Глобальная система позиционирования
![]() Логотип | |
Страна/страны происхождения | Соединенные Штаты |
---|---|
Operator(s) | US Space Force |
Type | Military, civilian |
Status | Operational |
Coverage | Global |
Accuracy | 30–500 cm (0.98–16 ft) |
Constellation size | |
Nominal satellites | 24 |
Current usable satellites | 38 (32 operational) |
First launch | February 22, 1978 |
Total launches | 75 |
Orbital characteristics | |
Regime(s) | 6 MEO planes |
Orbital height | 20,180 km (12,540 mi) |
Orbital period | 1⁄2 sd or 11 hours and 58 minutes |
Revisit period | 1 sidereal day |
Other details | |
Cost | $12 billion[1] (initial constellation) $1.84 billion per year (2023)[1] (operating cost) |
Website | gps.gov |
Geodesy |
---|
![]() |
Система глобального позиционирования ( GPS ), первоначально Navstar GPS , [2] — спутниковая радионавигационная система, принадлежащая правительству США и эксплуатируемая Космическими силами США . [3] Это одна из глобальных навигационных спутниковых систем (GNSS), которая предоставляет о геолокации и информацию в времени GPS-приемнику любой точке Земли или вблизи нее, где есть беспрепятственная прямая видимость для четырех или более спутников GPS. [4] Он не требует от пользователя передачи каких-либо данных и работает независимо от приема телефона или Интернета, хотя эти технологии могут повысить полезность информации о местоположении GPS. Он предоставляет возможности критического позиционирования военным, гражданским и коммерческим пользователям по всему миру. Хотя правительство Соединенных Штатов создало, контролирует и обслуживает систему GPS, к ней свободно доступен каждый, у кого есть GPS-приемник. [5]
Overview
[edit]The GPS project was started by the U.S. Department of Defense in 1973. The first prototype spacecraft was launched in 1978 and the full constellation of 24 satellites became operational in 1993.
After Korean Air Lines Flight 007 was shot down when it mistakenly entered Soviet airspace, President Ronald Reagan announced that the GPS system would be made available for civilian use as of September 16, 1983;[6] however, initially this civilian use was limited to an average accuracy of 100 meters (330 ft) by use of Selective Availability (SA), a deliberate error introduced into the GPS data (which military receivers could correct for).
As civilian GPS usage grew, there was increasing pressure to remove this error. The SA system was temporarily disabled during the Gulf War, as a shortage of military GPS units meant that many US soldiers were using civilian GPS units sent from home. In the 1990s, Differential GPS systems from the US Coast Guard, Federal Aviation Administration, and similar agencies in other countries began to broadcast local GPS corrections, reducing the effect of both SA degradation and atmospheric effects (that military receivers also corrected for). The US military had also developed methods to perform local GPS jamming, meaning that the ability to globally degrade the system was no longer necessary. As a result, President Bill Clinton signed a bill ordering that Selective Availability be disabled on May 1 2000;[7] and, in 2007, the US government announced that the next generation of GPS satellites would not include the feature at all.
Advances in technology and new demands on the existing system have now led to efforts to modernize the GPS and implement the next generation of GPS Block III satellites and Next Generation Operational Control System (OCX)[8] which was authorized by the U.S. Congress in 2000. When Selective Availability was discontinued, GPS was accurate to about 5 meters (16 ft). GPS receivers that use the L5 band have much higher accuracy of 30 centimeters (12 in), while those for high-end applications such as engineering and land surveying are accurate to within 2 cm (3⁄4 in) and can even provide sub-millimeter accuracy with long-term measurements.[7][9][10] Consumer devices such as smartphones can be accurate to 4.9 m (16 ft) or better when used with assistive services like Wi-Fi positioning.[11]
As of July 2023[update], 18 GPS satellites broadcast L5 signals, which are considered pre-operational prior to being broadcast by a full complement of 24 satellites in 2027.[12]
History
[edit]
The GPS project was launched in the United States in 1973 to overcome the limitations of previous navigation systems,[13] combining ideas from several predecessors, including classified engineering design studies from the 1960s. The U.S. Department of Defense developed the system, which originally used 24 satellites, for use by the United States military, and became fully operational in 1993. Civilian use was allowed from the 1980s. Roger L. Easton of the Naval Research Laboratory, Ivan A. Getting of The Aerospace Corporation, and Bradford Parkinson of the Applied Physics Laboratory are credited with inventing it.[14] The work of Gladys West on the creation of the mathematical geodetic Earth model is credited as instrumental in the development of computational techniques for detecting satellite positions with the precision needed for GPS.[15][16]
The design of GPS is based partly on similar ground-based radio-navigation systems, such as LORAN and the Decca Navigator, developed in the early 1940s.
