Д.П. (2014). «Как дрозофила обнаруживает летучие феромоны: передача сигналов, схемы и поведение». В Муцинья-Каретта C (ред.). Нейробиология химической связи . Границы в неврологии. CRC Press/Тейлор и Фрэнсис. ISBN 978-1-4665-5341-5 . ПМИД 24830032 . Проверено 30 мая 2019 г.
- ^ Латурни М., Биллетер Дж. К. (август 2016 г.). «Самки Drosophila melanogaster восстанавливают свою привлекательность после спаривания, удаляя мужские антиафродизиакальные феромоны» . Природные коммуникации . 7 (1): 12322. Бибкод : 2016NatCo...712322L . дои : 10.1038/ncomms12322 . ПМЦ 4976142 . ПМИД 27484362 .
- ^ Ван Л., Хан Х., Мерен Дж., Хирой М., Биллетер Дж.К., Миямото Т. и др. (июнь 2011 г.). «Иерархическая хемосенсорная регуляция социальных взаимодействий самцов и самцов у дрозофилы» . Природная неврология . 14 (6): 757–62. дои : 10.1038/nn.2800 . ПМК 3102769 . ПМИД 21516101 .
- ↑ Перейти обратно: Перейти обратно: а б с Лим Р.С., Эййольфсдоттир Э, Шин Э, Перона П., Андерсон DJ (27 августа 2014 г.). «Как еда контролирует агрессию у дрозофилы» . ПЛОС ОДИН . 9 (8): e105626. Бибкод : 2014PLoSO...9j5626L . дои : 10.1371/journal.pone.0105626 . ПМК 4146546 . ПМИД 25162609 .
- ^ Эрион Р., ДиАнджело-младший, Крокер А., Сегал А. (сентябрь 2012 г.). «Взаимодействие между сном и обменом веществ у дрозофилы с измененной передачей сигналов октопамина» . Журнал биологической химии . 287 (39): 32406–14. дои : 10.1074/jbc.M112.360875 . ПМЦ 3463357 . ПМИД 22829591 .
- ↑ Перейти обратно: Перейти обратно: а б с Кайзер М.С., Мэйнваринг Б., Юэ З., Сегал А. (июль 2015 г.). Гриффит LC (ред.). «Депривация сна подавляет агрессию у дрозофилы» . электронная жизнь . 4 : e07643. doi : 10.7554/eLife.07643 . ПМЦ 4515473 . ПМИД 26216041 .
- ^ Харди Р.К., Рагху П. (сентябрь 2001 г.). «Визуальная трансдукция у дрозофилы». Природа . 413 (6852): 186–93. Бибкод : 2001Natur.413..186H . дои : 10.1038/35093002 . ПМИД 11557987 . S2CID 4415605 .
- ↑ Перейти обратно: Перейти обратно: а б с д Шарки Ч.Р., Бланко Дж., Лейбовиц М.М., Пинто-Бенито Д., Уордилл Т.Дж. (октябрь 2020 г.). «Спектральная чувствительность фоторецепторов дрозофилы» . Научные отчеты . 10 (1): 18242. Бибкод : 2020NatSR..1018242S . дои : 10.1038/s41598-020-74742-1 . ПМЦ 7588446 . ПМИД 33106518 . S2CID 215551298 .
Материал был скопирован и адаптирован из этого источника, который доступен по международной лицензии Creative Commons Attribution 4.0 .
- ^ Феуда Р., Гулти М., Задра Н., Гаспаретти Т., Розато Э., Пизани Д. и др. (август 2021 г.). «Филогеномика генов опсинов у двукрылых выявляет события, специфичные для линии, и контрастирующую эволюционную динамику у Anopheles и дрозофилы» . Геномная биология и эволюция . 13 (8): evab170. дои : 10.1093/gbe/evab170 . ПМЦ 8369074 . ПМИД 34270718 .
