RNA interference

RNA interference (RNAi) is a biological process in which RNA molecules are involved in sequence-specific suppression of gene expression by double-stranded RNA, through translational or transcriptional repression. Historically, RNAi was known by other names, including co-suppression, post-transcriptional gene silencing (PTGS), and quelling. The detailed study of each of these seemingly different processes elucidated that the identity of these phenomena were all actually RNAi. Andrew Fire and Craig C. Mello shared the 2006 Nobel Prize in Physiology or Medicine for their work on RNAi in the nematode worm Caenorhabditis elegans, which they published in 1998. Since the discovery of RNAi and its regulatory potentials, it has become evident that RNAi has immense potential in suppression of desired genes. RNAi is now known as precise, efficient, stable and better than antisense therapy for gene suppression.[1] Antisense RNA produced intracellularly by an expression vector may be developed and find utility as novel therapeutic agents.[2]
Two types of small ribonucleic acid (RNA) molecules, microRNA (miRNA) and small interfering RNA (siRNA), are central to components to the RNAi pathway. Once mRNA is degraded, post-transcriptional silencing occurs as protein translation is prevented. Transcription can be inhibited via the pre-transcriptional silencing mechanism of RNAi, through which an enzyme complex catalyzes DNA methylation at genomic positions complementary to complexed siRNA or miRNA. RNAi has an important role in defending cells against parasitic nucleotide sequences (e.g., viruses or transposons) and also influences development of organisms.
The RNAi pathway is a naturally occurring process found in many eukaryotes and animal cells. It is initiated by the enzyme Dicer, which cleaves long double-stranded RNA (dsRNA) molecules into short double-stranded fragments of approximately 21 to 23 nucleotide siRNAs. Each siRNA is unwound into two single-stranded RNAs (ssRNAs), the passenger (sense) strand and the guide (antisense) strand. The passenger strand is then cleaved by the protein Argonaute 2 (Ago2). The passenger strand is degraded and the guide strand is incorporated into the RNA-induced silencing complex (RISC). The RISC assembly then binds and degrades the target mRNA. Specifically, this is accomplished when the guide strand pairs with a complementary sequence in a mRNA molecule and induces cleavage by Ago2, a catalytic component of the RISC. In some organisms, this process spreads systemically, despite the initially limited molar concentrations of siRNA.[3]
RNAi is a valuable research tool, both in cell culture and in living organisms, because synthetic dsRNA introduced into cells can selectively and robustly induce suppression of specific genes of interest. RNAi may be used for large-scale screens that systematically shut down each gene (and the subsequent proteins it codes for) in the cell, which can help to identify the components necessary for a particular cellular process or an event such as cell division. The pathway is also used as a practical tool for food, medicine and insecticides.[4]
Cellular mechanism
[edit]
RNAi is an RNA-dependent gene silencing process that is controlled by RISC and is initiated by short double-stranded RNA molecules in a cell's cytoplasm, where they interact with the catalytic RISC component Argonaute.[6] When the dsRNA is exogenous (coming from infection by a virus with an RNA genome or laboratory manipulations), the RNA is imported directly into the cytoplasm and cleaved to short fragments by Dicer. The initiating dsRNA can also be endogenous (originating in the cell), as in pre-microRNAs expressed from RNA-coding genes in the genome. The primary transcripts from such genes are first processed to form the characteristic stem-loop structure of pre-miRNA in the nucleus, then exported to the cytoplasm. Thus, the two dsRNA pathways, exogenous and endogenous, converge at the RISC.[7]
Exogenous dsRNA initiates RNAi by activating the ribonuclease protein Dicer,[8] which binds and cleaves dsRNAs in plants, or short hairpin RNAs (shRNAs) in humans, to produce double-stranded fragments of 20–25 base pairs with a 2-nucleotide overhang at the 3′ end.[9] Bioinformatics studies on the genomes of multiple organisms suggest this length maximizes target-gene specificity and minimizes non-specific effects.[10] These short double-stranded fragments are called siRNAs. These siRNAs are then separated into single strands and integrated into an active RISC, by RISC-Loading Complex (RLC). RLC includes Dicer-2 and R2D2, and is crucial to unite Ago2 and RISC.[11] TATA-binding protein-associated factor 11 (TAF11) assembles the RLC by facilitating Dcr-2-R2D2 tetramerization, which increases the binding affinity to siRNA by 10-fold. Association with TAF11 would convert the R2-D2-Initiator (RDI) complex into the RLC.[12] R2D2 carries tandem double-stranded RNA-binding domains to recognize the thermodynamically stable terminus of siRNA duplexes, whereas Dicer-2 the other less stable extremity. Loading is asymmetric: the MID domain of Ago2 recognizes the thermodynamically stable end of the siRNA. Therefore, the "passenger" (sense) strand whose 5′ end is discarded by MID is ejected, while the saved "guide" (antisense) strand cooperates with AGO to form the RISC.[11]
After integration into the RISC, siRNAs base-pair to their target mRNA and cleave it, thereby preventing it from being used as a translation template.[13] Differently from siRNA, a miRNA-loaded RISC complex scans cytoplasmic mRNAs for potential complementarity. Instead of destructive cleavage (by Ago2), miRNAs rather target the 3′ untranslated region (UTR) regions of mRNAs where they typically bind with imperfect complementarity, thus blocking the access of ribosomes for translation.[14]
Exogenous dsRNA is detected and bound by an effector protein, known as RDE-4 in C. elegans and R2D2 in Drosophila, that stimulates Dicer activity.[15] The mechanism producing this length specificity is unknown and this protein only binds long dsRNAs.[15]
In C. elegans this initiation response is amplified through the synthesis of a population of 'secondary' siRNAs during which the Dicer-produced initiating or 'primary' siRNAs are used as templates.[16] These 'secondary' siRNAs are structurally distinct from Dicer-produced siRNAs and appear to be produced by an RNA-dependent RNA polymerase (RdRP).[17][18]
MicroRNA
[edit]
MicroRNAs (miRNAs) are genomically encoded non-coding RNAs that help regulate gene expression, particularly during development.[19] The phenomenon of RNAi, broadly defined, includes the endogenously induced gene silencing effects of miRNAs as well as silencing triggered by foreign dsRNA. Mature miRNAs are structurally similar to siRNAs produced from exogenous dsRNA, but before reaching maturity, miRNAs must first undergo extensive post-transcriptional modification. A miRNA is expressed from a much longer RNA-coding gene as a primary transcript known as a pri-miRNA which is processed, in the cell nucleus, to a 70-nucleotide stem-loop structure called a pre-miRNA by the microprocessor complex. This complex consists of an RNase III enzyme called Drosha and a dsRNA-binding protein DGCR8. The dsRNA portion of this pre-miRNA is bound and cleaved by Dicer to produce the mature miRNA molecule that can be integrated into the RISC complex; thus, miRNA and siRNA share the same downstream cellular machinery.[20] First, viral encoded miRNA was described in Epstein–Barr virus (EBV).[21] Thereafter, an increasing number of microRNAs have been described in viruses. VIRmiRNA is a comprehensive catalogue covering viral microRNA, their targets and anti-viral miRNAs[22] (see also VIRmiRNA resource: http://crdd.osdd.net/servers/virmirna/).
siRNAs derived from long dsRNA precursors differ from miRNAs in that miRNAs, especially those in animals, typically have incomplete base pairing to a target and inhibit the translation of many different mRNAs with similar sequences. In contrast, siRNAs typically base-pair perfectly and induce mRNA cleavage only in a single, specific target.[23] In Drosophila and C. elegans, miRNA and siRNA are processed by distinct Argonaute proteins and Dicer enzymes.[24][25]
Three prime untranslated regions and microRNAs
[edit]Three prime untranslated regions (3′UTRs) of mRNAs often contain regulatory sequences that post-transcriptionally cause RNAi. Such 3′-UTRs often contain both binding sites for miRNAs as well as for regulatory proteins. By binding to specific sites within the 3′-UTR, miRNAs can decrease gene expression of various mRNAs by either inhibiting translation or directly causing degradation of the transcript. The 3′-UTR also may have silencer regions that bind repressor proteins that inhibit the expression of a mRNA.
The 3′-UTR often contains microRNA response elements (MREs). MREs are sequences to which miRNAs bind. These are prevalent motifs within 3′-UTRs. Among all regulatory motifs within the 3′-UTRs (e.g. including silencer regions), MREs make up about half of the motifs.
As of 2023, the miRBase web site,[26] an archive of miRNA sequences and annotations, listed 28,645 entries in 271 biologic species. Of these, 1,917 miRNAs were in annotated human miRNA loci. miRNAs were predicted to have an average of about four hundred target mRNAs (affecting expression of several hundred genes).[27] Friedman et al.[27] estimate that >45,000 miRNA target sites within human mRNA 3′UTRs are conserved above background levels, and >60% of human protein-coding genes have been under selective pressure to maintain pairing to miRNAs.
Direct experiments show that a single miRNA can reduce the stability of hundreds of unique mRNAs.[28] Other experiments show that a single miRNA may repress the production of hundreds of proteins, but that this repression often is relatively mild (less than 2-fold).[29][30]
The effects of miRNA dysregulation of gene expression seem to be important in cancer.[31] For instance, in gastrointestinal cancers, nine miRNAs have been identified as epigenetically altered and effective in down regulating DNA repair enzymes.[32]
The effects of miRNA dysregulation of gene expression also seem to be important in neuropsychiatric disorders, such as schizophrenia, bipolar disorder, major depression, Parkinson's disease, Alzheimer's disease and autism spectrum disorders.[33][34][35]
RISC activation and catalysis
[edit]Exogenous dsRNA is detected and bound by an effector protein, known as RDE-4 in C. elegans and R2D2 in Drosophila, that stimulates Dicer activity.[15] This protein only binds long dsRNAs, but the mechanism producing this length specificity is unknown.[15] This RNA-binding protein then facilitates the transfer of cleaved siRNAs to the RISC complex.[36]
In C. elegans this initiation response is amplified through the synthesis of a population of 'secondary' siRNAs during which the Dicer-produced initiating or 'primary' siRNAs are used as templates.[16] These 'secondary' siRNAs are structurally distinct from Dicer-produced siRNAs and appear to be produced by an RNA-dependent RNA polymerase (RdRP).[17][18]


The active components of an RNA-induced silencing complex (RISC) are endonucleases called Argonaute proteins, which cleave the target mRNA strand complementary to their bound siRNA.[6] As the fragments produced by Dicer are double-stranded, they could each in theory produce a functional siRNA. However, only one of the two strands, which is known as the guide strand, binds Argonaute and directs gene silencing. The other anti-guide strand or passenger strand is degraded during RISC activation.[37] Although it was first believed that an ATP-dependent helicase separated these two strands,[38] the process proved to be ATP-independent and performed directly by the protein components of RISC.[3][39] However, an in vitro kinetic analysis of RNAi in the presence and absence of ATP showed that ATP may be required to unwind and remove the cleaved mRNA strand from the RISC complex after catalysis.[40] The guide strand tends to be the one whose 5′ end is less stably paired to its complement,[41] but strand selection is unaffected by the direction in which Dicer cleaves the dsRNA before RISC incorporation.[42] Instead, the R2D2 protein may serve as the differentiating factor by binding the more-stable 5′ end of the passenger strand.[43]
The structural basis for binding of RNA to the Argonaute protein was examined by X-ray crystallography of the binding domain of an RNA-bound Argonaute. Here, the phosphorylated 5′ end of the RNA strand enters a conserved basic surface pocket and makes contacts through a divalent cation (an atom with two positive charges) such as magnesium and by aromatic stacking (a process that allows more than one atom to share an electron by passing it back and forth) between the 5′ nucleotide in the siRNA and a conserved tyrosine residue. This site is thought to form a nucleation site for the binding of the siRNA to its mRNA target.[44] Analysis of the inhibitory effect of mismatches in either the 5’ or 3’ end of the guide strand has demonstrated that the 5’ end of the guide strand is likely responsible for matching and binding the target mRNA, while the 3’ end is responsible for physically arranging target mRNA into a cleavage-favorable RISC region.[40]
It is not understood how the activated RISC complex locates complementary mRNAs within the cell. Although the cleavage process has been proposed to be linked to translation, translation of the mRNA target is not essential for RNAi-mediated degradation.[45] Indeed, RNAi may be more effective against mRNA targets that are not translated.[46] Argonaute proteins are localized to specific regions in the cytoplasm called P-bodies (also cytoplasmic bodies or GW bodies), which are regions with high rates of mRNA decay;[47] miRNA activity is also clustered in P-bodies.[48] Disruption of P-bodies decreases the efficiency of RNAi, suggesting that they are a critical site in the RNAi process.[49]
Transcriptional silencing
[edit]
Components of the RNAi pathway are used in many eukaryotes in the maintenance of the organization and structure of their genomes. Modification of histones and associated induction of heterochromatin formation serves to downregulate genes pre-transcriptionally;[51] this process is referred to as RNA-induced transcriptional silencing (RITS), and is carried out by a complex of proteins called the RITS complex. In fission yeast this complex contains Argonaute, a chromodomain protein Chp1, and a protein called Tas3 of unknown function.[52] As a consequence, the induction and spread of heterochromatic regions requires the Argonaute and RdRP proteins.[53] Indeed, deletion of these genes in the fission yeast S. pombe disrupts histone methylation and centromere formation,[54] causing slow or stalled anaphase during cell division.[55] In some cases, similar processes associated with histone modification have been observed to transcriptionally upregulate genes.[56]