In 1955, Friedwardt Winterberg proposed a test of general relativity—detecting time slowing in a strong gravitational field using accurate atomic clocks placed in orbit inside artificial satellites. Special and general relativity predicted that the clocks on GPS satellites, as observed by those on Earth, run 38 microseconds faster per day than those on the Earth. The design of GPS corrects for this difference; because without doing so, GPS calculated positions would accumulate errors of up to 10 kilometers per day (6 mi/d).[17]
Predecessors
[edit]When the Soviet Union launched its first artificial satellite (Sputnik 1) in 1957, two American physicists, William Guier and George Weiffenbach, at Johns Hopkins University's Applied Physics Laboratory (APL) decided to monitor its radio transmissions.[18] Within hours they realized that, because of the Doppler effect, they could pinpoint where the satellite was along its orbit. The Director of the APL gave them access to their UNIVAC to do the heavy calculations required.

Early the next year, Frank McClure, the deputy director of the APL, asked Guier and Weiffenbach to investigate the inverse problem: pinpointing the user's location, given the satellite's. (At the time, the Navy was developing the submarine-launched Polaris missile, which required them to know the submarine's location.) This led them and APL to develop the TRANSIT system.[19] In 1959, ARPA (renamed DARPA in 1972) also played a role in TRANSIT.[20][21][22]
TRANSIT was first successfully tested in 1960.[23] It used a constellation of five satellites and could provide a navigational fix approximately once per hour.
In 1967, the U.S. Navy developed the Timation satellite, which proved the feasibility of placing accurate clocks in space, a technology required for GPS.
In the 1970s, the ground-based OMEGA navigation system, based on phase comparison of signal transmission from pairs of stations,[24] became the first worldwide radio navigation system. Limitations of these systems drove the need for a more universal navigation solution with greater accuracy.
Although there were wide needs for accurate navigation in military and civilian sectors, almost none of those was seen as justification for the billions of dollars it would cost in research, development, deployment, and operation of a constellation of navigation satellites. During the Cold War arms race, the nuclear threat to the existence of the United States was the one need that did justify this cost in the view of the United States Congress. This deterrent effect is why GPS was funded. It is also the reason for the ultra-secrecy at that time. The nuclear triad consisted of the United States Navy's submarine-launched ballistic missiles (SLBMs) along with United States Air Force (USAF) strategic bombers and intercontinental ballistic missiles (ICBMs). Considered vital to the nuclear deterrence posture, accurate determination of the SLBM launch position was a force multiplier.
Precise navigation would enable United States ballistic missile submarines to get an accurate fix of their positions before they launched their SLBMs.[25] The USAF, with two thirds of the nuclear triad, also had requirements for a more accurate and reliable navigation system. The U.S. Navy and U.S. Air Force were developing their own technologies in parallel to solve what was essentially the same problem.
To increase the survivability of ICBMs, there was a proposal to use mobile launch platforms (comparable to the Soviet SS-24 and SS-25) and so the need to fix the launch position had similarity to the SLBM situation.
In 1960, the Air Force proposed a radio-navigation system called MOSAIC (MObile System for Accurate ICBM Control) that was essentially a 3-D LORAN. A follow-on study, Project 57, was performed in 1963 and it was "in this study that the GPS concept was born". That same year, the concept was pursued as Project 621B, which had "many of the attributes that you now see in GPS"[26] and promised increased accuracy for Air Force bombers as well as ICBMs.