- ^ Харрис В.А., Старк В.С., Уокер Дж.А. (апрель 1976 г.). «Генетическое вскрытие фоторецепторной системы сложного глаза Drosophila melanogaster» . Журнал физиологии . 256 (2): 415–439. дои : 10.1113/jphysicalol.1976.sp011331 . ПМЦ 1309314 . ПМИД 16992509 . S2CID 45888026 .
- ↑ Перейти обратно: Перейти обратно: а б Острой С.Е., Уилсон М., Пак В.Л. (август 1974 г.). «Родопсин дрозофилы: фотохимия, экстракция и различия в мутанте фототрансдукции norp AP12». Связь с биохимическими и биофизическими исследованиями . 59 (3): 960–966. дои : 10.1016/s0006-291x(74)80073-2 . ПМИД 4213042 .
- ^ Острой ГП (ноябрь 1978 г.). «Характеристики родопсина дрозофилы у мутантов трансдукции зрения дикого типа и norpA» . Журнал общей физиологии . 72 (5): 717–732. дои : 10.1085/jgp.72.5.717 . ПМК 2228556 . ПМИД 105082 . S2CID 5802525 .
- ↑ Перейти обратно: Перейти обратно: а б с д и ж г Сальседо Э., Хубер А., Хенрих С., Чедвелл Л.В., Чоу В.Х., Полсен Р., Бритт С.Г. (декабрь 1999 г.). «Поглощающие синий и зеленый зрительные пигменты дрозофилы: эктопическая экспрессия и физиологическая характеристика родопсинов Rh5 и Rh6, специфичных для фоторецепторных клеток R8» . Журнал неврологии . 19 (24): 10716–10726. doi : 10.1523/jneurosci.19-24-10716.1999 . ПМК 6784940 . ПМИД 10594055 . S2CID 17575850 .
- ^ Киршфельд К., Франческини Н., Минке Б. (сентябрь 1977 г.). «Доказательства наличия сенсибилизирующего пигмента в фоторецепторах мух». Природа . 269 (5627): 386–390. Бибкод : 1977Natur.269..386K . дои : 10.1038/269386a0 . ПМИД 909585 . S2CID 28890008 .
- ^ Минке Б., Киршфельд К. (май 1979 г.). «Вклад сенсибилизирующего пигмента в спектры фоточувствительности родопсина и метародопсина мух» . Журнал общей физиологии . 73 (5): 517–540. дои : 10.1085/jgp.73.5.517 . ПМК 2215190 . ПМИД 458418 . S2CID 12451748 .
- ↑ Перейти обратно: Перейти обратно: а б Киршфельд К., Фейлер Р., Франческини Н. (1978). «Фотостабильный пигмент в рабдомере фоторецепторов № 7 мух». Журнал сравнительной физиологии А. 125 (3): 275–284. дои : 10.1007/BF00656606 . S2CID 40233531 .
- ^ Киршфельд К., Франческини Н. (июнь 1977 г.). «Фотостабильные пигменты в мембране фоторецепторов и их возможная роль». Биофизика структуры и механизма . 3 (2): 191–194. дои : 10.1007/BF00535818 . ПМИД 890056 . S2CID 5846094 .
- ^ Цукер К.С., Монтелл К., Джонс К., Лаверти Т., Рубин Г.М. (май 1987 г.). «Ген родопсина, экспрессируемый в фоторецепторной клетке R7 глаза дрозофилы: гомологии с другими молекулами, передающими сигнал» . Журнал неврологии . 7 (5): 1550–1557. doi : 10.1523/jneurosci.07-05-01550.1987 . ПМК 6568820 . ПМИД 2437266 . S2CID 1490332 .
- ↑ Перейти обратно: Перейти обратно: а б с Чоу В.Х., Холл К.Дж., Уилсон Д.Б., Уайдман К.Л., Таунсон С.М., Чедвелл Л.В., Бритт С.Г. (декабрь 1996 г.). «Идентификация нового опсина дрозофилы выявила специфическое паттернирование фоторецепторных клеток R7 и R8» . Нейрон . 17 (6): 1101–1115. дои : 10.1016/s0896-6273(00)80243-3 . ПМИД 8982159 . S2CID 18294965 .