The mechanism by which the RITS complex induces heterochromatin formation and organization is not well understood. Most studies have focused on the mating-type region in fission yeast, which may not be representative of activities in other genomic regions/organisms. In maintenance of existing heterochromatin regions, RITS forms a complex with siRNAs complementary to the local genes and stably binds local methylated histones, acting co-transcriptionally to degrade any nascent pre-mRNA transcripts that are initiated by RNA polymerase. The formation of such a heterochromatin region, though not its maintenance, is Dicer-dependent, presumably because Dicer is required to generate the initial complement of siRNAs that target subsequent transcripts.[57] Heterochromatin maintenance has been suggested to function as a self-reinforcing feedback loop, as new siRNAs are formed from the occasional nascent transcripts by RdRP for incorporation into local RITS complexes.[58] The relevance of observations from fission yeast mating-type regions and centromeres to mammals is not clear, as heterochromatin maintenance in mammalian cells may be independent of the components of the RNAi pathway.[59]
Crosstalk with RNA editing
[edit]The type of RNA editing that is most prevalent in higher eukaryotes converts adenosine nucleotides into inosine in dsRNAs via the enzyme adenosine deaminase (ADAR).[60] It was originally proposed in 2000 that the RNAi and A→I RNA editing pathways might compete for a common dsRNA substrate.[61] Some pre-miRNAs do undergo A→I RNA editing[62][63] and this mechanism may regulate the processing and expression of mature miRNAs.[63] Furthermore, at least one mammalian ADAR can sequester siRNAs from RNAi pathway components.[64] Further support for this model comes from studies on ADAR-null C. elegans strains indicating that A→I RNA editing may counteract RNAi silencing of endogenous genes and transgenes.[65]

Variation among organisms
[edit]Organisms vary in their ability to take up foreign dsRNA and use it in the RNAi pathway. The effects of RNAi can be both systemic and heritable in plants and C. elegans, although not in Drosophila or mammals. In plants, RNAi is thought to propagate by the transfer of siRNAs between cells through plasmodesmata (channels in the cell walls that enable communication and transport).[38] Heritability comes from methylation of promoters targeted by RNAi; the new methylation pattern is copied in each new generation of the cell.[67] A broad general distinction between plants and animals lies in the targeting of endogenously produced miRNAs; in plants, miRNAs are usually perfectly or nearly perfectly complementary to their target genes and induce direct mRNA cleavage by RISC, while animals' miRNAs tend to be more divergent in sequence and induce translational repression.[66] This translational effect may be produced by inhibiting the interactions of translation initiation factors with the mRNA's polyadenine tail.[68]
Some eukaryotic protozoa such as Leishmania major and Trypanosoma cruzi lack the RNAi pathway entirely.[69][70] Most or all of the components are also missing in some fungi, most notably the model organism Saccharomyces cerevisiae.[71] The presence of RNAi in other budding yeast species such as Saccharomyces castellii and Candida albicans, further demonstrates that inducing two RNAi-related proteins from S. castellii facilitates RNAi in S. cerevisiae.[72] That certain ascomycetes and basidiomycetes are missing RNAi pathways indicates that proteins required for RNA silencing have been lost independently from many fungal lineages, possibly due to the evolution of a novel pathway with similar function, or to the lack of selective advantage in certain niches.[73]
Related prokaryotic systems
[edit]Gene expression in prokaryotes is influenced by an RNA-based system similar in some respects to RNAi. Here, RNA-encoding genes control mRNA abundance or translation by producing a complementary RNA that anneals to an mRNA. However these regulatory RNAs are not generally considered to be analogous to miRNAs because the Dicer enzyme is not involved.[74] It has been suggested that CRISPR interference systems in prokaryotes are analogous to eukaryotic RNAi systems, although none of the protein components are orthologous.[75]
Biological functions
[edit]![]() | This section needs to be updated.(May 2020) |
Immunity
[edit]RNAi is a vital part of the immune response to viruses and other foreign genetic material, especially in plants where it may also prevent the self-propagation of transposons.[76] Plants such as Arabidopsis thaliana express multiple Dicer homologs that are specialized to react differently when the plant is exposed to different viruses.[77] Even before the RNAi pathway was fully understood, it was known that induced gene silencing in plants could spread throughout the plant in a systemic effect and could be transferred from stock to scion plants via grafting.[78] This phenomenon has since been recognized as a feature of the plant immune system which allows the entire plant to respond to a virus after an initial localized encounter.[79] In response, many plant viruses have evolved elaborate mechanisms to suppress the RNAi response.[80] These include viral proteins that bind short double-stranded RNA fragments with single-stranded overhang ends, such as those produced by Dicer.[81] Some plant genomes also express endogenous siRNAs in response to infection by specific types of bacteria.[82] These effects may be part of a generalized response to pathogens that downregulates any metabolic process in the host that aids the infection process.[83]
Although animals generally express fewer variants of the Dicer enzyme than plants, RNAi in some animals produces an antiviral response. In both juvenile and adult Drosophila, RNAi is important in antiviral innate immunity and is active against pathogens such as Drosophila X virus.[84][85] A similar role in immunity may operate in C. elegans, as Argonaute proteins are upregulated in response to viruses and worms that overexpress components of the RNAi pathway are resistant to viral infection.[86][87]
The role of RNAi in mammalian innate immunity is poorly understood, and relatively little data is available. However, the existence of viruses that encode genes able to suppress the RNAi response in mammalian cells may be evidence in favour of an RNAi-dependent mammalian immune response,[88][89] although this hypothesis has been challenged as poorly substantiated.[90] Evidence for the existence of a functional antiviral RNAi pathway in mammalian cells has been presented.[91][92]
Other functions for RNAi in mammalian viruses also exist, such as miRNAs expressed by the herpes virus that may act as heterochromatin organization triggers to mediate viral latency.[93]
Downregulation of genes
[edit]Endogenously expressed miRNAs, including both intronic and intergenic miRNAs, are most important in translational repression[66] and in the regulation of development, especially on the timing of morphogenesis and the maintenance of undifferentiated or incompletely differentiated cell types such as stem cells.[94] The role of endogenously expressed miRNA in downregulating gene expression was first described in C. elegans in 1993.[95] In plants this function was discovered when the "JAW microRNA" of Arabidopsis was shown to be involved in the regulation of several genes that control plant shape.[96] In plants, the majority of genes regulated by miRNAs are transcription factors;[97] thus miRNA activity is particularly wide-ranging and regulates entire gene networks during development by modulating the expression of key regulatory genes, including transcription factors as well as F-box proteins.[98] In many organisms, including humans, miRNAs are linked to the formation of tumors and dysregulation of the cell cycle. Here, miRNAs can function as both oncogenes and tumor suppressors.[99]
Evolution
[edit]Based on parsimony-based phylogenetic analysis, the most recent common ancestor of all eukaryotes most likely already possessed an early RNAi pathway; the absence of the pathway in certain eukaryotes is thought to be a derived characteristic.[100] This ancestral RNAi system probably contained at least one Dicer-like protein, one Argonaute, one PIWI protein, and an RNA-dependent RNA polymerase that may also have played other cellular roles. A large-scale comparative genomics study likewise indicates that the eukaryotic crown group already possessed these components, which may then have had closer functional associations with generalized RNA degradation systems such as the exosome.[101] This study also suggests that the RNA-binding Argonaute protein family, which is shared among eukaryotes, most archaea, and at least some bacteria (such as Aquifex aeolicus), is homologous to and originally evolved from components of the translation initiation system.
Applications
[edit]RNAi pathway for gene knockdown
[edit]Gene knockdown is a method used to reduce the expression of an organism’s specific genes. This is accomplished by using the naturally occurring process of RNAi.[6] This gene knockdown technique uses a double-stranded siRNA molecule that is synthesized with a sequence complementary to the gene of interest. The RNAi cascade begins once the Dicer enzyme starts to process siRNA. The end result of the process leads to degradation of mRNA and destroys any instructions needed to build certain proteins. Using this method, researchers are able to decrease (but not completely eliminate) the expression of a targeted gene. Studying the effects of this decrease in expression may show the physiological role or impact of the targeted gene products.[102][103]
Off-Target Effects of Gene Knockdown
[edit]Extensive efforts in computational biology have been directed toward the design of successful dsRNA reagents that maximize gene knockdown but minimize "off-target" effects. Off-target effects arise when an introduced RNA has a base sequence that can pair with and thus reduce the expression of multiple genes. Such problems occur more frequently when the dsRNA contains repetitive sequences. It has been estimated from studying the genomes of humans, C. elegans and S. pombe that about 10% of possible siRNAs have substantial off-target effects.[10] A multitude of software tools have been developed implementing algorithms for the design of general[104][105] mammal-specific,[106] and virus-specific[107] siRNAs that are automatically checked for possible cross-reactivity.
Depending on the organism and experimental system, the exogenous RNA may be a long strand designed to be cleaved by Dicer, or short RNAs designed to serve as siRNA substrates. In most mammalian cells, shorter RNAs are used because long double-stranded RNA molecules induce the mammalian interferon response, a form of innate immunity that reacts nonspecifically to foreign genetic material.[108] Mouse oocytes and cells from early mouse embryos lack this reaction to exogenous dsRNA and are therefore a common model system for studying mammalian gene-knockdown effects.[109] Specialized laboratory techniques have also been developed to improve the utility of RNAi in mammalian systems by avoiding the direct introduction of siRNA, for example, by stable transfection with a plasmid encoding the appropriate sequence from which siRNAs can be transcribed,[110] or by more elaborate lentiviral vector systems allowing the inducible activation or deactivation of transcription, known as conditional RNAi.[111][112]
Medications
[edit]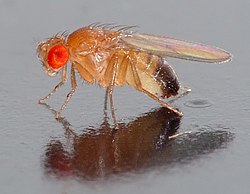

The technique of knocking down genes using RNAi therapeutics has demonstrated success in randomized controlled clinical studies. These medications are a growing class of siRNA-based drugs that decrease the expression of proteins encoded by certain genes. To date, five RNAi medications have been approved by regulatory authorities in the US and Europe: patisiran (2018), givosiran (2019), lumasiran (2020), inclisiran (2020 in Europe with anticipated US approval in 2021), and vutrisiran (2022).[113][114][115][116]
While all of the current regulatory body approved RNAi therapeutics focus on diseases that originate in the liver, additional medications under investigation target a host of disease areas including cardiovascular diseases, bleeding disorders, alcohol use disorders, cystic fibrosis, gout, carcinoma, and eye disorders.
Patisiran is the first double stranded siRNA-based medication approved in 2018 and developed by Alnylam Pharmaceuticals. Patisiran uses the RNAi cascade to suppress the gene that codes for TTR (transthryetin). Mutations in this gene may cause the misfolding of a protein responsible for hereditary ATTR amyloidosis. To achieve therapeutic response, patisiran is encased by a lipid nanoparticle membrane that facilitates crossover into the cytoplasm. Once inside the cell, the siRNA begins processing by the enzyme Dicer. Patisiran is administered by a healthcare professional through an intravenous infusion with dosing based on body weight. Warnings and precautions include risk of infusion-related reactions and reduced vitamin A levels (serum).[117]
In 2019, the FDA and EMA approved givosiran for the treatment of adults with acute hepatic porphyria (AHP).[118] The FDA also granted givosiran a breakthrough therapy designation, priority review designation, and orphan drug designation for the treatment of acute hepatic porphyria (AHP) in November 2019.[119] By 2020, givosiran received EMA approval.[120] Givosiran is an siRNA that breaks down aminolevulinic acid synthase 1 (ALAS1) mRNA in the liver. Breaking down ALAS1 mRNA prevents toxins (responsible for neurovisceral attacks and AHP disease) such as aminolevulinic acid (ALA) and porphobilinogen (PBG) from accumulating.[121][122][123][124] To facilitate entry into the cytoplasm, givosiran uses GalNAc ligands and enters into liver cells. The medication is administered subcutaneously by a healthcare professional with dosing based on body weight. Warnings and precautions include risk of anaphylactic reactions, hepatic toxicity, renal toxicity and injection site reactions.[125]
Lumasiran was approved as a siRNA-based medication in 2020 for use in both the European Union and the United States.[126][127] This medication is used for the treatment of primary hyperoxaluria type 1 (PH1) in pediatric and adult populations. The drug is designed to reduce hepatic oxalate production and urinary oxalate levels through RNAi by targeting hydroxyacid oxidase 1 (HAO1) mRNA for breakdown. Lowering HAO1 enzyme levels reduces the oxidation of glycolate to glyoxylate (which is a substrate for oxalate). Lumasiran is administered subcutaneously by a healthcare professional with dosing based on body weight.[128] Data from randomized controlled clinical trials indicate that the most common adverse reaction that was reported was injection site reactions. These reactions were mild and were present in 38 percent of patients treated with lumasiran.[129]
In 2022, the FDA and EMA approved vutrisiran for the treatment of adults with hereditary transthyretin mediated amyloidosis with polyneuropathy stage 1 or 2.[130][131] Vutrisiran is designed to break down the mRNA that codes for transthyretin.
Other investigational drugs using RNAi that are being developed by pharmaceutical companies such as Arrowhead Pharmaceuticals, Dicerna, Alnylam Pharmaceuticals, Amgen, and Sylentis. These medications cover a variety of targets via RNAi and diseases.