Updates from the Navy TRANSIT system were too slow for the high speeds of Air Force operation. The Naval Research Laboratory (NRL) continued making advances with their Timation (Time Navigation) satellites, first launched in 1967, second launched in 1969, with the third in 1974 carrying the first atomic clock into orbit and the fourth launched in 1977.[27]
Another important predecessor to GPS came from a different branch of the United States military. In 1964, the United States Army orbited its first Sequential Collation of Range (SECOR) satellite used for geodetic surveying.[28] The SECOR system included three ground-based transmitters at known locations that would send signals to the satellite transponder in orbit. A fourth ground-based station, at an undetermined position, could then use those signals to fix its location precisely. The last SECOR satellite was launched in 1969.[29]
Development
[edit]With these parallel developments in the 1960s, it was realized that a superior system could be developed by synthesizing the best technologies from 621B, Transit, Timation, and SECOR in a multi-service program. Satellite orbital position errors, induced by variations in the gravity field and radar refraction among others, had to be resolved. A team led by Harold L Jury of Pan Am Aerospace Division in Florida from 1970 to 1973, used real-time data assimilation and recursive estimation to do so, reducing systematic and residual errors to a manageable level to permit accurate navigation.[30]
During Labor Day weekend in 1973, a meeting of about twelve military officers at the Pentagon discussed the creation of a Defense Navigation Satellite System (DNSS). It was at this meeting that the real synthesis that became GPS was created. Later that year, the DNSS program was named Navstar.[31] Navstar is often erroneously considered an acronym for "NAVigation System using Timing And Ranging" but was never considered as such by the GPS Joint Program Office (TRW may have once advocated for a different navigational system that used that acronym).[32] With the individual satellites being associated with the name Navstar (as with the predecessors Transit and Timation), a more fully encompassing name was used to identify the constellation of Navstar satellites, Navstar-GPS.[33] Ten "Block I" prototype satellites were launched between 1978 and 1985 (an additional unit was destroyed in a launch failure).[34]
The effect of the ionosphere on radio transmission was investigated in a geophysics laboratory of Air Force Cambridge Research Laboratory, renamed to Air Force Geophysical Research Lab (AFGRL) in 1974. AFGRL developed the Klobuchar model for computing ionospheric corrections to GPS location.[35] Of note is work done by Australian space scientist Elizabeth Essex-Cohen at AFGRL in 1974. She was concerned with the curving of the paths of radio waves (atmospheric refraction) traversing the ionosphere from NavSTAR satellites.[36]
After Korean Air Lines Flight 007, a Boeing 747 carrying 269 people, was shot down by a Soviet interceptor aircraft after straying in prohibited airspace because of navigational errors,[37] in the vicinity of Sakhalin and Moneron Islands, President Ronald Reagan issued a directive making GPS freely available for civilian use, once it was sufficiently developed, as a common good.[38] The first Block II satellite was launched on February 14, 1989,[39] and the 24th satellite was launched in 1994. The GPS program cost at this point, not including the cost of the user equipment but including the costs of the satellite launches, has been estimated at US$5 billion (equivalent to $10 billion in 2023).[40]
Initially, the highest-quality signal was reserved for military use, and the signal available for civilian use was intentionally degraded, in a policy known as Selective Availability. This changed on May 1, 2000, with President Bill Clinton signing a policy directive to turn off Selective Availability to provide the same accuracy to civilians that was afforded to the military. The directive was proposed by the U.S. Secretary of Defense, William Perry, in view of the widespread growth of differential GPS services by private industry to improve civilian accuracy. Moreover, the U.S. military was developing technologies to deny GPS service to potential adversaries on a regional basis.[41] Selective Availability was removed from the GPS architecture beginning with GPS-III.
Since its deployment, the U.S. has implemented several improvements to the GPS service, including new signals for civil use and increased accuracy and integrity for all users, all the while maintaining compatibility with existing GPS equipment. Modernization of the satellite system has been an ongoing initiative by the U.S. Department of Defense through a series of satellite acquisitions to meet the growing needs of the military, civilians, and the commercial market.
As of early 2015, high-quality Standard Positioning Service (SPS) GPS receivers provided horizontal accuracy of better than 3.5 meters (11 ft),[7] although many factors such as receiver and antenna quality and atmospheric issues can affect this accuracy.
GPS is owned and operated by the United States government as a national resource. The Department of Defense is the steward of GPS. The Interagency GPS Executive Board (IGEB) oversaw GPS policy matters from 1996 to 2004. After that, the National Space-Based Positioning, Navigation and Timing Executive Committee was established by presidential directive in 2004 to advise and coordinate federal departments and agencies on matters concerning the GPS and related systems.[42] The executive committee is chaired jointly by the Deputy Secretaries of Defense and Transportation. Its membership includes equivalent-level officials from the Departments of State, Commerce, and Homeland Security, the Joint Chiefs of Staff and NASA. Components of the executive office of the president participate as observers to the executive committee, and the FCC chairman participates as a liaison.
The U.S. Department of Defense is required by law to "maintain a Standard Positioning Service (as defined in the federal radio navigation plan and the standard positioning service signal specification) that will be available on a continuous, worldwide basis" and "develop measures to prevent hostile use of GPS and its augmentations without unduly disrupting or degrading civilian uses".