- ↑ Перейти обратно: Перейти обратно: а б с д Фейлер Р., Бьорнсон Р., Киршфельд К., Мисмер Д., Рубин Г.М., Смит Д.П. и др. (октябрь 1992 г.). «Эктопическая экспрессия ультрафиолетовых родопсинов в синих фоторецепторных клетках дрозофилы: зрительная физиология и фотохимия трансгенных животных» . Журнал неврологии . 12 (10): 3862–3868. doi : 10.1523/jneurosci.12-10-03862.1992 . ПМК 6575971 . ПМИД 1403087 .
- ^ Монтелл С., Джонс К., Цукер С., Рубин Дж. (май 1987 г.). «Второй ген опсина, экспрессируемый в чувствительных к ультрафиолету фоторецепторных клетках R7 Drosophila melanogaster» . Журнал неврологии . 7 (5): 1558–1566. doi : 10.1523/JNEUROSCI.07-05-01558.1987 . ПМК 6568825 . ПМИД 2952772 . S2CID 17003459 .
- ^ Хубер А., Шульц С., Бентроп Дж., Гроелл С., Вольфрум Ю., Полсен Р. (апрель 1997 г.). «Молекулярное клонирование родопсина Rh6 дрозофилы: зрительный пигмент подмножества фоторецепторных клеток R8». Письма ФЭБС . 406 (1–2): 6–10. дои : 10.1016/s0014-5793(97)00210-x . ПМИД 9109375 . S2CID 18368117 .
- ^ Поллок Дж. А., Бензер С. (июнь 1988 г.). «Локализация транскрипта четырех генов опсина в трех зрительных органах дрозофилы; RH2 специфичен для глазка». Природа . 333 (6175): 779–782. Бибкод : 1988Natur.333..779P . дои : 10.1038/333779a0 . ПМИД 2968518 . S2CID 4303934 .
- ↑ Перейти обратно: Перейти обратно: а б Фейлер Р., Харрис В.А., Киршфельд К., Верхан С., Цукер К.С. (июнь 1988 г.). «Направленная неправильная экспрессия гена опсина дрозофилы приводит к изменению зрительной функции». Природа . 333 (6175): 737–741. Бибкод : 1988Natur.333..737F . дои : 10.1038/333737a0 . ПМИД 2455230 . S2CID 4248264 .
- ^ Сакаи К., Цуцуи К., Ямасита Т., Ивабе Н., Такахаши К., Вада А., Шичида Ю. (август 2017 г.). «Родопсин Rh7 дрозофилы melanogaster представляет собой датчик УФ-видимого света с чрезвычайно широким спектром поглощения» . Научные отчеты . 7 (1): 7349. Бибкод : 2017НатСР...7.7349С . дои : 10.1038/s41598-017-07461-9 . ПМЦ 5544684 . ПМИД 28779161 . S2CID 3276084 .
- ^ Ни Дж.Д., Байк Л.С., Холмс Т.К., Монтелл С. (май 2017 г.). «Родопсин в мозге участвует в циркадном фотоувлечении у дрозофилы» . Природа . 545 (7654): 340–344. Бибкод : 2017Natur.545..340N . дои : 10.1038/nature22325 . ПМЦ 5476302 . ПМИД 28489826 . S2CID 4468254 .
- ^ Фогт К. (1 февраля 1984 г.). «Хромофор зрительного пигмента некоторых отрядов насекомых» . Журнал естественных исследований C. 39 (1–2): 196–197. дои : 10.1515/znc-1984-1-236 . S2CID 88980658 .
- ^ Фогт К., Киршфельд К. (апрель 1984 г.). «Химическая идентичность хромофоров зрительного пигмента мух». Naturwissenschaften . 71 (4): 211–213. Бибкод : 1984NW.....71..211В . дои : 10.1007/BF00490436 . S2CID 24205801 .
- ^ Гюманн М., Портер М.Л., Бок М.Дж. (август 2022 г.). «Глюопсины: опсины без лизина, связывающего сетчатку» . Клетки . 11 (15): 2441. doi : 10.3390/cells11152441 . ПМЦ 9368030 . ПМИД 35954284 .