Investigational RNAi therapeutics in development:
Drug | Target | Delivery System | Disease | Phase | Status | Company | Identifier |
---|---|---|---|---|---|---|---|
ALN–VSP02 | KSP and VEGF | LNP | Solid tumours | I | Completed | Alnylam Pharmaceuticals | NCT01158079 |
siRNA–EphA2–DOPC | EphA2 | LNP | Advanced cancers | I | Recruiting | MD Anderson Cancer Center | NCT01591356 |
Atu027 | PKN3 | LNP | Solid tumours | I | Completed | Silence Therapeutics | NCT00938574 |
TKM–080301 | PLK1 | LNP | Cancer | I | Recruiting | Tekmira Pharmaceutical | NCT01262235 |
TKM–100201 | VP24, VP35, Zaire Ebola L-polymerase | LNP | Ebola-virus infection | I | Recruiting | Tekmira Pharmaceutical | NCT01518881 |
ALN–RSV01 | RSV nucleocapsid | Naked siRNA | Respiratory syncytial virus infections | II | Completed | Alnylam Pharmaceuticals | NCT00658086 |
PRO-040201 | ApoB | LNP | Hypercholesterolaemia | I | Terminated | Tekmira Pharmaceutical | NCT00927459 |
ALN–PCS02 | PCSK9 | LNP | Hypercholesterolaemia | I | Completed | Alnylam Pharmaceuticals | NCT01437059 |
ALN–TTR02 | TTR | LNP | Transthyretin-mediated amyloidosis | II | Recruiting | Alnylam Pharmaceuticals | NCT01617967 |
CALAA-01 | RRM2 | Cyclodextrin NP | Solid tumours | I | Active | Calando Pharmaceuticals | NCT00689065 |
TD101 | K6a (N171K mutation) | Naked siRNA | Pachyonychia congenita | I | Completed | Pachyonychia Congenita Project | NCT00716014 |
AGN211745 | VEGFR1 | Naked siRNA | Age-related macular degeneration, choroidal neovascularization | II | Terminated | Allergan | NCT00395057 |
QPI-1007 | CASP2 | Naked siRNA | Optic atrophy, non-arteritic anterior ischaemic optic neuropathy | I | Completed | Quark Pharmaceuticals | NCT01064505 |
I5NP | p53 | Naked siRNA | Kidney injury, acute renal failure | I | Completed | Quark Pharmaceuticals | NCT00554359 |
Delayed graft function, complications of kidney transplant | I, II | Recruiting | Quark Pharmaceuticals | NCT00802347 | |||
PF-655 (PF-04523655) | RTP801 (Proprietary target) | Naked siRNA | Choroidal neovascularization, diabetic retinopathy, diabetic macular oedema | II | Active | Quark Pharmaceuticals | NCT01445899 |
siG12D LODER | KRAS | LODER polymer | Pancreatic cancer | II | Recruiting | Silenseed | NCT01676259 |
Bevasiranib | VEGF | Naked siRNA | Diabetic macular oedema, macular degeneration | II | Completed | Opko Health | NCT00306904 |
SYL1001 | TRPV1 | Naked siRNA | Ocular pain, dry-eye syndrome | I, II | Recruiting | Sylentis | NCT01776658 |
SYL040012 | ADRB2 | Naked siRNA | Ocular hypertension, open-angle glaucoma | II | Recruiting | Sylentis | NCT01739244 |
CEQ508 | CTNNB1 | Escherichia coli-carrying shRNA | Familial adenomatous polyposis | I, II | Recruiting | Marina Biotech | Unknown |
RXi-109 | CTGF | Self-delivering RNAi compound | Cicatrix scar prevention | I | Recruiting | RXi Pharmaceuticals | NCT01780077 |
ALN–TTRsc | TTR | siRNA–GalNAc conjugate | Transthyretin-mediated amyloidosis | I | Recruiting | Alnylam Pharmaceuticals | NCT01814839 |
ARC-520 | Conserved regions of HBV | DPC | HBV | I | Recruiting | Arrowhead Research | NCT01872065 |
Legal categorization and legal issues in a near future
[edit]Currently, both miRNA and SiRNA are currently chemically synthesized and so, are legally categorized inside EU and in USA as "simple" medicinal products. But as bioengineered siRNA (BERAs) are in development, these would be classified as biological medicinal products, at least in EU. The development of the BERAs technology raises the question of the categorization of drugs having the same mechanism of action but being produced chemically or biologically. This lack of consistency should be addressed.[132]
Delivery mechanisms
[edit]To achieve the clinical potential of RNAi, siRNA must be efficiently transported to the cells of target tissues. However, there are various barriers that must be fixed before it can be used clinically. For example, "naked" siRNA is susceptible to several obstacles that reduce its therapeutic efficacy.[133] Additionally, once siRNA has entered the bloodstream, naked RNA can be degraded by serum nucleases and can stimulate the innate immune system.[133] Due to its size and highly polyanionic (containing negative charges at several sites) nature, unmodified siRNA molecules cannot readily enter the cells through the cell membrane. Therefore, artificial or nanoparticle encapsulated siRNA must be used. If siRNA is transferred across the cell membrane, unintended toxicities can occur if therapeutic doses are not optimized, and siRNAs can exhibit off-target effects (e.g. unintended downregulation of genes with partial sequence complementarity).[134] Even after entering the cells, repeated dosing is required since their effects are diluted at each cell division. In response to these potential issues and barriers, two approaches help facilitate siRNA delivery to target cells: lipid nanoparticles and conjugates.[135]
Lipid nanoparticles
[edit]Lipid nanoparticles (LNPs) are based on liposome-like structures that are typically made of an aqueous center surrounded by a lipid shell.[136] A subset of liposomal structures used for delivery drugs to tissues rest in large unilamellar vesicles (LUVs) which may be 100 nm in size. LNP delivery mechanisms have become an increasing source of encasing nucleic acids and may include plasmids, CRISPR and mRNA.[137]
The first approved use of lipid nanoparticles as a drug delivery mechanism began in 2018 with the siRNA drug patisiran, developed by Alnylam Pharmaceuticals. Dicerna Pharmaceuticals, Persomics, Sanofi and Sirna Therapeutics also worked to bring RNAi therapies to market.[138][139]
Other recent applications include two FDA approved COVID-19 vaccines: mRNA-1273, developed by Moderna. and BNT162b, developed by a collaboration between Pfizer and BioNtech.[140] These two vaccines use lipid nanoparticles to deliver antigen mRNA. Encapsulating the mRNA molecule in lipid nanoparticles was a critical breakthrough for producing viable mRNA vaccines, solving a number of key technical barriers in delivering the mRNA molecule into the host cell as distributed through apolipoprotein E (apoE) in the low-density lipoprotein receptor (LDLR). In December 2020, Novartis announced that positive results from phase III efficacy studies deemed inclisiran was a treatment for heterozygous familial hypercholesterolemia (HeFH) and atherosclerotic cardiovascular disease (ASCVD).[141]
Conjugates
[edit]In addition to LNPs, RNAi therapeutics have targeted delivery through siRNA conjugates (e.g., GalNAc, carbohydrates, peptides, aptamers, antibodies).[142] Therapeutics using siRNA conjugates have been developed for rare or genetic diseases such as acute hepatic porphyria (AHP), hemophilia, primary hyperoxaluria (PH) and hereditary ATTR amyloidosis as well as other cardiometabolic diseases such as hypertension and non-alcoholic steatohepatitis (NASH).[143]
Biotechnology
[edit]RNAi has been used for a variety of other applications including food, crops and insecticides. The use of the RNAi pathway has developed numerous products such as foods like Arctic apples, nicotine-free tobacco, decaffeinated coffee, nutrient fortified vegetation and hypoallergenic crops.[144][145][146] The emerging use of RNAi has the potential to develop many other products for future use.
Viral infection
[edit]Antiviral treatment is one of the earliest proposed RNAi-based medical applications, and two different types have been developed. The first type is to target viral RNAs. Many studies have shown that targeting viral RNAs can suppress the replication of numerous viruses, including HIV,[147] HPV,[148] hepatitis A,[149] hepatitis B,[150] influenza virus,[151][152][153][154] respiratory syncytial virus (RSV),[154] SARS coronavirus (SARS-CoV),[154] adenovirus[154] and measles virus.[155] The other strategy is to block the initial viral entries by targeting the host cell genes.[156] For example, suppression of chemokine receptors (CXCR4 and CCR5) on host cells can prevent HIV viral entry.[157]
Cancer
[edit]While traditional chemotherapy can effectively kill cancer cells, lack of specificity for discriminating normal cells and cancer cells in these treatments usually cause severe side effects. Numerous studies have demonstrated that RNAi can provide a more specific approach to inhibit tumor growth by targeting cancer-related genes (i.e., oncogene).[158] It has also been proposed that RNAi can enhance the sensitivity of cancer cells to chemotherapeutic agents, providing a combinatorial therapeutic approach with chemotherapy.[159] Another potential RNAi-based treatment is to inhibit cell invasion and migration.[160]
Compared with chemotherapy or other anti-cancer drugs, there are a lot of advantages of siRNA drug.[161] SiRNA acts on the post-transcriptional stage of gene expression, so it does not modify or change DNA in a deleterious effect.[161] SiRNA can also be used to produce a specific response in a certain type of way, such as by downgrading suppression of gene expression.[161] In a single cancer cell, siRNA can cause dramatic suppression of gene expression with just several copies.[161] This happens by silencing cancer-promoting genes with RNAi, as well as targeting an mRNA sequence.[161]
RNAi drugs treat cancer by silencing certain cancer promoting genes.[161] This is done by complementing the cancer genes with the RNAi, such as keeping the mRNA sequences in accordance with the RNAi drug.[161] Ideally, RNAi is should be injected and/or chemically modified so the RNAi can reach cancer cells more efficiently.[161] RNAi uptake and regulation is controlled by the kidneys.[161]
Neurological diseases
[edit]RNAi strategies also show potential for treating neurodegenerative diseases. Studies in cells and in mouse have shown that specifically targeting Amyloid beta-producing genes (e.g. BACE1 and APP) by RNAi can significantly reduced the amount of Aβ peptide which is correlated with the cause of Alzheimer's disease.[162][163][164] In addition, this silencing-based approaches also provide promising results in treatment of Parkinson's disease and Polyglutamine disease.[165][166][167]
Stimulation of immune response
[edit]The human immune system is divided into two separate branches: the innate immune system and the adaptive immune system.[168] The innate immune system is the first defense against infection and responds to pathogens in a generic fashion.[168] On the other hand, the adaptive immune system, a system that was evolved later than the innate, is composed mainly of highly specialized B and T cells that are trained to react to specific portions of pathogenic molecules.[168]
The challenge between old pathogens and new has helped create a system of guarded cells and particles that are called safe framework.[168] This framework has given humans an army of systems that search out and destroy invader particles, such as pathogens, microscopic organisms, parasites, and infections.[168] The mammalian safe framework has developed to incorporate siRNA as a tool to indicate viral contamination, which has allowed siRNA is create an intense innate immune response.[168]
siRNA is controlled by the innate immune system, which can be divided into the acute inflammatory responses and antiviral responses.[168] The inflammatory response is created with signals from small signaling molecules, or cytokines.[168] These include interleukin-1 (IL-1), interleukin-6 (IL-6), interleukin-12 (IL-12) and tumor necrosis factor α (TNF-α).[168] The innate immune system generates inflammation and antiviral responses, which cause the release pattern recognition receptors (PRRs).[168] These receptors help in labeling which pathogens are viruses, fungi, or bacteria.[168] Moreover, the importance of siRNA and the innate immune system is to include more PRRs to help recognize different RNA structures.[168] This makes it more likely for the siRNA to cause an immunostimulant response in the event of the pathogen.[168]
Food
[edit]RNAi has been used to genetically engineer plants to produce lower levels of natural plant toxins. Such techniques take advantage of the stable and heritable RNAi phenotype in plant stocks. Cotton seeds are rich in dietary protein but naturally contain the toxic terpenoid product gossypol, making them unsuitable for human consumption. RNAi has been used to produce cotton stocks whose seeds contain reduced levels of delta-cadinene synthase, a key enzyme in gossypol production, without affecting the enzyme's production in other parts of the plant, where gossypol is itself important in preventing damage from plant pests.[169]
Development efforts have successfully reduced the levels of allergens in tomato plants[170] and fortification of plants such as tomatoes with dietary antioxidants.[171] RNAi silencing of alpha-amylase have also been used to decrease Aspergillus flavus fungal growth in maize which would have otherwise contaminated the kernels with dangerous aflatoxins.[172] Silencing lachrymatory factor synthase in onions have produced tearless onions and RNAi has been used in BP1 genes in rapeseeds to improve photosynthesis.[173] SBEIIa and SBEIIb genes in wheat have been targeted in wheat in order to produce higher levels of amylose in order to improve bowel function,[174] and Travella et al. 2006 employed RNAi for functional genomics an investigation of hexaploid bread races, while virus-induced gene silencing (VIGS, a subtype of RNAi) was used by Scofield et al. 2005 to investigate the mechanism of resistance provided by Lr21 against wheat leaf rust in hexaploid wheat.[175]
Insecticides
[edit]RNAi is under development as an insecticide, employing multiple approaches, including genetic engineering and topical application.[4] Cells in the midgut of some insects take up the dsRNA molecules in the process referred to as environmental RNAi.[176] In some insects the effect is systemic as the signal spreads throughout the insect's body (referred to as systemic RNAi).[177]
Animals exposed to RNAi at doses millions of times higher than anticipated human exposure levels show no adverse effects.[178] RNAi has varying effects in different species of Lepidoptera (butterflies and moths).[179]
Drosophila spp., Bombyx mori, Locusta spp., Spodoptera spp., Tribolium castaneum, Nilaparvata lugens, Helicoverpa armigera, and Apis mellifera are models that have been widely used to learn how RNAi works within particular taxa of insects. Musca domestica has two Ago2 genes and Glossina morsitans three, as found by Lewis et al. 2016 and Hain et al. 2010.[180][181] In the case of the miRNA pathway Diuraphis noxia has two Ago1s, M. domestica two Dcr1s, Acyrthosiphon pisum two each of Ago1 and Loqs and Dcr1 and four of Pasha. While in the piRNA, G. morsitans and A. pisum have two or three Ago3s each.[181] This has led to identification of future insecticide development targets, and the modes of action and reasons for insecticide resistance of other insecticides.[181]
Transgenic plants
[edit]Transgenic crops have been made to express dsRNA, carefully chosen to silence crucial genes in target pests. These dsRNAs are designed to affect only insects that express specific gene sequences. As a proof of principle, in 2009 a study showed RNAs that could kill any one of four fruit fly species while not harming the other three.[4]
Topical
[edit]Alternatively dsRNA can be supplied without genetic engineering. One approach is to add them to irrigation water. The molecules are absorbed into the plants' vascular system and poison insects feeding on them. Another approach involves spraying dsRNA like a conventional pesticide. This would allow faster adaptation to resistance. Such approaches would require low cost sources of dsRNAs that do not currently exist.[4]
Functional genomics
[edit]Подходы к дизайну библиотек RNAi по всему геному могут потребовать большей изощренности, чем конструкция одной миРНК для определенного набора экспериментальных условий. Искусственные нейронные сети часто используются для проектирования SIRNA библиотек [ 182 ] и предсказать их вероятной эффективности при нокдауне гена. [ 183 ] Массовый геномный скрининг широко рассматривается как многообещающий метод аннотации генома и вызвал разработку высокопроизводительных методов скрининга на основе микрочипов . [ 184 ] [ 185 ]
Скрининг генома
[ редактировать ]Исследование RNAI в масштабе генома зависит от технологии высокопроизводительного скрининга (HTS). Технология RNAI HTS позволяет скринингу потерь по потере функции по всему геному и широко используется при идентификации генов, связанных со специфическими фенотипами. Эта технология была провозглашена как потенциальная вторая волна геномики, после первой волны геномики экспрессии генов микрочипов и полиморфизма с одним нуклеотидным полиморфизмом . платформ обнаружения [ 186 ] Одним из основных преимуществ скрининга RNAi в масштабе генома является его способность одновременно опросить тысячи генов. С возможностью генерирования большого количества данных на эксперимент, скрининг RNAI в масштабе генома привел к взрыву скоростей генерации данных. Использование таких крупных наборов данных является фундаментальной проблемой, требующей подходящей статистики/биоинформатики. Основной процесс скрининга RNAI на основе клеток включает в себя выбор библиотеки RNAI, надежных и стабильных типов клеток, трансфекцию с помощью агентов RNAI, лечения/инкубации, обнаружения сигналов, анализа и идентификации важных генов или терапевтических мишеней. [ 187 ]
История
[ редактировать ]РНКИ открытие
[ редактировать ]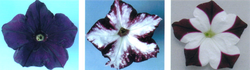
Процесс РНКи был назван «совместной категорией» и «подача», когда наблюдалось до знания механизма, связанного с РНК. Открытию РНКи предшествовали сначала наблюдения за ингибированием транскрипции антисмысловой РНК, экспрессируемой в трансгенных растениях, [ 189 ] и более прямо по сообщениям о неожиданных результатах в экспериментах, выполняемых учеными растения в Соединенных Штатах и Нидерландах в начале 1990 -х годов. [ 190 ] В попытке изменить цветы цветов в петуниях , исследователи ввели дополнительные копии гена, кодирующей халконесинтазу , ключевой фермент для пигментации цветов в растения петунии обычно розового или фиолетового цветового цвета. Ожидалось, что ген из сверхэкспрессии приведет к более темным цветам, но вместо этого заставил некоторые цветы иметь менее заметный фиолетовый пигмент, иногда в разнообразных узорах, что указывает на то, что активность халконе синтазы была существенно снижена или подавлялась специфичным для контекста. Позже это будет объяснено как результат того, что трансген вставлен рядом с промоторами в противоположном направлении в различных положениях по всему геномам некоторых трансформантов, что приводит к экспрессии антисмысловых транскриптов и молчания генов, когда эти промоторы активны. [ Цитация необходима ] Еще одно раннее наблюдение за РНКИ произошло из исследования грибной нейроспоры Crassa , [ 191 ] Хотя это не было сразу признано связанным. Дальнейшее исследование явления у растений показало, что подавление было связано с посттранскрипционным ингибированием экспрессии генов посредством повышенной скорости деградации мРНК. [ 192 ] Это явление называлось совместно-супрессией экспрессии генов , но молекулярный механизм оставался неизвестным. [ 193 ]
Вскоре после этого вирологи растений , работающие над повышением устойчивости растений к вирусным заболеваниям, наблюдали за аналогичным неожиданным явлением. Хотя было известно, что растения, экспрессирующие вирус-специфические белки, демонстрировали повышенную толерантность или устойчивость к вирусной инфекции, не ожидалось, что растения, несущие только короткие, некодирующие области последовательностей вирусной РНК, будут иметь сходные уровни защиты. Исследователи полагали, что вирусная РНК, продуцируемая трансгенами, также может ингибировать репликацию вируса. [ 194 ] Обратный эксперимент, в котором короткие последовательности генов растений были введены в вирусы, показал, что целевой ген был подавлен в инфицированном растении. [ 195 ] Это явление было помечено «индуцированным вирусом генов» (VIGS), [ 175 ] и набор таких явлений был коллективно называется пост -транскрипционным молчанием генов. [ 196 ]
После этих первоначальных наблюдений в растениях лаборатории искали это явление в других организмах. [ 197 ] [ 198 ] Первый случай молчания РНК у животных была задокументирована в 1996 году, когда Го и Кемфу заметили, что, введя смысл и антисмысловую РНК в мРНК PAR-1 у Caenorhabditis elegans , вызвало деградацию сообщения PAR-1. [ 199 ] Считалось, что эта деградация была вызвана одноцепочечной РНК (SSRNA), но два года спустя, в 1998 году, Fire и Mello обнаружили, что эта способность молчать экспрессию гена PAR-1 была фактически вызвана двумя цельной РНК (дцРНК ) [ 199 ] Крейг С. Мелло и Эндрю Фьер 1998 года Nature Paper сообщил о мощном эффекте молчания генов после введения двойной страной РНК в C. elegans . [ 200 ] При исследовании регуляции выработки мышечного белка они наблюдали, что ни мРНК, ни антисмысловая инъекции РНК не оказывали влияния на продукцию белка, но двухцепочечная РНК успешно замолчала целевой ген. В результате этой работы они придумали термин RNAI . Это открытие представляло собой первую идентификацию причинного агента для этого явления. Fire и Mello были удостоены Нобелевской премии 2006 года по физиологии или медицине . [ 6 ] [ 201 ]
RNAI Therapeutics
[ редактировать ]Сразу после пожара и новаторского открытия Мелло, Elbashir et al. Обнаружено, используя синтетически сделанную небольшую интерферирующую РНК (миРНК), можно было нацелить молчание специфических последовательностей в гене, а не молчать весь ген. [ 202 ] Только год спустя МакКаффри и его коллеги продемонстрировали, что это специфическое из последовательности молчание имело терапевтические применения, нацеленные на последовательность вируса гепатита С у трансгенных мышей. [ 203 ] С тех пор несколько исследователей пытались расширить терапевтические применения RNAI, в частности, стремятся нацелить гены, которые вызывают различные виды рака . [ 204 ] [ 205 ] К 2006 году первое применение для достижения клинических испытаний проводилось в лечении дегенерации желтого пятна и респираторного синцитиального вируса . [ 206 ] Четыре года спустя было начато первое клиническое исследование фазы I фазы с использованием системы доставки наночастиц для нацеливания на твердые опухоли . [ 207 ]
FDA одобрило первое лекарство на основе SIRNA ( Patisiran ) в 2018 году. Givosiran и Lumasiran впоследствии получили одобрение FDA для лечения AHP и PH1 в 2019 и 2020 годах соответственно. [ 113 ] Inclisiran получил одобрение EMA в 2020 году на лечение высокого уровня холестерина и в настоящее время находится в рассмотрении FDA. [ 208 ]
Смотрите также
[ редактировать ]Ссылки
[ редактировать ]- ^ Saurabh S, Vidyarthi AS, Prasad D (март 2014 г.). «Вмешательство РНК: концепция реальности в улучшении урожая» . Планта 239 (3): 543–64. BIBCODE : 2014Plant.239..543S . doi : 10.1007/s00425-013-2019-5 . PMID 24402564 .