Timeline and modernization
[edit]![]() |
Block | Launch period | Satellite launches | Currently in orbit and healthy | |||
---|---|---|---|---|---|---|
Success | Failure | In preparation | Planned | |||
I | 1978–1985 | 10 | 1 | 0 | 0 | 0 |
II | 1989–1990 | 9 | 0 | 0 | 0 | 0 |
IIA | 1990–1997 | 19 | 0 | 0 | 0 | 0 |
IIR | 1997–2004 | 12 | 1 | 0 | 0 | 7 |
IIR-M | 2005–2009 | 8 | 0 | 0 | 0 | 7 |
IIF | 2010–2016 | 12 | 0 | 0 | 0 | 12 |
IIIA | 2018– | 6 | 0 | 4 | 0 | 6 |
IIIF | — | 0 | 0 | 0 | 22 | 0 |
Total | 76 | 2 | 4 | 22 | 32 | |
(Last update: September 3, 2023) USA-203 from Block IIR-M is unhealthy |
- In 1972, the USAF Central Inertial Guidance Test Facility (Holloman AFB) conducted developmental flight tests of four prototype GPS receivers in a Y configuration over White Sands Missile Range, using ground-based pseudo-satellites.[47]
- In 1978, the first experimental Block-I GPS satellite was launched.[34]
- In 1983, after Soviet interceptor aircraft shot down the civilian airliner KAL 007 that strayed into prohibited airspace because of navigational errors, killing all 269 people on board, U.S. President Ronald Reagan announced that GPS would be made available for civilian uses once it was completed,[48][49] although it had been publicly known as early as 1979, that the CA code (Coarse/Acquisition code) would be available to civilian users.[50][51]
- By 1985, ten more experimental Block-I satellites had been launched to validate the concept.
- Beginning in 1988, command and control of these satellites was moved from Onizuka AFS, California to the 2nd Satellite Control Squadron (2SCS) located at Falcon Air Force Station in Colorado Springs, Colorado.[52][53]
- On February 14, 1989, the first modern Block-II satellite was launched.
- The Gulf War from 1990 to 1991 was the first conflict in which the military widely used GPS.[54]
- In 1991, DARPA's project to create a miniature GPS receiver successfully ended, replacing the previous 16 kg (35 lb) military receivers with a 1.25 kg (2.8 lb) all-digital handheld GPS receiver.[21]
- In 1991, TomTom, a Dutch sat-nav manufacturer was founded.
- In 1992, the 2nd Space Wing, which originally managed the system, was inactivated and replaced by the 50th Space Wing.
Emblem of the 50th Space Wing - By December 1993, GPS achieved initial operational capability (IOC), with a full constellation (24 satellites) available and providing the Standard Positioning Service (SPS).[55]
- Full Operational Capability (FOC) was declared by Air Force Space Command (AFSPC) in April 1995, signifying full availability of the military's secure Precise Positioning Service (PPS).[55]
- In 1996, recognizing the importance of GPS to civilian users as well as military users, U.S. President Bill Clinton issued a policy directive[56] declaring GPS a dual-use system and establishing an Interagency GPS Executive Board to manage it as a national asset.
- In 1998, United States Vice President Al Gore announced plans to upgrade GPS with two new civilian signals for enhanced user accuracy and reliability, particularly with respect to aviation safety, and in 2000 the United States Congress authorized the effort, referring to it as GPS III.
- On May 2, 2000 "Selective Availability" was discontinued as a result of the 1996 executive order, allowing civilian users to receive a non-degraded signal globally.
- In 2004, the United States government signed an agreement with the European Community establishing cooperation related to GPS and Europe's Galileo system.
- In 2004, United States President George W. Bush updated the national policy and replaced the executive board with the National Executive Committee for Space-Based Positioning, Navigation, and Timing.[57]
- November 2004, Qualcomm announced successful tests of assisted GPS for mobile phones.[58]
- In 2005, the first modernized GPS satellite was launched and began transmitting a second civilian signal (L2C) for enhanced user performance.[59]
- On September 14, 2007, the aging mainframe-based Ground Segment Control System was transferred to the new Architecture Evolution Plan.[60]
- On May 19, 2009, the United States Government Accountability Office issued a report warning that some GPS satellites could fail as soon as 2010.[61]
- On May 21, 2009, the Air Force Space Command allayed fears of GPS failure, saying: "There's only a small risk we will not continue to exceed our performance standard."[62]
- On January 11, 2010, an update of ground control systems caused a software incompatibility with 8,000 to 10,000 military receivers manufactured by a division of Trimble Navigation Limited of Sunnyvale, Calif.[clarification needed][63]
- On February 25, 2010,[64] the U.S. Air Force awarded the contract to Raytheon Company to develop the GPS Next Generation Operational Control System (OCX) to improve accuracy and availability of GPS navigation signals, and serve as a critical part of GPS modernization.
Awards
[edit]
On February 10, 1993, the National Aeronautic Association selected the GPS Team as winners of the 1992 Robert J. Collier Trophy, the US's most prestigious aviation award. This team combines researchers from the Naval Research Laboratory, the USAF, the Aerospace Corporation, Rockwell International Corporation, and IBM Federal Systems Company. The citation honors them "for the most significant development for safe and efficient navigation and surveillance of air and spacecraft since the introduction of radio navigation 50 years ago".