- ^ Катана Р., Гуан С., Занини Д., Ларсен М.Е., Хиральдо Д., Гертен Б.Р. и др. (сентябрь 2019 г.). «Независимая от хромофора роль апопротеинов опсина в механорецепторах дрозофилы» . Современная биология . 29 (17): 2961–2969.е4. Бибкод : 2019CBio...29E2961K . дои : 10.1016/j.cub.2019.07.036 . ПМИД 31447373 . S2CID 201420079 .
- ↑ Перейти обратно: Перейти обратно: а б Люнг Нью-Йорк, Тхакур Д.П., Гурав А.С., Ким Ш., Ди Пицио А., Нив М.Ю., Монтелл С. (апрель 2020 г.). «Функции опсинов во вкусе дрозофилы» . Современная биология . 30 (8): 1367–1379.е6. Бибкод : 2020CBio...30E1367L . дои : 10.1016/j.cub.2020.01.068 . ПМЦ 7252503 . ПМИД 32243853 .
- ^ Кумбаласири Т., Роллаг, доктор медицинских наук, Изольди М.К., Каструччи А.М., Провенсио I (март 2007 г.). «Меланопсин запускает высвобождение внутренних запасов кальция в ответ на свет». Фотохимия и фотобиология . 83 (2): 273–279. дои : 10.1562/2006-07-11-RA-964 . ПМИД 16961436 . S2CID 23060331 .
- ↑ Перейти обратно: Перейти обратно: а б Рагху П., Колли Н.Дж., Вебель Р., Джеймс Т., Хасан Г., Данин М. и др. (май 2000 г.). «Нормальная фототрансдукция в фоторецепторах дрозофилы, лишенных гена рецептора InsP (3)». Молекулярная и клеточная нейронауки . 15 (5): 429–45. дои : 10.1006/mcne.2000.0846 . ПМИД 10833300 . S2CID 23861204 .
- ^ Харди Р.К., Юусола М. (октябрь 2015 г.). «Фототрансдукция у дрозофилы» . Современное мнение в нейробиологии . 34 : 37–45. дои : 10.1016/j.conb.2015.01.008 . ПМИД 25638280 . S2CID 140206989 .
- ^ Ван Т., Сюй Х., Обервинклер Дж., Гу Ю., Харди Р.К., Монтелл С. (февраль 2005 г.). «Функции световой активации, адаптации и выживания клеток обменника Na+/Ca2+ CalX» . Нейрон . 45 (3): 367–78. дои : 10.1016/j.neuron.2004.12.046 . ПМИД 15694324 .
- ^ Рейн К., Цёклер М., Мадер М.Т., Грюбель К., Гейзенберг М. (февраль 2002 г.). «Стандартный мозг дрозофилы» . Современная биология . 12 (3): 227–31. Бибкод : 2002CBio...12..227R . дои : 10.1016/S0960-9822(02)00656-5 . ПМИД 11839276 . S2CID 15785406 .
- ^ Докинз Р., Докинз М. (1976). «Иерархическая организация и облегчение позы: правила ухода за мухами». Поведение животных . 24 (4): 739–755. дои : 10.1016/S0003-3472(76)80003-6 . S2CID 53186674 .
- ↑ Перейти обратно: Перейти обратно: а б с Дэвис WJ (1979). «Поведенческие иерархии». Тенденции в нейронауках . 2 (2): 5–7. дои : 10.1016/0166-2236(79)90003-1 . S2CID 53180462 .
- ↑ Перейти обратно: Перейти обратно: а б Сидс А.М., Равбар П., Чунг П., Хэмпель С., Мидгли Ф.М., Менш Б.Д., Симпсон Дж.Х. (август 2014 г.). «Иерархия подавления среди конкурирующих двигательных программ стимулирует последовательный уход у дрозофилы» . электронная жизнь . 3 : e02951. doi : 10.7554/eLife.02951 . ПМЦ 4136539 . ПМИД 25139955 .