- ^ Вайс Б., Дэвидкова Г., Чжоу Л.В. (март 1999 г.). «Антисмысленная генная терапия РНК для изучения и модуляции биологических процессов» . Клеточные и молекулярные науки о жизни . 55 (3): 334–58. doi : 10.1007/s000180050296 . PMC 11146801 . PMID 10228554 . S2CID 9448271 .
- ^ Jump up to: а беременный Matranga C, Tomari Y, Shin C, Bartel DP, Zamore PD (ноябрь 2005 г.). «Расщепление пассажирских цепей облегчает сборку миРНК в EAGY2-содержащие ферментные комплексы RNAI» . Клетка . 123 (4): 607–20. doi : 10.1016/j.cell.2005.08.044 . PMID 16271386 .
- ^ Jump up to: а беременный в дюймовый Kupferschmidt K (август 2013 г.). «Смертельная доза РНК» . Наука . 341 (6147): 732–3. Bibcode : 2013sci ... 341..732K . doi : 10.1126/science.341.6147.732 . PMID 23950525 .
- ^ Macrae IJ, Zhou K, Li F, Repic A, Brooks AN, Cande WZ, et al. (Январь 2006 г.). «Структурная основа для двухцепочечной обработки РНК Dicer». Наука . 311 (5758): 195–8. Bibcode : 2006sci ... 311..195m . doi : 10.1126/science.1121638 . PMID 16410517 . S2CID 23785494 .
- ^ Jump up to: а беременный в дюймовый Данехольт Б. "Расширенная информация: РНК -интерференция" . Нобелевская премия по физиологии или медицине 2006 . Архивировано из оригинала 20 января 2007 года . Получено 25 января 2007 года .
- ^ Багасра О, Приллиман К.Р. (август 2004 г.). «Интерференция РНК: молекулярная иммунная система». Журнал молекулярной гистологии . 35 (6): 545–53. Citeseerx 10.1.1.456.1701 . doi : 10.1007/s10735-004-2192-8 . PMID 15614608 . S2CID 2966105 .
- ^ Бернштейн Е., Кауди А.А., Хаммонд С.М., Хэннон Г.Дж. (январь 2001 г.). «Роль бинтатной рибонуклеазы на стадии инициации помехи РНК». Природа . 409 (6818): 363–6. Bibcode : 2001natur.409..363b . doi : 10.1038/35053110 . PMID 11201747 . S2CID 4371481 .
- ^ Siomi H, Siomi MC (январь 2009 г.). «На пути к чтению кода РНК-интерференции». Природа . 457 (7228): 396–404. Bibcode : 2009natur.457..396s . doi : 10.1038/nature07754 . PMID 19158785 . S2CID 205215974 .
Zamore PD, Tuschl T, Sharp PA, Bartel DP (март 2000 г.). «РНКи: двухцепочечная РНК направляет АТФ-зависимое расщепление мРНК с 21-23 интервалами нуклеотидов» . Клетка . 101 (1): 25–33. doi : 10.1016/s0092-8674 (00) 80620-0 . PMID 10778853 .
Вермейлен А., Бехлен Л., Рейнольдс А., Вольфсон А., Маршалл В.С., Карпилоу Дж. И др. (Май 2005 г.). «Вклад структуры дцРНК в специфичность и эффективность DICER» . РНК . 11 (5): 674–82. doi : 10.1261/rna.7272305 . PMC 1370754 . PMID 15811921 .
Кастанотто Д., Росси Дж.Дж. (январь 2009 г.). «Обещания и подводные камни терапии на основе РНК-интерференции» . Природа . 457 (7228): 426–33. Bibcode : 2009natur.457..426c . doi : 10.1038/nature07758 . PMC 2702667 . PMID 19158789 . - ^ Jump up to: а беременный Qiu S, Adema CM, Lane T (2005). «Вычислительное исследование нецелевых эффектов интерференции РНК» . Исследование нуклеиновых кислот . 33 (6): 1834–47. doi : 10.1093/nar/gki324 . PMC 1072799 . PMID 15800213 .
- ^ Jump up to: а беременный Наканиши К (сентябрь 2016 г.). «Анатомия RISC: как небольшие РНК и шапероны активируют аргонатные белки?» Полем Wiley Междисциплинарные обзоры: РНК . 7 (5): 637–60. doi : 10.1002/wrna.1356 . PMC 5084781 . PMID 27184117 .
- ^ Liang C, Wang Y, Murota Y, Liu X, Smith D, Siomi MC, et al. (Сентябрь 2015). «TAF11 собирает комплекс нагрузки RISC для повышения эффективности RNAi» . Молекулярная клетка . 59 (5): 807–18. doi : 10.1016/j.molcel.2015.07.006 . PMC 4560963 . PMID 26257286 .
- ^ Ahlquist P (май 2002). «РНК-зависимые РНК-полимеразы, вирусы и молчание РНК». Наука . 296 (5571): 1270–3. Bibcode : 2002sci ... 296.1270a . doi : 10.1126/science.1069132 . PMID 12016304 . S2CID 42526536 .
- ^ Робертс Т.С. (2015). «Машина для микроРНК». МикроРНА: Основная наука . Достижения в области экспериментальной медицины и биологии. Тол. 887. С. 15–30. doi : 10.1007/978-3-319-22380-3_2 . ISBN 978-3-319-22379-7 Полем PMID 26662984 .
- ^ Jump up to: а беременный в дюймовый Паркер Г., Экерт Д., Басс Б. (2006). «RDE-4 преимущественно связывает длинную дцРНК, и ее димеризация необходима для расщепления дсРНК к миРНК» . РНК . 12 (5): 807–18. doi : 10.1261/rna.2338706 . PMC 1440910 . PMID 16603715 .
- ^ Jump up to: а беременный Baulcombe DC (январь 2007 г.). «Молекулярная биология. Усиленное молчание». Наука . 315 (5809): 199–200. doi : 10.1126/science.1138030 . PMID 17218517 . S2CID 46285020 .
- ^ Jump up to: а беременный Pak J, Fire A (январь 2007 г.). «Отдельные популяции первичных и вторичных эффекторов во время RNAi у C. elegans». Наука . 315 (5809): 241–4. Bibcode : 2007sci ... 315..241p . doi : 10.1126/science.1132839 . PMID 17124291 . S2CID 46620298 .
- ^ Jump up to: а беременный Sijen T, Steiner FA, Thijssen KL, Plasterk RH (январь 2007 г.). «Вторичные миРНК возникают из -за бесвязного синтеза РНК и образуют отдельный класс». Наука . 315 (5809): 244–7. Bibcode : 2007sci ... 315..244S . doi : 10.1126/science.1136699 . PMID 17158288 . S2CID 9483460 . (Втянут, см doi : 10.1126/science.abf4837 , PMID 33214285 , Retraction Watch )
- ^ Wang Ql, Li ZH (май 2007 г.). «Функции микроРНК в растениях» . Границы в биологии . 12 : 3975–82. doi : 10.2741/2364 . PMC 2851543 . PMID 17485351 . S2CID 23014413 .
Zhao Y, Srivastava D (апрель 2007 г.). «Просмотр функции микроРНК». Тенденции в биохимических науках . 32 (4): 189–97. doi : 10.1016/j.tibs.2007.02.006 . PMID 17350266 . - ^ Грегори Р.И., Чендримада Т.П., Шихаттар Р. (2006). «Биогенез микроРНК: выделение и характеристика микропроцессорного комплекса». Протоколы микроРНК . Методы в молекулярной биологии. Тол. 342. С. 33–47. doi : 10.1385/1-59745-123-1: 33 . ISBN 978-1-59745-123-9 Полем PMID 16957365 .
- ^ Pfeffer S, Zavolan M, Grässer FA, Chien M, Russo JJ, Ju J, et al. (Апрель 2004 г.). «Идентификация кодируемых вирусом микроРНК». Наука . 304 (5671): 734–6. Bibcode : 2004sci ... 304..734p . doi : 10.1126/science.1096781 . PMID 15118162 . S2CID 25287167 .
- ^ Qureshi A, Thakur N, Monga I, Thakur A, Kumar M (1 января 2014 года). «Virmirna: комплексный ресурс для экспериментально проверенных вирусных микроРНК и их мишеней» . База данных . 2014 : BAU103. doi : 10.1093/база данных/BAU103 . PMC 4224276 . PMID 25380780 .
- ^ Pillai RS, Bhattacharyya SN, Filipowicz W (2007). «Репрессия синтеза белка с помощью miRNAS: сколько механизмов?». Тенденции Cell Biol . 17 (3): 118–26. doi : 10.1016/j.tcb.2006.12.007 . PMID 17197185 .
- ^ Окамура К., Ишизука А., Сиоми Х., Сиоми М. (2004). «Отличительные роли для аргонатных белков в небольших путях расщепления РНК, направленных на РНК» . Гены Дев . 18 (14): 1655–66. doi : 10.1101/gad.1210204 . PMC 478188 . PMID 15231716 .
- ^ Ли Y, Накахара К., Фам Дж, Ким К, Хе З, Сонтхеймер Е. и др. (2004). «Отдельные роли для Drosophila Dicer-1 и Dicer-2 в путях молчания siRNA/miRNA» . Клетка . 117 (1): 69–81. doi : 10.1016/s0092-8674 (04) 00261-2 . PMID 15066283 .
- ^ mirbase.org
- ^ Jump up to: а беременный Friedman RC, Farh KK, Burge CB, Bartel DP (2009). «Большинство мРНК млекопитающих являются консервативными целями микроРНК» . Геном Res . 19 (1): 92–105. doi : 10.1101/gr.082701.108 . PMC 2612969 . PMID 18955434 .
- ^ Lim LP, Lau NC, Garrett-Engele P, Grimson A, Schelter JM, Castle J, et al. (Февраль 2005 г.). «Анализ микрочипов показывает, что некоторые микроРНК подавляют большое количество целевых мРНК». Природа . 433 (7027): 769–73. Bibcode : 2005natur.433..769L . doi : 10.1038/nature03315 . PMID 15685193 . S2CID 4430576 .
- ^ Selbach M, Schwanhäusser B, Thierfelder N, Fang Z, Khanin R, Rajewsky N (сентябрь 2008 г.). «Широко распространенные изменения в синтезе белка, вызванные микроРНК». Природа . 455 (7209): 58–63. Bibcode : 2008natur.455 ... 58 с . doi : 10.1038/nature07228 . PMID 18668040 . S2CID 4429008 .
- ^ Baek D, Villén J, Shin C, Camargo FD, Gygi SP, Bartel DP (сентябрь 2008 г.). «Влияние микроРНК на выход белка» . Природа . 455 (7209): 64–71. Bibcode : 2008natur.455 ... 64b . doi : 10.1038/nature07242 . PMC 2745094 . PMID 18668037 .
- ^ Palmer EI, из Campos SG, Campos M, De Souza NC, Warrior ID, Carvalho AL, et al. (Июль 2011). «Механизмы и роль микронной дерегуляции в начале и прогрессировании рака» . Биология генетика и молекулярная . 34 (3): 363–70. Doi : 10.1590/s1415-47572011000300001 . PMC 3168173 . PMID 21931505 .