Two GPS developers received the National Academy of Engineering Charles Stark Draper Prize for 2003:
- Ivan Getting, emeritus president of The Aerospace Corporation and an engineer at MIT, established the basis for GPS, improving on the World War II land-based radio system called LORAN (Long-range Radio Aid to Navigation).
- Bradford Parkinson, professor of aeronautics and astronautics at Stanford University, conceived the present satellite-based system in the early 1960s and developed it in conjunction with the U.S. Air Force. Parkinson served twenty-one years in the Air Force, from 1957 to 1978, and retired with the rank of colonel.
GPS developer Roger L. Easton received the National Medal of Technology on February 13, 2006.[65]
Francis X. Kane (Col. USAF, ret.) was inducted into the U.S. Air Force Space and Missile Pioneers Hall of Fame at Lackland A.F.B., San Antonio, Texas, March 2, 2010, for his role in space technology development and the engineering design concept of GPS conducted as part of Project 621B.
In 1998, GPS technology was inducted into the Space Foundation Space Technology Hall of Fame.[66]
On October 4, 2011, the International Astronautical Federation (IAF) awarded the Global Positioning System (GPS) its 60th Anniversary Award, nominated by IAF member, the American Institute for Aeronautics and Astronautics (AIAA). The IAF Honors and Awards Committee recognized the uniqueness of the GPS program and the exemplary role it has played in building international collaboration for the benefit of humanity.[67]
On December 6, 2018, Gladys West was inducted into the Air Force Space and Missile Pioneers Hall of Fame in recognition of her work on an extremely accurate geodetic Earth model, which was ultimately used to determine the orbit of the GPS constellation.[68]
On February 12, 2019, four founding members of the project were awarded the Queen Elizabeth Prize for Engineering with the chair of the awarding board stating: "Engineering is the foundation of civilisation; there is no other foundation; it makes things happen. And that's exactly what today's Laureates have done – they've made things happen. They've re-written, in a major way, the infrastructure of our world."[69]
Principles
[edit]This section needs additional citations for verification. (March 2015) |
The GPS satellites carry very stable atomic clocks that are synchronized with one another and with the reference atomic clocks at the ground control stations; any drift of the clocks aboard the satellites from the reference time maintained on the ground stations is corrected regularly.[70] Since the speed of radio waves (speed of light)[71] is constant and independent of the satellite speed, the time delay between when the satellite transmits a signal and the ground station receives it is proportional to the distance from the satellite to the ground station. With the distance information collected from multiple ground stations, the location coordinates of any satellite at any time can be calculated with great precision.
Each GPS satellite carries an accurate record of its own position and time, and broadcasts that data continuously. Based on data received from multiple GPS satellites, an end user's GPS receiver can calculate its own four-dimensional position in spacetime; However, at a minimum, four satellites must be in view of the receiver for it to compute four unknown quantities (three position coordinates and the deviation of its own clock from satellite time).[72]
More detailed description
[edit]Each GPS satellite continually broadcasts a signal (carrier wave with modulation) that includes:
- A pseudorandom code (sequence of ones and zeros) that is known to the receiver. By time-aligning a receiver-generated version and the receiver-measured version of the code, the time of arrival (TOA) of a defined point in the code sequence, called an epoch, can be found in the receiver clock time scale
- A message that includes the time of transmission (TOT) of the code epoch (in GPS time scale) and the satellite position at that time
Conceptually, the receiver measures the TOAs (according to its own clock) of four satellite signals. From the TOAs and the TOTs, the receiver forms four time of flight (TOF) values, which are (given the speed of light) approximately equivalent to receiver-satellite ranges plus time difference between the receiver and GPS satellites multiplied by speed of light, which are called pseudo-ranges. The receiver then computes its three-dimensional position and clock deviation from the four TOFs.
In practice the receiver position (in three dimensional Cartesian coordinates with origin at the Earth's center) and the offset of the receiver clock relative to the GPS time are computed simultaneously, using the navigation equations to process the TOFs.
The receiver's Earth-centered solution location is usually converted to latitude, longitude and height relative to an ellipsoidal Earth model. The height may then be further converted to height relative to the geoid, which is essentially mean sea level. These coordinates may be displayed, such as on a moving map display, or recorded or used by some other system, such as a vehicle guidance system.
User-satellite geometry
[edit]Although usually not formed explicitly in the receiver processing, the conceptual time differences of arrival (TDOAs) define the measurement geometry. Each TDOA corresponds to a hyperboloid of revolution (see Multilateration). The line connecting the two satellites involved (and its extensions) forms the axis of the hyperboloid. The receiver is located at the point where three hyperboloids intersect.[73][74]
It is sometimes incorrectly said that the user location is at the intersection of three spheres. While simpler to visualize, this is the case only if the receiver has a clock synchronized with the satellite clocks (i.e., the receiver measures true ranges to the satellites rather than range differences). There are marked performance benefits to the user carrying a clock synchronized with the satellites. Foremost is that only three satellites are needed to compute a position solution. If it were an essential part of the GPS concept that all users needed to carry a synchronized clock, a smaller number of satellites could be deployed, but the cost and complexity of the user equipment would increase.