- ^ Матис А., Мамиданна П., Кюри К.М., Абе Т., Мурти В.Н., Матис М.В., Бетге М. (сентябрь 2018 г.). «DeepLabCut: безмаркерная оценка позы определенных пользователем частей тела с помощью глубокого обучения». Природная неврология . 21 (9): 1281–1289. дои : 10.1038/s41593-018-0209-y . ПМИД 30127430 . S2CID 4748395 .
- ^ Штраус Р., Гейзенберг М. (август 1990 г.). «Координация ног при прямолинейной ходьбе и поворотах у Drosophila melanogaster». Журнал сравнительной физиологии А. 167 (3): 403–12. дои : 10.1007/BF00192575 . ПМИД 2121965 . S2CID 12965869 .
- ^ ДеАнджелис Б.Д., Заватоне-Вет Дж.А., Кларк Д.А. (июнь 2019 г.). «Дрозофила» . электронная жизнь . 8 . doi : 10.7554/eLife.46409 . ПМК 6598772 . ПМИД 31250807 .
- ^ Возница А., Бокемюль Т., Дюбберт М., Шольц Х., Бюшгес А. (февраль 2013 г.). «Координация между ногами при контроле скорости ходьбы у дрозофилы» . Журнал экспериментальной биологии . 216 (Часть 3): 480–91. дои : 10.1242/jeb.078139 . ПМИД 23038731 .
- ^ Мендес К.С., Бартос И., Акай Т., Марка С., Манн Р.С. (январь 2013 г.). «Количественная оценка параметров походки свободно идущего дикого типа и сенсорной депривации Drosophila melanogaster» . электронная жизнь . 2 : е00231. doi : 10.7554/eLife.00231 . ПМЦ 3545443 . ПМИД 23326642 .
- ^ Щецинский Н.С., Бокемюль Т., Чокли А.С., Бюшгес А. (ноябрь 2018 г.). «Дрозофила» . Журнал экспериментальной биологии . 221 (Часть 22): jeb189142. дои : 10.1242/jeb.189142 . ПМИД 30274987 .
- ^ Хупер С.Л. (май 2012 г.). «Размер тела и нейронный контроль движения» . Современная биология . 22 (9): Р318-22. Бибкод : 2012CBio...22.R318H . дои : 10.1016/j.cub.2012.02.048 . ПМИД 22575473 .
- ↑ Перейти обратно: Перейти обратно: а б с Фрай С.Н., Саяман Р., Дикинсон М.Х. (апрель 2003 г.). «Аэродинамика маневров свободного полета дрозофилы» (PDF) . Наука . 300 (5618): 495–8. Бибкод : 2003Sci...300..495F . дои : 10.1126/science.1081944 . ПМИД 12702878 . S2CID 40952385 . Архивировано из оригинала (PDF) 24 сентября 2015 г.
- ^ Хессельберг Т., Леманн Ф.О. (декабрь 2007 г.). «Поведение при повороте зависит от фрикционного демпфирования у плодовой мухи дрозофилы» . Журнал экспериментальной биологии . 210 (Часть 24): 4319–34. дои : 10.1242/jeb.010389 . ПМИД 18055621 .
- ^ Чианг А.С., Линь С.И., Чуанг С.К., Чанг Х.М., Се С.Х., Йе С.В. и др. (январь 2011 г.). «Трехмерная реконструкция проводящих сетей всего мозга у дрозофилы с разрешением одной клетки» . Современная биология . 21 (1): 1–11. Бибкод : 2011CBio...21....1C . дои : 10.1016/j.cub.2010.11.056 . ПМИД 21129968 . S2CID 17155338 .
- ^ «FlyCircuit — база данных нейронов головного мозга дрозофилы» . Проверено 30 августа 2013 г.
- ^ Майнерцхаген И.А., О'Нил С.Д. (март 1991 г.). «Синаптическая организация столбчатых элементов в пластинке дикого типа Drosophila melanogaster». Журнал сравнительной неврологии . 305 (2): 232–63. дои : 10.1002/cne.903050206 . ПМИД 1902848 . S2CID 35301798 .