- ^ Бернштейн С, Бернштейн Х (май 2015). «Эпигенетическое восстановление репарации ДНК при прогрессировании на желудочно -кишечный рак» . Всемирный журнал желудочно -кишечной онкологии . 7 (5): 30–46. doi : 10.4251/wjgo.v7.i5.30 . PMC 4434036 . PMID 25987950 .
- ^ Maffioletti E, Tardito D, Gennarelli M, Bocchio-Chiavetto L (2014). «Микро -шпионы от мозга до периферии: новые подсказки из исследований микроРНК при нейропсихиатрических расстройствах» . Границы в клеточной нейробиологии . 8 : 75. DOI : 10.3389/fncel.2014.00075 . PMC 3949217 . PMID 24653674 .
- ^ Mellios N, Sur M (2012). «Новая роль микроРНК в расстройствах шизофрении и аутистического спектра» . Границы в психиатрии . 3 : 39. doi : 10.3389/fpsyt.2012.00039 . PMC 3336189 . PMID 22539927 .
- ^ Geaghan M, Cairns MJ (август 2015 г.). «МикроРНК и посттранскрипционная дисрегуляция в психиатрии» . Биологическая психиатрия . 78 (4): 231–9. doi : 10.1016/j.biopsych.2014.12.009 . HDL : 1959.13/1335073 . PMID 25636176 .
- ^ Liu Q, Rand TA, Kalidas S, Du F, Kim HE, Smith DP, et al. (Сентябрь 2003 г.). «R2D2, мост между инициацией и эффекторными этапами пути Drosophila RNAI». Наука . 301 (5641): 1921–5. Bibcode : 2003sci ... 301.1921L . doi : 10.1126/science.1088710 . PMID 14512631 . S2CID 41436233 .
- ^ Грегори Р.И., Чендримада Т.П., Куч Н., Шихаттар Р (ноябрь 2005 г.). «Человеческий RISC объединяет биогенез микроРНК и посттранскрипционное молчание генов» . Клетка . 123 (4): 631–40. doi : 10.1016/j.cell.2005.10.022 . PMID 16271387 .
- ^ Jump up to: а беременный Lodish H, Berk A, Matsudaira P, Kaiser CA, Krieger M, Scott MP, et al. (2004). Молекулярная клеточная биология (5 -е изд.). WH Freeman: Нью -Йорк, Нью -Йорк. ISBN 978-0-7167-4366-8 .
- ^ Leuschner PJ, Ameres SL, Kueng S, Martinez J (март 2006 г.). «Расщепление пассажирской нити миРНК во время сборки RISC в клетках человека» . Embo сообщает . 7 (3): 314–20. doi : 10.1038/sj.embor.7400637 . PMC 1456892 . PMID 16439995 .
- ^ Jump up to: а беременный Хейли Б., Замор П.Д. (июль 2004 г.). «Кинетический анализ ферментного комплекса РНКи». Природа структурная и молекулярная биология . 11 (7): 599–606. doi : 10.1038/nsmb780 . PMID 15170178 . S2CID 12400060 .
- ^ Schwarz DS, Hutvágner G, Du T, Xu Z, Aronin N, Zamore PD (октябрь 2003 г.). «Асимметрия в сборке ферментного комплекса РНКи» . Клетка . 115 (2): 199–208. doi : 10.1016/s0092-8674 (03) 00759-1 . PMID 14567917 .
- ^ Preall JB, He Z, Gorra JM, Sontheimer EJ (март 2006 г.). «Короткий мешающий отбор РНК не зависит от полярности обработки дцРНК во время РНКи у дрозофилы» . Текущая биология . 16 (5): 530–5. Bibcode : 2006cbio ... 16..530p . doi : 10.1016/j.cub.2006.01.061 . PMID 16527750 .
- ^ Tomari Y, Matranga C, Haley B, Martinez N, Zamore PD (ноябрь 2004 г.). «Белковый датчик для асимметрии миРНК». Наука . 306 (5700): 1377–80. Bibcode : 2004sci ... 306.1377t . doi : 10.1126/science.1102755 . PMID 15550672 . S2CID 31558409 .
- ^ MA JB, Yuan YR, Meister G, Pei Y, Tuschl T, Patel DJ (март 2005 г.). «Структурная основа для 5'-эндов-специфического распознавания направляющей РНК белком A. fulgidus piwi» . Природа . 434 (7033): 666–70. Bibcode : 2005natur.434..666m . doi : 10.1038/nature03514 . PMC 4694588 . PMID 15800629 .
- ^ Sen G, Wehrman T, Blau H (2005). «Трансляция мРНК не является предпосылкой для небольшого мешающего РНК-опосредованного расщепления мРНК». Дифференциация . 73 (6): 287–93. doi : 10.1111/j.1432-0436.2005.00029.x . PMID 16138829 . S2CID 41117614 .
- ^ Гу С., Росси Дж. (2005). «Необеспечение РНКи от активной трансляции в клетках млекопитающих» . РНК . 11 (1): 38–44. doi : 10.1261/rna.7158605 . PMC 1370689 . PMID 15574516 .
- ^ Sen G, Blau H (2005). «Аргонат 2/RISC находится на местах распада мРНК млекопитающих, известного как цитоплазматические тела». Nat Cell Biol . 7 (6): 633–6. doi : 10.1038/ncb1265 . PMID 15908945 . S2CID 6085169 .
- ^ Лиан С., Джакимиу А., Эйстатхии Т., Хэмел Дж., Фрицлер М., Чан Е. (2006). «Тела GW, микроРНК и клеточный цикл» . Клеточный цикл . 5 (3): 242–5. doi : 10.4161/cc.5.3.2410 . PMID 16418578 .
- ^ Jakymiw A, Lian S, Eystathioy T, Li S, Satoh M, Hamel J, et al. (2005). «Разрушение P тел ухудшает вмешательство РНК млекопитающих». Nat Cell Biol . 7 (12): 1267–74. doi : 10.1038/ncb1334 . PMID 16284622 . S2CID 36630239 .
- ^ Hammond S, Bernstein E, Beach D, Hannon G (2000). «РНК-направленная нуклеаза опосредует посттранскрипционное молчание генов в клетках дрозофилы». Природа . 404 (6775): 293–6. Bibcode : 2000natur.404..293H . doi : 10.1038/35005107 . PMID 10749213 . S2CID 9091863 .
- ^ Холмквист Г.П., Эшли Т. (2006). «Организация хромосом и модификация хроматина: влияние на функцию генома и эволюцию». Цитогенетические и геномные исследования . 114 (2): 96–125. doi : 10.1159/000093326 . PMID 16825762 . S2CID 29910065 .
- ^ Вердель А., Цзя С., Гербер С., Сугияма Т., Гиги С., Гревал С.И. и др. (Январь 2004 г.). «RNAI-опосредованное нацеливание гетерохроматина комплексом RITS» . Наука . 303 (5658): 672–6. Bibcode : 2004sci ... 303..672V . doi : 10.1126/science.1093686 . PMC 3244756 . PMID 14704433 .
- ^ Ирвин Д.В., Заратиги М., Толиа Н.Х., Гото Д.Б., Читвуд Д.Х., Вон М.В. и др. (Август 2006 г.). «Аргонаутная нарезка требуется для гетерохроматического молчания и распространения». Наука . 313 (5790): 1134–7. Bibcode : 2006sci ... 313.1134i . doi : 10.1126/science.1128813 . PMID 16931764 . S2CID 42997104 .
- ^ Volpe TA, Kidner C, Hall IM, Teng G, Grewal SI, Martienssen RA (сентябрь 2002 г.). «Регуляция гетерохроматического молчания и метилирования лизина-9 гистона H3 с помощью РНКи» . Наука . 297 (5588): 1833–7. Bibcode : 2002sci ... 297.1833v . doi : 10.1126/science.1074973 . PMID 12193640 . S2CID 2613813 .
- ^ Volpe T, Schramke V, Hamilton GL, White SA, Teng G, Martienssen RA, et al. (2003). «РНК -интерференция требуется для нормальной функции центромеры у делящихся дрожжей». Исследование хромосом . 11 (2): 137–46. doi : 10.1023/a: 1022815931524 . PMID 12733640 . S2CID 23813417 .
- ^ Li LC, Okino St, Zhao H, Pookot D, Place RF, Urakami S, et al. (Ноябрь 2006 г.). «Маленькие дцРНК индуцируют активацию транскрипции в клетках человека» . Труды Национальной академии наук Соединенных Штатов Америки . 103 (46): 17337–42. Bibcode : 2006pnas..10317337L . doi : 10.1073/pnas.0607015103 . PMC 1859931 . PMID 17085592 .
- ^ Noma K, Sugiyama T, Cam H, Verdel A, Zofall M, Jia S, et al. (Ноябрь 2004 г.). «RITS действует в CIS, чтобы способствовать опосредованному интерферентному интерферентному интерференции транскрипции и посттранскрипционного молчания» . Природа генетика . 36 (11): 1174–80. doi : 10.1038/ng1452 . PMID 15475954 .
- ^ Sugiyama T, Cam H, Verdel A, Moazed D, Grewal Si (январь 2005 г.). «РНК-зависимая РНК-полимераза является важным компонентом самосогловой сцепления сцепления гетерохроматина с выработкой миРНК» . Труды Национальной академии наук Соединенных Штатов Америки . 102 (1): 152–7. Bibcode : 2005pnas..102..152S . doi : 10.1073/pnas.0407641102 . PMC 544066 . PMID 15615848 .
- ^ Wang F, Koyama N, Nishida H, Haraguchi T, Reith W, Tsukamoto T (июнь 2006 г.). «Сборка и поддержание гетерохроматина, инициируемого трансгеновыми повторами, не зависят от пути интерференции РНК в клетках млекопитающих» . Молекулярная и клеточная биология . 26 (11): 4028–40. doi : 10.1128/mcb.02189-05 . PMC 1489094 . PMID 16705157 .
- ^ Басс Бл (2002). «Редактирование РНК с помощью аденозин деминаз, которые действуют на РНК» . Ежегодный обзор биохимии . 71 : 817–46. doi : 10.1146/annurev.biochem.71.110601.135501 . PMC 1823043 . PMID 12045112 .
- ^ Басс BL (апрель 2000 г.). «Двухцепочечная РНК как матрица для молчания генов» . Клетка . 101 (3): 235–8. doi : 10.1016/s0092-8674 (02) 71133-1 . PMID 10847677 .
- ^ Luciano DJ, Mirsky H, Vendetti NJ, Maas S (август 2004 г.). «РНК -редактирование предшественника miRNA» . РНК . 10 (8): 1174–7. doi : 10.1261/rna.7350304 . PMC 1370607 . PMID 15272117 .
- ^ Jump up to: а беременный Ян В., Чендримада Т.П., Ван К., Хигучи М., Сибург П.П., Шихаттар Р. и др. (Январь 2006 г.). «Модуляция обработки и экспрессии микроРНК посредством редактирования РНК адаром деминаз» . Природа структурная и молекулярная биология . 13 (1): 13–21. doi : 10.1038/nsmb1041 . PMC 2950615 . PMID 16369484 .
- ^ Yang W, Wang Q, Howell KL, Lee JT, Cho DS, Murray JM, et al. (Февраль 2005 г.). «Адар1 РНК -деаминаза ограничивает короткую мешающую эффективность РНК в клетках млекопитающих» . Журнал биологической химии . 280 (5): 3946–53. doi : 10.1074/jbc.m407876200 . PMC 2947832 . PMID 15556947 .
- ^ Нишикура К (декабрь 2006 г.). «Редактор встречает глушитель: перекрестные помехи между редактированием РНК и интерференцией РНК» . Природа обзор молекулярной клеточной биологии . 7 (12): 919–31. doi : 10.1038/nrm2061 . PMC 2953463 . PMID 17139332 .
- ^ Jump up to: а беременный в Saumet A, Lecellier CH (2006). "Антивирусное молчание РНК: мы похожи на растения?" Полем Ретровирология . 3 (1): 3. DOI : 10.1186/1742-4690-3-3 . PMC 1363733 . PMID 16409629 .
- ^ Jones L, Ratcliff F, Baulcombe DC (май 2001 г.). «РНК-направленное молчание транскрипционного гена в растениях может быть унаследовано независимо от триггера РНК и требует MET1 для поддержания» . Текущая биология . 11 (10): 747–57. Bibcode : 2001cbio ... 11..747j . doi : 10.1016/s0960-9822 (01) 00226-3 . PMID 11378384 . S2CID 16789197 .
- ^ Humphreys DT, Westman BJ, Martin Di, Preiss T (ноябрь 2005 г.). «МикроРНК контролирует инициацию трансляции путем ингибирования эукариотического фактора инициации 4E/CAP и поли (A) хвоста» . Труды Национальной академии наук Соединенных Штатов Америки . 102 (47): 16961–6. Bibcode : 2005pnas..10216961H . doi : 10.1073/pnas.0506482102 . PMC 1287990 . PMID 16287976 .
- ^ Darocha WD, Otsu K, Teixeira SM, Donelson Je (февраль 2004 г.). «Тесты цитоплазматического интерференции РНК (RNAi) и построение промоторной системы TTRACECLINE-индуцируемой T7 в Trypanosoma Cruzi». Молекулярная и биохимическая паразитология . 133 (2): 175–86. doi : 10.1016/j.molbiopara.2003.10.005 . PMID 14698430 .
- ^ Робинсон К.А., Беверли С.М. (май 2003 г.). «Улучшения в эффективности трансфекции и тестов подходов РНК -интерференции (RNAi) в простейском паразите Leishmania». Молекулярная и биохимическая паразитология . 128 (2): 217–28. doi : 10.1016/s0166-6851 (03) 00079-3 . PMID 12742588 .
- ^ Aravind L, Watanabe H, Lipman DJ, Koonin EV (октябрь 2000 г.). «Специфичная для линии потеря и дивергенция функционально связанных генов у эукариот» . Труды Национальной академии наук Соединенных Штатов Америки . 97 (21): 11319–24. Bibcode : 2000pnas ... 9711319a . doi : 10.1073/pnas.200346997 . PMC 17198 . PMID 11016957 .
- ^ Дринненберг И.А., Вайнберг Д.Е., Се К.Т., Мауэр Дж.П., Вулф К.Х., Финк Г.Р. и др. (Октябрь 2009 г.). «РНКИ в начинающих дрожжах» . Наука . 326 (5952): 544–550. Bibcode : 2009sci ... 326..544d . doi : 10.1126/science.1176945 . PMC 3786161 . PMID 19745116 .
- ^ Nakayashiki H, Kadotani N, Mayama S (июль 2006 г.). «Эволюция и диверсификация белков с молчанием РНК в грибах» (PDF) . Журнал молекулярной эволюции . 63 (1): 127–35. Bibcode : 2006jmole..63..127n . doi : 10.1007/s00239-005-0257-2 . PMID 16786437 . S2CID 22639035 . Архивировано из оригинала (PDF) 6 августа 2020 года . Получено 4 декабря 2019 года .