Receiver in continuous operation
[edit]The description above is representative of a receiver start-up situation. Most receivers have a track algorithm, sometimes called a tracker, that combines sets of satellite measurements collected at different times—in effect, taking advantage of the fact that successive receiver positions are usually close to each other. After a set of measurements are processed, the tracker predicts the receiver location corresponding to the next set of satellite measurements. When the new measurements are collected, the receiver uses a weighting scheme to combine the new measurements with the tracker prediction. In general, a tracker can (a) improve receiver position and time accuracy, (b) reject bad measurements, and (c) estimate receiver speed and direction.
The disadvantage of a tracker is that changes in speed or direction can be computed only with a delay, and that derived direction becomes inaccurate when the distance traveled between two position measurements drops below or near the random error of position measurement. GPS units can use measurements of the Doppler shift of the signals received to compute velocity accurately.[75] More advanced navigation systems use additional sensors like a compass or an inertial navigation system to complement GPS.
Non-navigation applications
[edit]GPS requires four or more satellites to be visible for accurate navigation. The solution of the navigation equations gives the position of the receiver along with the difference between the time kept by the receiver's on-board clock and the true time-of-day, thereby eliminating the need for a more precise and possibly impractical receiver based clock. Applications for GPS such as time transfer, traffic signal timing, and synchronization of cell phone base stations, make use of this cheap and highly accurate timing. Some GPS applications use this time for display, or, other than for the basic position calculations, do not use it at all.
Although four satellites are required for normal operation, fewer apply in special cases. If one variable is already known, a receiver can determine its position using only three satellites. For example, a ship on the open ocean usually has a known elevation close to 0m, and the elevation of an aircraft may be known.[a] Some GPS receivers may use additional clues or assumptions such as reusing the last known altitude, dead reckoning, inertial navigation, or including information from the vehicle computer, to give a (possibly degraded) position when fewer than four satellites are visible.[76][77][78]
Structure
[edit]This section needs additional citations for verification. (March 2015) |
The current GPS consists of three major segments. These are the space segment, a control segment, and a user segment.[51] The U.S. Space Force develops, maintains, and operates the space and control segments. GPS satellites broadcast signals from space, and each GPS receiver uses these signals to calculate its three-dimensional location (latitude, longitude, and altitude) and the current time.[79]
Space segment
[edit]

The space segment (SS) is composed of 24 to 32 satellites, or Space Vehicles (SV), in medium Earth orbit, and also includes the payload adapters to the boosters required to launch them into orbit. The GPS design originally called for 24 SVs, eight each in three approximately circular orbits,[80] but this was modified to six orbital planes with four satellites each.[81] The six orbit planes have approximately 55° inclination (tilt relative to the Earth's equator) and are separated by 60° right ascension of the ascending node (angle along the equator from a reference point to the orbit's intersection).[82] The orbital period is one-half of a sidereal day, i.e., 11 hours and 58 minutes, so that the satellites pass over the same locations[83] or almost the same locations[84] every day. The orbits are arranged so that at least six satellites are always within line of sight from everywhere on the Earth's surface (see animation at right).[85] The result of this objective is that the four satellites are not evenly spaced (90°) apart within each orbit. In general terms, the angular difference between satellites in each orbit is 30°, 105°, 120°, and 105° apart, which sum to 360°.[86]
Orbiting at an altitude of approximately 20,200 km (12,600 mi); orbital radius of approximately 26,600 km (16,500 mi),[87] each SV makes two complete orbits each sidereal day, repeating the same ground track each day.[88] This was very helpful during development because even with only four satellites, correct alignment means all four are visible from one spot for a few hours each day. For military operations, the ground track repeat can be used to ensure good coverage in combat zones.
As of February 2019[update],[89] there are 31 satellites in the GPS constellation, 27 of which are in use at a given time with the rest allocated as stand-bys. A 32nd was launched in 2018, but as of July 2019 is still in evaluation. More decommissioned satellites are in orbit and available as spares. The additional satellites improve the precision of GPS receiver calculations by providing redundant measurements. With the increased number of satellites, the constellation was changed to a nonuniform arrangement. Such an arrangement was shown to improve accuracy but also improves reliability and availability of the system, relative to a uniform system, when multiple satellites fail.[90] With the expanded constellation, nine satellites are usually visible at any time from any point on the Earth with a clear horizon, ensuring considerable redundancy over the minimum four satellites needed for a position.