- ^ Ривера-Альба М., Виталадевуни С.Н., Мищенко Ю., Мищенко Ю., Лу З., Такемура С.Ю. и др. (декабрь 2011 г.). «Экономия проводки и исключение объема определяют расположение нейронов в мозгу дрозофилы» . Современная биология . 21 (23): 2000–5. Бибкод : 2011CBio...21.2000R . дои : 10.1016/j.cub.2011.10.022 . ПМЦ 3244492 . ПМИД 22119527 .
- ^ Такемура С.Ю., Бхариоке А., Лу З., Нерн А., Виталадевуни С., Ривлин П.К. и др. (август 2013 г.). «Схема визуального обнаружения движения, предложенная коннектомикой дрозофилы» . Природа . 500 (7461): 175–81. Бибкод : 2013Natur.500..175T . дои : 10.1038/nature12450 . ПМЦ 3799980 . ПМИД 23925240 .
- ^ Такемура С.Ю., Асо Ю., Хиге Т., Вонг А., Лу З., Сюй К.С. и др. (июль 2017 г.). «Мозг дрозофилы» . электронная жизнь . 6 : е26975. дои : 10.7554/eLife.26975 . ПМК 5550281 . ПМИД 28718765 .
- ^ «Весь мозг плодовой мухи, полученный с помощью электронной микроскопии» . Журнал Ученый . Проверено 15 июля 2018 г.
- ^ Чжэн З., Лауритцен Дж.С., Перлман Э., Робинсон К.Г., Николс М., Милки Д. и др. (июль 2018 г.). «Полный объем электронной микроскопии мозга взрослой Drosophila melanogaster» . Клетка . 174 (3): 730–743.e22. bioRxiv 10.1101/140905 . дои : 10.1016/j.cell.2018.06.019 . ПМК 6063995 . ПМИД 30033368 .
- ^ Сюй К.С., Янушевски М., Лу З., Такемура С.Ю., Хейворт К., Хуан Г., Шиномия К., Майтин-Шепард Дж., Акерман Д., Берг С., Блейкли Т. и др. (2020). «Коннектом центрального мозга взрослой дрозофилы ». биоRxiv . Лаборатория Колд-Спринг-Харбор: 21.01.2020.911859. дои : 10.1101/2020.01.21.911859 . S2CID 213140797 .
- ^ «Инструменты анализа для коннектомики» . ХХМИ.
- ^ Фелпс, Джаспер С.; Хильдебранд, Дэвид Грант Колберн; Грэм, Бретт Дж.; Куан, Аарон Т.; Томас, Логан А.; Нгуен, Три М.; Буманн, Джулия; Азеведо, Энтони В.; Сустар, Энн; Агравал, Света; Лю, Мингуань; Шэнни, Брендан Л.; Функе, Ян; Тутхилл, Джон К.; Ли, Вэй-Чунг Аллен (2 февраля 2021 г.). «Реконструкция цепей управления моторикой взрослой дрозофилы с помощью автоматизированной просвечивающей электронной микроскопии» . Клетка . 184 (3): 759–774.e18. дои : 10.1016/j.cell.2020.12.013 . ПМЦ 8312698 . ПМИД 33400916 .
- ^ Шеффер Л.К., Сюй К.С., Янушевски М., Лу З., Такемура С.Ю., Хейворт К.Дж., Хуанг Г., Шиномия К., Мейтлин-Шепард Дж., Берг С., Клементс Дж. и др. (2020). «Коннектом и анализ центрального мозга взрослой дрозофилы » . биоRxiv . 9 . Колд-Спринг-Харбор. дои : 10.1101/2020.04.07.030213 . ПМЦ 7546738 . ПМИД 32880371 . S2CID 215790785 .