- ^ Морита Т., Мочизуки Ю., Айба Х (март 2006 г.). «Трансляционная репрессия достаточна для молчания генов бактериальными небольшими некодирующими РНК в отсутствие разрушения мРНК» . Труды Национальной академии наук Соединенных Штатов Америки . 103 (13): 4858–63. Bibcode : 2006pnas..103.4858m . doi : 10.1073/pnas.0509638103 . PMC 1458760 . PMID 16549791 .
- ^ Макарова К.С., Гришин Н.В., Шабалина С.А., Вольф Йи, Кунин Э.В. (март 2006 г.). «Предполагаемая иммунная система на основе РНК в прокариотах: вычислительный анализ прогнозируемого ферментативного механизма, функциональные аналогии с эукариотической РНКи и гипотетические механизмы действия» . Биология прямой . 1 : 7. doi : 10.1186/1745-6150-1-7 . PMC 1462988 . PMID 16545108 .
- ^ Stram Y, Kuzntzova L (июнь 2006 г.). «Ингибирование вирусов с помощью РНК -интерференции» . Вирусные гены . 32 (3): 299–306. doi : 10.1007/s11262-005-6914-0 . PMC 7088519 . PMID 16732482 .
- ^ Блевинс Т., Раджесваран Р., Шивапрасад П.В., Беназаряны Д., Си-Аммур А., Парк Х.С. и др. (2006). «Четыре изделия из растений опосредуют вирусный малый РНК -биогенез, а ДНК -вирус индуцировал молчание» . Исследование нуклеиновых кислот . 34 (21): 6233–46. doi : 10.1093/nar/gkl886 . PMC 1669714 . PMID 17090584 .
- ^ Palauqui JC, Elmayan T, Pollien JM, Vaucheret H (август 1997 г.). «Системное приобретенное молчание: трансгене-специфическое посттранскрипционное молчание передается путем прививки от молчаливных запасов к не приличным Scions» . Embo Journal . 16 (15): 4738–45. doi : 10.1093/emboj/16.15.4738 . PMC 1170100 . PMID 9303318 .
- ^ Voinnet O (август 2001 г.). «Глушение РНК как иммунная система растений против вирусов». Тенденции в генетике . 17 (8): 449–59. doi : 10.1016/s0168-9525 (01) 02367-8 . PMID 11485817 .
- ^ Люси AP, Guo HS, Li WX, Ding SW (апрель 2000 г.). «Подавление посттранскрипционного молчания гена вирусным белком растения, локализованное в ядре» . Embo Journal . 19 (7): 1672–80. doi : 10.1093/emboj/19.7.1672 . PMC 310235 . PMID 10747034 .
- ^ Mérai Z, Kerényi Z, Kertész S, Magna M, Lakatos L, Silhavy D (июнь 2006 г.). «Двойное связывание РНК может быть общей вирусной стратегией РНК растительной РНК для подавления молчания РНК» . Журнал вирусологии . 80 (12): 5747–56. doi : 10.1128/jvi.01963-05 . PMC 1472586 . PMID 16731914 .
- ^ Katiyar-Agarwal S, Morgan R, Dahlbeck D, Borsani O, Villegas A, Zhu JK, et al. (Ноябрь 2006 г.). «Индуцируемая патогеном эндогенная миРНК при иммунитете растений» . Труды Национальной академии наук Соединенных Штатов Америки . 103 (47): 18002–7. Bibcode : 2006pnas..10318002K . doi : 10.1073/pnas.0608258103 . PMC 1693862 . PMID 17071740 .
- ^ Фриц Дж. Х., Жирардин С.Е., Филпотт DJ (июнь 2006 г.). «Врожденная иммунная защита через РНК -вмешательство». Science's Stke . 2006 (339): PE27. doi : 10.1126/stke.3392006pe27 . PMID 16772641 . S2CID 33972766 .
- ^ Замбон Р.А., Вахария Вн, Ву Л.П. (май 2006 г.). «РНКи является противовирусным иммунным ответом на вирус дцРНК у дрозофилы меланогастер» . Клеточная микробиология . 8 (5): 880–9. doi : 10.1111/j.1462-5822.2006.00688.x . PMID 16611236 . S2CID 32439482 .
- ^ Wang XH, Aliyari R, Li WX, Li HW, Kim K, Carthew R, et al. (Апрель 2006 г.). «РНК -интерференция направляет врожденный иммунитет против вирусов у взрослых дрозофилы» . Наука . 312 (5772): 452–4. Bibcode : 2006sci ... 312..452W . doi : 10.1126/science.1125694 . PMC 1509097 . PMID 16556799 .
- ^ Lu R, Maduro M, Li F, Li HW, Broitman-Maduro G, Li WX, et al. (Август 2005 г.). «Репликация вируса животных и RNAi-опосредованное антивирусное молчание у Caenorhabditis elegans» . Природа . 436 (7053): 1040–1043. Bibcode : 2005natur.436.1040L . doi : 10.1038/nature03870 . PMC 1388260 . PMID 16107851 .
- ^ Wilkins C, Dishongh R, Moore SC, Whitt MA, Chow M, Machaca K (август 2005 г.). «Вмешательство РНК является механизм антивирусной защиты у Caenorhabditis elegans». Природа . 436 (7053): 1044–7. Bibcode : 2005natur.436.1044W . doi : 10.1038/nature03957 . PMID 16107852 . S2CID 4431035 .
- ^ Berkhout B, Haasnoot J (май 2006 г.). «Взаимодействие между вирусной инфекцией и механизмом клеточной РНК -интерференции» . Письма Febs . 580 (12): 2896–902. BIBCODE : 2006FEBSL.580.2896B . doi : 10.1016/j.febslet.2006.02.070 . PMC 7094296 . PMID 16563388 .
- ^ Schütz S, Sarnow P (январь 2006 г.). «Взаимодействие вирусов с путем помехи РНК млекопитающих» . Вирусология . 344 (1): 151–7. doi : 10.1016/j.virol.2005.09.034 . PMID 16364746 .
- ^ Cullen BR (июнь 2006 г.). «Является ли РНК -интерференция вовлечена в внутренний противовирусенный иммунитет у млекопитающих?». Природа иммунология . 7 (6): 563–7. doi : 10.1038/ni1352 . PMID 16715068 . S2CID 23467688 .
- ^ Maillard PV, Ciaudo C, Marchais A, Li Y, Jay F, Ding SW, et al. (Октябрь 2013). «Антивирусное интерференцию РНК в клетках млекопитающих» . Наука . 342 (6155): 235–8. Bibcode : 2013sci ... 342..235M . doi : 10.1126/science.1241930 . PMC 3853215 . PMID 24115438 .
- ^ Li Y, Lu J, Han Y, Fan X, Ding SW (октябрь 2013). «РНК -интерференция функционирует как механизм противовирусного иммунитета у млекопитающих» . Наука . 342 (6155): 231–4. Bibcode : 2013sci ... 342..231L . doi : 10.1126/science.1241911 . PMC 3875315 . PMID 24115437 .
- ^ Li HW, Ding SW (октябрь 2005 г.). «Противовирусное молчание у животных» . Письма Febs . 579 (26): 5965–73. Bibcode : 2005 Febsl.579.5965L . doi : 10.1016/j.febslet.2005.08.034 . PMC 1350842 . PMID 16154568 .
- ^ Carrington JC, Ambros V (июль 2003 г.). «Роль микроРНК в развитии растений и животных» . Наука . 301 (5631): 336–8. Bibcode : 2003sci ... 301..336c . doi : 10.1126/science.1085242 . PMID 12869753 . S2CID 43395657 .
- ^ Lee RC, Feinbaum RL, Ambros V (декабрь 1993 г.). «Гетерохронный ген C. elegans ген Lin-4 кодирует небольшие РНК с антисмысловой комплементарностью LIN-14» . Клетка . 75 (5): 843–54. doi : 10.1016/0092-8674 (93) 90529-y . PMID 8252621 .
- ^ Palatnik JF, Allen E, Wu X, Schommer C, Schwab R, Carrington JC, et al. (Сентябрь 2003 г.). «Контроль морфогенеза листьев микроРНК» . Природа . 425 (6955): 257–63. Bibcode : 2003natur.425..257p . doi : 10.1038/nature01958 . PMID 12931144 . S2CID 992057 .
- ^ Zhang B, Pan X, Cobb GP, Anderson TA (январь 2006 г.). «МИККОРНА ПАНД: небольшая регуляторная молекула с большим ударом» . Биология развития . 289 (1): 3–16. doi : 10.1016/j.ydbio.2005.10.036 . PMID 16325172 .
- ^ Jones-Rhoades MW, Bartel DP, Bartel B (2006). «МикроРНК и их регулирующие роли в растениях». Ежегодный обзор биологии растений . 57 : 19–53. doi : 10.1146/annurev.arplant.57.032905.105218 . PMID 16669754 . S2CID 13010154 .
- ^ Zhang B, Pan X, Cobb GP, Anderson TA (февраль 2007 г.). «МикроРНК в качестве онкогена и опухолевых супрессоров» . Биология развития . 302 (1): 1–12. doi : 10.1016/j.ydbio.2006.08.028 . PMID 16989803 .
- ^ Cerutti H, Casas-Mollano JA (август 2006 г.). «О происхождении и функциях РНК-опосредованного молчания: от протистов к человеку» . Текущая генетика . 50 (2): 81–99. doi : 10.1007/s00294-006-0078-x . PMC 2583075 . PMID 16691418 .
- ^ Anantharaman V, Koonin EV, Aravind L (апрель 2002 г.). «Сравнительная геномика и эволюция белков, участвующих в метаболизме РНК» . Исследование нуклеиновых кислот . 30 (7): 1427–64. doi : 10.1093/nar/30.7.1427 . PMC 101826 . PMID 11917006 .
- ^ Voorhoeve PM, Agami R (январь 2003 г.). «Нокдаун встает». Тенденции в биотехнологии . 21 (1): 2–4. doi : 10.1016/s0167-7799 (02) 00002-1 . PMID 12480342 .
- ^ Munkácsy G, Sztupinszki Z, Herman P, Bán B, Monetary Z, Szarvas N, et al. (Сентябрь 2016 г.). «Валидация эффективности молчания РНКи с использованием данных генов показывает 18,5% частоту отказов в 429 независимых экспериментах» . Молекулярная терапия: нуклеиновые кислоты . 5 (9): E366. Doi : 10.1038/mtna.2016.66 . PMC 5056990 . PMID 27673562 .
- ^ Найто Ю., Ямада Т., Мацумия Т., Уи-Тей К, Сайго К., Моришита С. (июль 2005 г.). «DSCHECK: высокочувствительное программное обеспечение для поиска вне цели для двухцепочечных РНК-опосредованных интерференций РНК» . Исследование нуклеиновых кислот . 33 (Проблема веб -сервера): W589–91. doi : 10.1093/nar/gki419 . PMC 1160180 . PMID 15980542 .
- ^ Хеншель А., Буххольц Ф., Хаберманн Б (июль 2004 г.). «Deqor: веб-инструмент для проектирования и контроля качества SIRNAS» . Исследование нуклеиновых кислот . 32 (Проблема веб -сервера): W113–20. doi : 10.1093/nar/gkh408 . PMC 441546 . PMID 15215362 .
- ^ Найто Ю., Ямада Т., Уи-Тей К., Моришита С., Сайго К (июль 2004 г.). «Sidirect: высокоэффективное программное обеспечение для дизайна SIRNA для мировой миРНК для интерференции РНК млекопитающих» . Исследование нуклеиновых кислот . 32 (Проблема веб -сервера): W124–9. doi : 10.1093/nar/gkh442 . PMC 441580 . PMID 15215364 .
- ^ Naito Y, Ui-Tei K, Nishikawa T, Takebe Y, Saigo K (июль 2006 г.). «Sivirus: веб-программное обеспечение для дизайна антивирусной миРНК для очень расходящихся вирусных последовательностей» . Исследование нуклеиновых кислот . 34 (Проблема веб -сервера): W448–50. doi : 10.1093/nar/gkl214 . PMC 1538817 . PMID 16845046 .
- ^ Рейнольдс А., Андерсон Э.М., Вермейлен А., Федоров Ю., Робинсон К., Лике Д. и др. (Июнь 2006 г.). «Индукция ответа интерферона миРНК зависит от длины типа и дуплекса» . РНК . 12 (6): 988–93. doi : 10.1261/rna.2340906 . PMC 1464853 . PMID 16611941 .
- ^ Stein P, Zeng F, Pan H, Schultz RM (октябрь 2005 г.). «Отсутствие неспецифических эффектов РНК интерференции, вызванных длинной двухцепочечной РНК в ооцитах мыши» . Биология развития . 286 (2): 464–71. doi : 10.1016/j.ydbio.2005.08.015 . PMID 16154556 .
- ^ Brummelkamp TR, Bernards R , Agami R (апрель 2002 г.). «Система для стабильной экспрессии коротких мешающих РНК в клетках млекопитающих». Наука . 296 (5567): 550–3. Bibcode : 2002sci ... 296..550B . doi : 10.1126/science.1068999 . HDL : 1874/15573 . PMID 11910072 . S2CID 18460980 .
- ^ Tiscornia G, Tergaonkar V, Galimi F, Verma IM (май 2004 г.). «Индуцируемое рекомбиназой РНК-интерференция, опосредованная лентивирусными векторами» . Труды Национальной академии наук Соединенных Штатов Америки . 101 (19): 7347–51. Bibcode : 2004pnas..101.7347t . doi : 10.1073/pnas.0402107101 . PMC 409921 . PMID 15123829 .
- ^ Вентура А., Мейснер А., Диллон К.П., Макманус М., Шарп П.А., Ван Паридж Л. и др. (Июль 2004 г.). «Условное условное интерференцию РНК от трансгенов» . Труды Национальной академии наук Соединенных Штатов Америки . 101 (28): 10380–5. Bibcode : 2004pnas..10110380V . doi : 10.1073/pnas.0403954101 . PMC 478580 . PMID 15240889 .
- ^ Jump up to: а беременный Адамс Д. (5 июля 2018 г.). Полем Журнал медицины Новой Англии 379 (1): 11–2 doi : 10.1056/nejmo 1716153 HDL : 2445/138257 . 29972753PMID S2CID 205102839 .
- ^ Balwani M, Sardh E, Ventura P, Peiró PA, Rees DC, Stölzel U, et al. (11 июня 2020 г.). «Фаза 3 Исследования RNAI Therapeutic Givosiran для острого прерывистого порфирии» . Новая Англия Журнал медицины . 382 (24): 2289–2301. doi : 10.1056/nejmoa1913147 . ISSN 0028-4793 . PMID 32521132 .
- ^ SF SF, Hullon MJ, Cocha P, Al. (1 апреля 2021 г.). Полем Новый английский журнал медицины 384 (13): 1216–1 doi : 10.1056/ nejmo2 ISSN 1533-4 PMID 33789010 .