Control segment
[edit]
The control segment (CS) is composed of:
- a master control station (MCS),
- an alternative master control station,
- four dedicated ground antennas, and
- six dedicated monitor stations.
The MCS can also access Satellite Control Network (SCN) ground antennas (for additional command and control capability) and NGA (National Geospatial-Intelligence Agency) monitor stations. The flight paths of the satellites are tracked by dedicated U.S. Space Force monitoring stations in Hawaii, Kwajalein Atoll, Ascension Island, Diego Garcia, Colorado Springs, Colorado and Cape Canaveral, along with shared NGA monitor stations operated in England, Argentina, Ecuador, Bahrain, Australia and Washington DC.[91] The tracking information is sent to the MCS at Schriever Space Force Base 25 km (16 mi) ESE of Colorado Springs, which is operated by the 2nd Space Operations Squadron (2 SOPS) of the U.S. Space Force. Then 2 SOPS contacts each GPS satellite regularly with a navigational update using dedicated or shared (AFSCN) ground antennas (GPS dedicated ground antennas are located at Kwajalein, Ascension Island, Diego Garcia, and Cape Canaveral). These updates synchronize the atomic clocks on board the satellites to within a few nanoseconds of each other, and adjust the ephemeris of each satellite's internal orbital model. The updates are created by a Kalman filter that uses inputs from the ground monitoring stations, space weather information, and various other inputs.[92]
When a satellite's orbit is being adjusted, the satellite is marked unhealthy, so receivers do not use it. After the maneuver, engineers track the new orbit from the ground, upload the new ephemeris, and mark the satellite healthy again.
The operation control segment (OCS) currently serves as the control segment of record. It provides the operational capability that supports GPS users and keeps the GPS operational and performing within specification.
OCS successfully replaced the legacy 1970s-era mainframe computer at Schriever Air Force Base in September 2007. After installation, the system helped enable upgrades and provide a foundation for a new security architecture that supported U.S. armed forces.
OCS will continue to be the ground control system of record until the new segment, Next Generation GPS Operation Control System[8] (OCX), is fully developed and functional. The US Department of Defense has claimed that the new capabilities provided by OCX will be the cornerstone for revolutionizing GPS's mission capabilities, enabling U.S. Space Force to greatly enhance GPS operational services to U.S. combat forces, civil partners and myriad domestic and international users.[93][94] The GPS OCX program also will reduce cost, schedule and technical risk. It is designed to provide 50%[95] sustainment cost savings through efficient software architecture and Performance-Based Logistics. In addition, GPS OCX is expected to cost millions less than the cost to upgrade OCS while providing four times the capability.
The GPS OCX program represents a critical part of GPS modernization and provides significant information assurance improvements over the current GPS OCS program.
- OCX will have the ability to control and manage GPS legacy satellites as well as the next generation of GPS III satellites, while enabling the full array of military signals.
- Built on a flexible architecture that can rapidly adapt to the changing needs of today's and future GPS users allowing immediate access to GPS data and constellation status through secure, accurate and reliable information.
- Provides the warfighter with more secure, actionable and predictive information to enhance situational awareness.
- Enables new modernized signals (L1C, L2C, and L5) and has M-code capability, which the legacy system is unable to do.
- Provides significant information assurance improvements over the current program including detecting and preventing cyber attacks, while isolating, containing and operating during such attacks.
- Supports higher volume near real-time command and control capabilities and abilities.
On September 14, 2011,[96] the U.S. Air Force announced the completion of GPS OCX Preliminary Design Review and confirmed that the OCX program is ready for the next phase of development. The GPS OCX program missed major milestones and pushed its launch into 2021, 5 years past the original deadline. According to the Government Accounting Office in 2019, the 2021 deadline looked shaky.[97]
The project remained delayed in 2023, and was (as of June 2023) 73% over its original estimated budget.[98][99] In late 2023, Frank Calvelli, the assistant secretary of the Air Force for space acquisitions and integration, stated that the project was estimated to go live some time during the summer of 2024.[100]
User segment
[edit]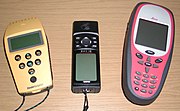
The user segment (US) is composed of hundreds of thousands of U.S. and allied military users of the secure GPS Precise Positioning Service, and tens of millions of civil, commercial and scientific users of the Standard Positioning Service. In general, GPS receivers are composed of an antenna, tuned to the frequencies transmitted by the satellites, receiver-processors, and a highly stable clock (often a crystal oscillator). They may also include a display for providing location and speed information to the user.