- ^ Попович, Сергей; Макрина, Томас; Кемниц, Нико; Кастро, Мануэль; Нехоран, Барак; Цзя, Чжэнь; Бэ, Дж. Александр; Митчелл, Эрик; Му, Шан; Траутман, Эрик Т.; Заальфельд, Стефан; Ли, Кай; Сын, Себастьян (27 марта 2022 г.). «Петамасштабный конвейер для точного выравнивания изображений, полученных при серийной секционной электронной микроскопии». bioRxiv 10.1101/2022.03.25.485816 .
- ^ Виндинг, Майкл; Педиго, Бенджамин Д.; Барнс, Кристофер Л.; Патсолич, Хизер Г.; Парк, Янгсер; Казимирс, Том; Фушики, Акира; Андраде, Ингрид В.; Хандельвал, Авинаш; Вальдес-Алеман, Хавьер; Ли, Фэн; Рэндел, Надин; Барсотти, Элизабет; Коррейя, Ана; Феттер, Ричард Д. (10 марта 2023 г.). «Коннектом мозга насекомого» . Наука . 379 (6636): eadd9330. дои : 10.1126/science.add9330 . ПМК 7614541 . ПМИД 36893230 .
- ^ «Невредные виды» . Здоровье растений Австралии . Проверено 19 сентября 2017 г.
- ^ МакИви С. (5 февраля 2014 г.). «Фруктовые мушки: случай ошибочной идентификации» . Австралийский музей . Проверено 19 сентября 2017 г.
Дальнейшее чтение [ править ]
- Колер Р.Э. (1994). Повелители мух: генетика дрозофилы и экспериментальная жизнь . Чикаго: Издательство Чикагского университета. ISBN 978-0-226-45063-6 .
- Гилберт С.Ф. (2000). Биология развития (6-е изд.). Сандерленд (Массачусетс): Sinauer Associates; 2000. ISBN 978-0-87893-243-6 .
- Перримон Н., Бонини Н.М., Диллон П. (март 2016 г.). «Плодовые мушки на передовой: поступательное воздействие дрозофилы» . Модели и механизмы заболеваний . 9 (3): 229–31. дои : 10.1242/dmm.024810 . ПМЦ 4833334 . ПМИД 26935101 .
- Хендерсон М. (8 апреля 2010 г.). «Ссора из-за плодовой мушки Drosophila melanogaster назвала учёных клопов» . Таймс . Австралиец . Проверено 19 сентября 2017 г.
Внешние ссылки [ править ]


- «Быстрое и простое знакомство с Drosophila melanogaster » . Виртуальная библиотека дрозофилы .
- «Ресурсный центр геномики дрозофилы» - собирает, хранит и распространяет дрозофилы . клоны ДНК и клеточные линии
- «Блумингтонский центр запасов дрозофил» - собирает, хранит и распространяет штаммы Drosophila melanogaster для исследований.
- «FlyBase — база данных генов и геномов дрозофилы» .
- «Программа просмотра карт NCBI – Drosophila melanogaster » .
- «Виртуальная библиотека дрозофилы» .
- Беркли «Проект генома дрозофилы » .
- «ФлайМов» . – видеоресурсы по дрозофилы развитию
- « Номенклатура дрозофил — наименование генов» . Архивировано из оригинала 8 октября 2011 года.
- Посмотреть геном Fruitfly на Ensembl
- Просмотрите сборку генома dm6 в браузере генома UCSC .
- Manchester Fly Facility - для публики. Архивировано 13 мая 2015 г. в Wayback Machine . Манчестерского университета
- Веб-сайт droso4schools со школьными ресурсами о дрозофиле.
- Часть 1 обучающего видеоролика «Маленькая муха: БОЛЬШОЕ воздействие», объясняющая историю и значение модельного организма дрозофилы .
- Часть 2 образовательных видеороликов «Маленькая муха: БОЛЬШОЕ воздействие», объясняющая, как проводятся исследования дрозофилы .
- «Внутри летающей лаборатории» — трансляция WGBH и PBS в серии программ «Любопытно» , январь 2008 г.
- «Как муха обнаруживает яд». Архивировано 13 января 2013 г. на archive.today. В статьеWhyFiles.org описывается, как плодовая мушка пробует на вкус химическое вещество, убивающее личинок, в пище.