- ^ Adams D, Tournev IL, Taylor MS, Coelho T, Planté-Bordeneuve V, Berk JL, et al. (Март 2023 г.). «Эффективность и безопасность Vutrisiran для пациентов с наследственным транстиретино-опосредованным амилоидозом с полинейропатией: рандомизированное клиническое исследование» . Амилоид . 30 (1): 18–26. doi : 10.1080/13506129.2022.2091985 . HDL : 1959,4/UNSWORKS_80922 . ISSN 1744-2818 . PMID 35875890 .
- ^ «Метка: опатро-говорящая инъекция, ликерный комплекс » Daily Med 10 Там 2021.
- ^ «Пакет одобрения наркотиков: givlaari (Givosiran) инъекция» . FDA .
- ^ Gonzalez-Aseguinolaza G (11 июня 2020 года). «Givosiran - управление РНК -вмешательством для борьбы с атаками Порфирии». Новая Англия Журнал медицины . 382 (28–4793): 2366–2367. doi : 10.1056/nejme2010986 . PMID 32521139 . S2CID 219592948 .
- ^ «Гивлаари» . Европейское агентство лекарств .
- ^ Puy H, Gouya L, Deybach JC (2010). «Порфирия». Лансет . 375 (9718): 924–937. doi : 10.1016/s0140-6736 (09) 61925-5 . PMID 20226990 . S2CID 208791867 .
- ^ Simon A, Pompilus F, Querbes W, Wei A, Strzok S, Penz C, et al. (2018). «Перспектива пациента на острую прерывистую порфирию с частыми атаками: заболевание с прерывистыми и хроническими проявлениями» . Пациент . 11 (5): 527–537. doi : 10.1007/s40271-018-0319-3 . PMC 6132435 . PMID 29915990 .
- ^ Пишик Э., Кауппинен Р. (2015). «Обновление клинического лечения острого прерывистого порфирии» . Применение клинической генетики . 8 : 201–214. doi : 10.2147/tacg.s48605 . PMC 4562648 . PMID 26366103 .
- ^ Бисселл Д.М., Лай Дж.С., Мейстер Р.К., Блан П.Д. (2015). «Роль дельта-аминолевулиновой кислоты в симптомах острой порфирии» . Американский журнал медицины . 128 (3): 313–317. doi : 10.1016/j.amjmed.2014.10.026 . PMC 4339446 . PMID 25446301 .
- ^ «Метка: givlaarivisiran впрыскивание натрия, раствор» . Daily Med . 5 апреля 2022 года.
- ^ «Пакет одобрения лекарств: oxlumo» . FDA .
- ^ "Oxlumo" . Европейское агентство лекарств .
- ^ «Метка: Oxlumo-Lumasiran внедрение, раствор» . Daily Med . 2 декабря 2021 года.
- ^ SF SF, Hullon MJ, Cocha P, Al. (1 апреля 2021 г.). Полем Журнал медицины Новой Англии 384 (13): 1216–1 doi : 10.1056/ nejmo2 PMID 33789010 . S2CID 232482623 .
- ^ Исследование CF (27 ноября 2023 г.). «Снимки для испытаний наркотиков: Amvuttra» . FDA .
- ^ «Амвуттра | Европейское агентство лекарственных средств» . www.ema.europa.eu . Получено 11 января 2024 года .
- ^ Guerriaud M, Kohli E (2022). «Препараты и регулирование на основе РНК: к необходимой эволюции определений, выпущенных из законодательства Европейского Союза» . Границы в медицине . 9 doi : 10.3389/fmed.2022.1012497 . ISSN 2296-858X . PMC 9618588 . PMID 36325384 .
- ^ Jump up to: а беременный Kanasty R, Dorkin Jr, Vegas A, Anderson D (ноябрь 2013). «Материалы доставки для терапии миРНК». Природные материалы . 12 (11): 967–77. Bibcode : 2013natma..12..967k . doi : 10.1038/nmat3765 . PMID 24150415 .
- ^ Wittrup A, Lieberman J (сентябрь 2015 г.). «Обивает болезнь: отчет о прогрессе о терапии миРНК» . Nature Reviews Genetics . 16 (9): 543–52. doi : 10.1038/nrg3978 . PMC 4756474 . PMID 26281785 .
- ^ Saluzaro EA, Salueiro WG, Moro RP, TL, AL. (Там 2019). «Interperence Rena имеет результат продолжающихся элеганов » нуклеиновых кислот Исследование 47 (8): 3957–3 doi : 10.1093/nar/gkz154 . PMC 6486631 . PMID 30838421 .
- ^ Li W, Szoka FC (24 марта 2007 г.). «Наночастицы на основе липидов для доставки нуклеиновых кислот». Pharm Res . 24 (3): 438–449. doi : 10.1007/s11095-006-9180-5 . PMID 17252188 . S2CID 9995555 .
- ^ Leung AK, Tam Yy, Cullis PR (2014). «Липидные наночастицы для короткой мешающей доставки РНК». Невирусные векторы для генной терапии- перенос генов на основе липидов и полимеров . Достижения в области генетики. Тол. 88. С. 71–110. doi : 10.1016/b978-0-12-800148-6.00004-3 . ISBN 978-0-12-800148-6 Полем PMC 7149983 . PMID 25409604 .
- ^ Джон Ламаттина (15 апреля 2014 г.). «Окрашивание Big Pharma на RNAi показывает, что новые технологии не гарантируют успех в исследованиях и разработках» . Форбс .
- ^ Эрик Бендер (1 сентября 2014 г.). «Второе пришествие РНКИ» . Ученый .
- ^ Баден Лр (2021). «Эффективность и безопасность вакцины мРНК-1273 SARS-COV-2» . Новая Англия Журнал медицины . 384 (5): 403–416. doi : 10.1056/nejmoa2035389 . PMC 7787219 . PMID 33378609 .
- ^ Фицджеральд К., Уайт С., Бородовский А., Беттенкур Б.Р., Страйс А., Клаузен В. (январь 2017 г.). «Очень прочный терапевтический ингибитор РНКи PCSK9» . Новая Англия Журнал медицины . 376 (1): 41–51. doi : 10.1056/nejmoa1609243 . PMC 5778873 . PMID 27959715 .
- ^ Kruspe S, Giangrande P (2017). «Аптамер-сирна химеры: открытие, прогресс и будущие перспективы» . Биомедицины . 5 (4): 45. doi : 10.3390/Biomedicines5030045 . PMC 5618303 . PMID 28792479 .
- ^ Balwani M, Sardh E, Ventura P, Peiró PA, Rees DC, Stölzel U, et al. (11 июня 2020 г.). «Фаза 3 Исследования RNAI Therapeutic Givosiran для острого прерывистого порфирии» . Новая Англия Журнал медицины . 382 (24): 2289–2301. doi : 10.1056/nejmoa1913147 . PMID 32521132 . S2CID 219586624 .
- ^ Andersson M, Melander M, Pojmark P, Larsson H, Bülow L, Hofvander P (2006). «Целевое подавление генов с помощью РНК-интерференции: эффективный метод производства линий с высоким амилозовым картофелем» . Журнал биотехнологии . 123 (2): 137–148. doi : 10.1016/j.jbiotec.2005.11.001 . PMID 16466822 .
- ^ Фриззи А., Хуан С. (2010). «Постукивание путей молчания РНК для биотехнологии растений» . Plant Biotechnology Journal . 8 (6): 655–677. doi : 10.1111/j.1467-7652.2010.00505.x . PMID 20331529 .
- ^ Parrott W, Chassy B, Ligon J, Meyer L, Patrick J, Zhou J, et al. (2010). «Применение принципов оценки безопасности продуктов питания и корм для оценки трансгенных подходов к модуляции генов в сельскохозяйственных культурах» . Британская ассоциация промышленных биологических исследований . 48 (7): 1773–1790. doi : 10.1016/j.fct.2010.04.017 . PMID 20399824 .
- ^ Berkhout B (апрель 2004 г.). «Вмешательство РНК как антивирусное подход: нацеливание на ВИЧ-1». Современное мнение о молекулярной терапии . 6 (2): 141–5. PMID 15195925 .
- ^ Цзян М., Милнер Дж. (Сентябрь 2002 г.). «Селективное молчание экспрессии вирусных генов в HPV-позитивных клетках карциномы шейки матки человека, обработанных миРНК, праймер интерференции РНК» . Онкоген . 21 (39): 6041–8. doi : 10.1038/sj.onc.1205878 . PMID 12203116 .
- ^ Кусов Ю., Канда Т., Палменберг А., Сгро Дж.Ю., Гаусс-Мюллер В. (июнь 2006 г.). «Сильком вирусной инфекции гепатита А небольшими мешающими РНК» . Журнал вирусологии . 80 (11): 5599–610. doi : 10.1128/jvi.01773-05 . PMC 1472172 . PMID 16699041 .
- ^ Цзя Ф., Чжан Юз, Лю С.М. (октябрь 2006 г.). «Система на основе ретровируса для стабильной тишины генов вируса гепатита В путем РНК-интерференции» . Биотехнологические письма . 28 (20): 1679–85. doi : 10.1007/s10529-006-9138-z . PMID 16900331 . S2CID 34511611 .
- ^ Li YC, Kong LH, Cheng BZ, Li KS (декабрь 2005 г.). «Строительство векторов экспрессии миРНК вируса гриппа и их ингибирующее влияние на умножение вируса гриппа». Птиевые заболевания . 49 (4): 562–73. doi : 10.1637/7365-041205R2.1 . PMID 16405000 . S2CID 86214047 .
- ^ Ханна М., Саксена Л., Раджпут Р., Кумар Б., Прасад Р. (2015). «Генсинг генов: терапевтический подход к борьбе с вирусными инфекциями гриппа». Будущая микробиология . 10 (1): 131–40. doi : 10.2217/fmb.14.94 . PMID 25598342 .
- ^ Раджпут Р., Ханна М., Кумар П., Кумар Б., Шарма С., Гупта Н. и др. (Декабрь 2012 г.). «Небольшая мешающая РНК, нацеленная на транскрипт Нонтруктурного гена 1, ингибирует репликацию вируса гриппа А у экспериментальных мышей». Нуклеиновая терапия . 22 (6): 414–22. doi : 10.1089/nat.2012.0359 . PMID 23062009 .
- ^ Jump up to: а беременный в дюймовый Asha K, Kumar P, Sanicas M, Meseko CA, Khanna M, Kumar B (декабрь 2018 г.). «Достижения в терапии на основе нуклеиновых кислот против респираторных вирусных инфекций» . Журнал клинической медицины . 8 (1): 6. doi : 10.3390/jcm8010006 . PMC 6351902 . PMID 30577479 .
- ^ Hu L, Wang Z, Hu C, Liu X, Yao L, Li W, et al. (2005). «Ингибирование умножения вируса кори в клеточной культуре на РНК -интерференцию». Acta virologica . 49 (4): 227–34. PMID 16402679 .
- ^ Куреши А., Такур Н., Монга И., Такур А., Кумар М. (2014). «Virmirna: комплексный ресурс для экспериментально проверенных вирусных микроРНК и их мишеней» . База данных . 2014 . doi : 10.1093/база данных/BAU103 . PMC 4224276 . PMID 25380780 .
- ^ Кроу С. (2003). «Подавление экспрессии рецептора хемокинов с помощью РНК-интерференции позволяет ингибировать репликацию ВИЧ-1, Martínez et al.» . СПИД . 17 (Suppl 4): S103–5. doi : 10.1097/00002030-200317004-00014 . PMID 15080188 .
- ^ Fuchs U, Damm-Welk C, Borkhardt A (август 2004 г.). «Сильком генов, связанных с болезнью, небольшими мешающими РНК». Современное молекулярное лекарство . 4 (5): 507–17. doi : 10.2174/1566524043360492 . PMID 15267222 .
- ^ Cioca DP, Aoki Y, Kiyosawa K (февраль 2003 г.). «Интерференция РНК является функциональным путем с терапевтическим потенциалом в клеточных линиях миелоидного лейкоза человека» . Генная терапия рака . 10 (2): 125–33. doi : 10.1038/sj.cgt.7700544 . PMID 12536201 .
- ^ Lapteva N, Yang AG, Sanders DE, Strube RW, Chen Sy (январь 2005 г.). «Нокдаун CXCR4 с помощью небольшой мешающей РНК отменяет рост опухоли молочной железы in vivo» . Генная терапия рака . 12 (1): 84–9. doi : 10.1038/sj.cgt.7700770 . PMID 15472715 .
- ^ Jump up to: а беременный в дюймовый и фон глин час я Xu CF, Wang J (1 февраля 2015 г.). «Системы доставки для разработки лекарственных средств SIRNA при терапии рака» . Азиатский журнал фармацевтических наук . 10 (1): 1–12. doi : 10.1016/j.ajps.2014.08.011 .
- ^ Singer O, Marr RA, Rockenstein E, Crews L, Coufal Ng, Gage FH, et al. (Октябрь 2005 г.). «Нацеливание на BACE1 с миРНК ослабевает невропатологию болезни Альцгеймера в трансгенной модели». Nature Neuroscience . 8 (10): 1343–9. doi : 10.1038/nn1531 . PMID 16136043 . S2CID 6978101 .
- ^ Rodríguez-Lebron E, Gouvion CM, Moore SA, Davidson BL, Paulson HL (сентябрь 2009 г.). «Аллель-специфическая RNAi смягчает фенотипическое прогрессирование в трансгенной модели болезни Альцгеймера» . Молекулярная терапия . 17 (9): 1563–73. doi : 10.1038/mt.2009.123 . PMC 2835271 . PMID 19532137 .
- ^ Piedrahita D, Hernández I, López-Tobón A, Fedorov D, Obara B, Manjunath BS, et al. (Октябрь 2010). «Связывание CDK5 уменьшает нейрофибриллярные клубки у мышей трансгенных болезней Альцгеймера» . Журнал нейробиологии . 30 (42): 13966–76. doi : 10.1523/jneurosci.3637-10.2010 . PMC 3003593 . PMID 20962218 .
- ^ Рауль С., Баркер С.Д., Эбишер П (март 2006 г.). «Вирусное моделирование и коррекция нейродегенеративных заболеваний с помощью РНК-интерференции» . Генная терапия . 13 (6): 487–95. doi : 10.1038/sj.gt.3302690 . PMID 16319945 .
- ^ Harper SQ, Steper PD, He X, Eliason SL, Martins IH, Mao Q, et al. (Апрель 2005 г.). «РНК -интерференция улучшает моторные и невропатологические нарушения в модели мыши болезни Хантингтона» . Труды Национальной академии наук Соединенных Штатов Америки . 102 (16): 5820–5. Bibcode : 2005pnas..102.5820h . doi : 10.1073/pnas.0501507102 . PMC 556303 . PMID 15811941 .
- ^ Будро Р.Л., Родригес-Леброн Э., Дэвидсон Б.Л. (апрель 2011 г.). «Медицина RNAi для мозга: прогрессирует и вызовы» . Молекулярная генетика человека . 20 (R1): R21–7. doi : 10.1093/hmg/ddr137 . PMC 3095054 . PMID 21459775 .
- ^ Jump up to: а беременный в дюймовый и фон глин час я Дж k л м Уайтхед К.А., Далман Дж., Лангер Р.С., Андерсон Д.Г. (2011). «Сильком или стимуляция? Доставка миРНК и иммунная система». Ежегодный обзор химической и биомолекулярной инженерии . 2 : 77–96. doi : 10.1146/annurev-chembioeng-061010-114133 . PMID 22432611 . S2CID 28803811 .
- ^ Sunilkumar G, Campbell LM, Puckhaber L, Stypanovic Rd, Rathore KS (ноябрь 2006 г.). «Инженерное хлопок для использования в питании человека путем тканевого специфического восстановления токсичного госсипола» . Труды Национальной академии наук Соединенных Штатов Америки . 103 (48): 18054–9. doi : 10.1073/pnas.0605389103 . PMC 1838705 . PMID 17110445 .
- ^ Le LQ, Lorenz Y, Scheurer S, Fötisch K, Enrique E, Bartra J, et al. (Март 2006 г.). «Дизайн томатных фруктов с уменьшенной аллергенности путем DSRNAi-опосредованной ингибирования экспрессии NS-LTP (LYC E 3)» . Plant Biotechnology Journal . 4 (2): 231–42. doi : 10.1111/j.1467-7652.2005.00175.x . PMID 17177799 .
- ^ Niggeweg R, Michael AJ, Martin C (июнь 2004 г.). «Инженерные установки с повышенным уровнем антиоксидантной хлорогеновой кислоты». Nature Biotechnology . 22 (6): 746–54. doi : 10.1038/nbt966 . PMID 15107863 . S2CID 21588259 .
- ^ Гилберт М.К., Маджамдар Р., Раджасекаран К., Чен Зи, Вэй К., Сиплер С.М. и др. (Июнь 2018 г.). «РНК-интерференционное молчание гена альфа-амилазы (AMY1) у Aspergillus flavus снижает рост грибов и выработку афлатоксина в ядрах кукурузы». Планта 247 (6): 1465–1473. Bibcode : 2018plant.247.1465g . doi : 10.1007/s00425-018-2875-0 . PMID 29541880 . S2CID 3918937 .
- ^ Katoch R, Thakur N (март 2013 г.). «Вмешательство РНК: многообещающая техника улучшения традиционных культур». Международный журнал пищевых наук и питания . 64 (2): 248–59. doi : 10.3109/09637486.2012.713918 . PMID 22861122 . S2CID 45212581 .
- ^ Katoch R, Thakur N (март 2013 г.). «Достижения в области технологии интерференции РНК и ее влияния на улучшение питания, заболевание и контроль насекомых у растений». Прикладная биохимия и биотехнология . 169 (5): 1579–605. doi : 10.1007/s12010-012-0046-5 . PMID 23322250 . S2CID 23733295 .
- ^ Jump up to: а беременный Дауни Р.К., Лин М., Корси Б., Фику А., Лиллемо М., Оливер Р.П. и др. (27 июля 2021 г.). «Septoria nodorum пятно пшеницы: лечение заболеваний и размножение устойчивости перед лицом смещения динамики заболеваний и меняющейся среды». Фитопатология . 111 (6). Американское фитопатологическое общество : фито - 07–20–028. doi : 10.1094/phyto-07-20-0280-rvw . HDL : 20.500.11937/83208 . ISSN 0031-949X . PMID 33245254 . S2CID 227181536 .
- ^ Ivashuta S, Zhang Y, Wiggins BE, Ramaseshadri P, Segers GC, Johnson S, et al. (Май 2015). «Экологическая РНКи у травоядных насекомых» . РНК . 21 (5): 840–50. doi : 10.1261/rna.048116.114 . PMC 4408792 . PMID 25802407 .
- ^ Miller SC, Miyata K, Brown SJ, Tomoyasu Y (2012). «Расширение системных интерференций РНК в The Castaneum Tribolium Tribolium Tribolium : параметры, влияющие на эффективность RNAi» . Plos один . 7 (10): E47431. Bibcode : 2012ploso ... 747431M . doi : 10.1371/journal.pone.0047431 . PMC 3484993 . PMID 23133513 .
- ^ Petrick JS, Friedrich GE, Carleton SM, Kessenich CR, Silvanovich A, Zhang Y, et al. (Ноябрь 2016). «Корневой червя, активный РНК DVSNF7: Повторная доза пероральная токсикологическая оценка в поддержку безопасности человека и млекопитающих» . Регуляторная токсикология и фармакология . 81 : 57–68. doi : 10.1016/j.yrtph.2016.07.009 . PMID 27436086 .
- ^ Terenius O, Papanicolaou A, Garbutt JS, Eleftherianos I, Huvenne H, Kanginakudru S, et al. (Февраль 2011 г.). «Вмешательство РНК в Lepidoptera: обзор успешных и неудачных исследований и последствий для экспериментального дизайна» . Журнал физиологии насекомых . 57 (2): 231–45. Bibcode : 2011jinsp..57..231t . doi : 10.1016/j.jinsphys.2010.11.006 . HDL : 1854/LU-11101411 . PMID 21078327 .
- ^ Mongelli V, Saleh MC (29 сентября 2016 г.). «Ошибки не должны молчать: небольшие пути РНК и противовирусные реакции у насекомых» (PDF) . Ежегодный обзор вирусологии . 3 (1). Ежегодные обзоры : 573–589. doi : 10.1146/annurev-virology-1110615-042447 . ISSN 2327-056X . PMID 27741406 . S2CID 38499958 .
- ^ Jump up to: а беременный в Чжу К.Ю., Палли -С.Р. (7 января 2020 года). «Механизмы, приложения и проблемы РНК -интерференции насекомых» . Ежегодный обзор энтомологии . 65 (1). Ежегодные обзоры : 293–311. doi : 10.1146/annurev-ento-011019-025224 . ISSN 0066-4170 . PMC 9939233 . PMID 31610134 . S2CID 204702574 .
- ^ Huesken D, Lange J, Mickanin C, Weiler J, Asselbergs F, Warner J, et al. (Август 2005 г.). «Дизайн библиотеки миРНК в геноме с использованием искусственной нейронной сети». Nature Biotechnology . 23 (8): 995–1001. doi : 10.1038/nbt1118 . PMID 16025102 . S2CID 11030533 .
- ^ Ge G, Wong GW, Luo B (октябрь 2005 г.). «Прогнозирование эффективности нокдауна siRNA с использованием моделей искусственной нейронной сети». Биохимическая и биофизическая исследовательская коммуникация . 336 (2): 723–8. doi : 10.1016/j.bbrc.2005.08.147 . PMID 16153609 .
- ^ Джаниц М., Ванке Д., Лерач Х. (2006). «Высокопроизводительные интерференции РНК в функциональную геномику». РНК к медицину . Справочник по экспериментальной фармакологии. Тол. 173. С. 97–104. doi : 10.1007/3-540-27262-3_5 . ISBN 978-3-540-27261-8 Полем PMID 16594612 .
- ^ Vanhecke D, Janitz M (февраль 2005 г.). «Функциональная геномика с использованием высокопроизводительных интерференций РНК» . Drug Discovery сегодня . 10 (3): 205–12. doi : 10.1016/s1359-6446 (04) 03352-5 . HDL : 11858/00-001M-0000-0010-86E7-8 . PMID 15708535 . S2CID 9934291 .
- ^ Мэтсон Р.С. (2005). Применение технологии геномного и протеомного микрочипа при обнаружении лекарств . CRC Press. п. 6 ISBN 978-0-8493-1469-8 .
- ^ Чжан XHD (2011). Оптимальный высокопроизводительный скрининг: практический экспериментальный дизайн и анализ данных для исследования RNAI в масштабе генома . Издательство Кембриджского университета. с. IX - XIII. ISBN 978-0-521-73444-8 .
- ^ Мацке М.А., Мацке А.Дж. (2004). «Посадка семян новой парадигмы» . PLOS BIOL . 2 (5): E133. doi : 10.1371/journal.pbio.0020133 . PMC 406394 . PMID 15138502 .
- ^ Экер -младший, Дэвис Р.В. (август 1986 г.). «Ингибирование экспрессии генов в клетках растений путем экспрессии антисмысловой РНК» . Труды Национальной академии наук Соединенных Штатов Америки . 83 (15): 5372–6. Bibcode : 1986pnas ... 83,5372E . doi : 10.1073/pnas.83.15.5372 . PMC 386288 . PMID 16593734 .
- ^ Наполи С., Лемье С., Йоргенсен Р. (апрель 1990 г.). «Введение гена химерного синтазы халкона в петунии приводит к обратимой совместном положении гомологичных генов в транс» . Растительная ячейка . 2 (4): 279–289. doi : 10.1105/tpc.2.4.279 . PMC 159885 . PMID 12354959 .
- ^ Романо Н., Макино Дж (ноябрь 1992). «Окружение: временная инактивация экспрессии генов в нейроспоре Crassa путем трансформации с гомологичными последовательностями». Молекулярная микробиология . 6 (22): 3343–53. doi : 10.1111/j.1365-2958.1992.tb02202.x . PMID 1484489 . S2CID 31234985 .
- ^ Van Blokland R, Van Der Geest N, Mol JN, Koter JM (1994). «Трансгеновая опосредованная подавление экспрессии халконасинтазы в Petunia Hybrida является результатом увеличения оборота РНК» . Растение J. 6 (6): 861–77. doi : 10.1046/j.1365-313X.1994.6060861.x .
- ^ Mol Jn, Van der Krol AR (1991). Антисмысловые нуклеиновые кислоты и белки: основы и применения . М. Деккер. с. 4, 136. ISBN 978-0-8247-8516-1 .
- ^ Кови С., Аль-Кафф Н., Лайнгара А., Тернер Д. (1997). «Растения борьбы с инфекцией путем молчания генов». Природа . 385 (6619): 781–2. Bibcode : 1997natur.385..781c . doi : 10.1038/385781a0 . S2CID 43229760 .
- ^ Kumagai MH, Donson J, Della-Cioppa G, Harvey D, Hanley K, Grill LK (февраль 1995 г.). «Цитоплазматическое ингибирование биосинтеза каротиноидов с РНК, полученной из вируса» . Труды Национальной академии наук Соединенных Штатов Америки . 92 (5): 1679–83. Bibcode : 1995pnas ... 92.1679K . doi : 10.1073/pnas.92.5.1679 . PMC 42583 . PMID 7878039 .
- ^ Ratcliff F, Harrison BD, Baulcombe DC (июнь 1997 г.). «Сходство между вирусной защитой и молчанием генов у растений». Наука . 276 (5318): 1558–60. doi : 10.1126/science.276.5318.1558 . PMID 18610513 .
- ^ Guo S, Kemphues KJ (май 1995). «Par-1, ген, необходимый для установления полярности у эмбрионов C. elegans, кодирует предполагаемую киназу Ser/thr, которая асимметрично распределена» . Клетка . 81 (4): 611–20. doi : 10.1016/0092-8674 (95) 90082-9 . PMID 7758115 .
- ^ Pal-Bhadra M, Bhadra U, Birchler JA (август 1997 г.). «Космосенсия у дрозофилы: молчание генов алкогольной дегидрогеназы трансгенами белого ADH зависит от поликомба» . Клетка . 90 (3): 479–90. doi : 10.1016/s0092-8674 (00) 80508-5 . PMID 9267028 .
- ^ Jump up to: а беременный Sen GL, Blau HM (июль 2006 г.). «Краткая история РНКИ: молчание генов» . FASEB Journal . 20 (9): 1293–9. doi : 10.1096/fj.06-6014rev . PMID 16816104 . S2CID 12917676 .
- ^ Fire A, Xu S, Montgomery MK, Kostas SA, Driver SE, Mello CC (февраль 1998 г.). «Мощное и специфическое генетическое помехи с помощью двухцепочечного РНК у Caenorhabditis elegans» . Природа . 391 (6669): 806–11. Bibcode : 1998natur.391..806f . doi : 10.1038/35888 . PMID 9486653 . S2CID 4355692 .
- ^ Daneholt B (2 октября 2006 г.). «Нобелевская премия по физиологии или медицине 2006» . nobelprize.org . Получено 30 октября 2017 года .
- ^ Elbashir S, Harborth J, Lendeckel W, et al. (2001). «Дуплексы 21-нуклеотидных РНК опосредуют интерференцию РНК в культивируемых клетках млекопитающих». Природа . 411 (6836): 494–498. Bibcode : 2001natur.411..494e . doi : 10.1038/35078107 . PMID 11373684 . S2CID 710341 .
- ^ McCaffrey AP, Meuse L, Pham TT, Conklin DS, Hannon GJ , Kay Ma (июль 2002 г.). «Вмешательство РНК у взрослых мышей». Природа . 418 (6893): 38–9. Bibcode : 2002natur.418 ... 38M . doi : 10.1038/418038a . PMID 12097900 . S2CID 4361399 .
- ^ Деви Гр (сентябрь 2006 г.). «Подходы на основе миРНК в терапии рака» . Генная терапия рака . 13 (9): 819–29. doi : 10.1038/sj.cgt.7700931 . PMID 16424918 .
- ^ Стена Н.Р., Ши (октябрь 2003 г.). «Маленькая РНК: можно ли использовать интерференцию РНК для терапии?». Лансет . 362 (9393): 1401–3. doi : 10.1016/s0140-6736 (03) 14637-5 . PMID 14585643 . S2CID 25034627 .
- ^ SAH D (2006). «Терапевтический потенциал РНК -интерференции на неврологические расстройства». Life Sci . 79 (19): 1773–80. doi : 10.1016/j.lfs.2006.06.011 . PMID 16815477 .
- ^ Davis ME , Zuckerman JE, Choi CH, Seligson D, Tolcher A, Alabi CA , et al. (Апрель 2010). «Свидетельство РНКи у людей от системно вводимой миРНК через целевые наночастицы» . Природа . 464 (7291): 1067–70. Bibcode : 2010natur.464.1067d . doi : 10.1038/nature08956 . PMC 2855406 . PMID 20305636 .
- ^ «Novartis получает одобрение ЕС для Leqvio (Inclisiran), первой в классе миРНК для более низкого уровня холестерина с двумя дозами в год» . Novartis . 11 декабря 2020 года.
Внешние ссылки
[ редактировать ]

- Обзор процесса RNAI , от Кембриджского университета «Обнаженные ученые»
- Анимация процесса RNAi , от природы
- Nova Sciencenow объясняет RNAI -15-минутное видео о трансляции Nova , которое транслировалось на PBS , 26 июля 2005 г.
- Геномы молчания архивировали 10 августа 2019 года в экспериментах по интерференции RNA Machine Machine (RNAI) и биоинформатике в C. elegans для образования. Из Центра обучения ДНК Долана в лаборатории Холодного Спринг -Харбора.
- Экраны RNAI в C. elegans в 96-луночном жидком формате и их применение к систематической идентификации генетических взаимодействий (протокол)
- 2 американских «червя» выигрывают Нобелевскую работу за РНК , от Нью -Йорка Таймс
- молекулярной терапии Степень .
- Genomernai : база данных фенотипов из экспериментов по скринингу РНК в Drosophila melanogaster и Homo sapiens
- RNAI Архивировали 19 июня 2018 года в The Wayback Machine Инструменты