GPS receivers may include an input for differential corrections, using the RTCM SC-104 format. This is typically in the form of an RS-232 port at 4,800 bit/s speed. Data is actually sent at a much lower rate, which limits the accuracy of the signal sent using RTCM.[citation needed] Receivers with internal DGPS receivers can outperform those using external RTCM data.[citation needed] As of 2006[update], even low-cost units commonly include Wide Area Augmentation System (WAAS) receivers.

Many GPS receivers can relay position data to a PC or other device using the NMEA 0183 protocol. Although this protocol is officially defined by the National Marine Electronics Association (NMEA),[101] references to this protocol have been compiled from public records, allowing open source tools like gpsd to read the protocol without violating intellectual property laws.[clarification needed] Other proprietary protocols exist as well, such as the SiRF and MTK protocols. Receivers can interface with other devices using methods including a serial connection, USB, or Bluetooth.
Applications
[edit]This section needs additional citations for verification. (March 2015) |
While originally a military project, GPS is considered a dual-use technology, meaning it has significant civilian applications as well.
GPS has become a widely deployed and useful tool for commerce, scientific uses, tracking, and surveillance. GPS's accurate time facilitates everyday activities such as banking, mobile phone operations, and even the control of power grids by allowing well synchronized hand-off switching.[79]
Civilian
[edit]
Many civilian applications use one or more of GPS's three basic components: absolute location, relative movement, and time transfer.
- Amateur radio: clock synchronization required for several digital modes such as FT8, FT4 and JS8; also used with APRS for position reporting; is often critical during emergency and disaster communications support.
- Atmosphere: studying the troposphere delays (recovery of the water vapor content) and ionosphere delays (recovery of the number of free electrons).[102] Recovery of Earth surface displacements due to the atmospheric pressure loading.[103]
- Astronomy: both positional and clock synchronization data is used in astrometry and celestial mechanics and precise orbit determination.[104] GPS is also used in both amateur astronomy with small telescopes as well as by professional observatories for finding extrasolar planets.
- Automated vehicle: applying location and routes for cars and trucks to function without a human driver.
- Cartography: both civilian and military cartographers use GPS extensively.
- Cellular telephony: clock synchronization enables time transfer, which is critical for synchronizing its spreading codes with other base stations to facilitate inter-cell handoff and support hybrid GPS/cellular position detection for mobile emergency calls and other applications. The first handsets with integrated GPS launched in the late 1990s. The U.S. Federal Communications Commission (FCC) mandated the feature in either the handset or in the towers (for use in triangulation) in 2002 so emergency services could locate 911 callers. Third-party software developers later gained access to GPS APIs from Nextel upon launch, followed by Sprint in 2006, and Verizon soon thereafter.
- Clock synchronization: the accuracy of GPS time signals (±10 ns)[105] is second only to the atomic clocks they are based on, and is used in applications such as GPS disciplined oscillators.
- Disaster relief/emergency services: many emergency services depend upon GPS for location and timing capabilities.
- GPS-equipped radiosondes and dropsondes: measure and calculate the atmospheric pressure, wind speed and direction up to 27 km (89,000 ft) from the Earth's surface.
- Radio occultation for weather and atmospheric science applications.[106]
- Fleet tracking: used to identify, locate and maintain contact reports with one or more fleet vehicles in real-time.
- Geodesy: determination of Earth orientation parameters including the daily and sub-daily polar motion,[107] and length-of-day variabilities,[108] Earth's center-of-mass - geocenter motion,[109] and low-degree gravity field parameters.[110]
- Geofencing: vehicle tracking systems, person tracking systems, and pet tracking systems use GPS to locate devices that are attached to or carried by a person, vehicle, or pet. The application can provide continuous tracking and send notifications if the target leaves a designated (or "fenced-in") area.[111]
- Geotagging: applies location coordinates to digital objects such as photographs (in Exif data) and other documents for purposes such as creating map overlays with devices like Nikon GP-1
- GPS aircraft tracking
- GPS for mining: the use of RTK GPS has significantly improved several mining operations such as drilling, shoveling, vehicle tracking, and surveying. RTK GPS provides centimeter-level positioning accuracy.[112][113]
- GPS data mining: It is possible to aggregate GPS data from multiple users to understand movement patterns, common trajectories and interesting locations.[114] GPS data is today used in transportation and disaster engineering to forecast mobility in normal and evacuation situations (e.g., hurricanes, wildfires, earthquakes).[115][116][117][118]
- GPS tours: location determines what content to display; for instance, information about an approaching point of interest.
- Mental health: tracking mental health functioning and sociability.[119]
- Navigation: navigators value digitally precise velocity and orientation measurements, as well as precise positions in real-time with a support of orbit and clock corrections.[120]
- Orbit determination of low-orbiting satellites with GPS receiver installed on board, such as GOCE,[121] GRACE, Jason-1, Jason-2, TerraSAR-X, TanDEM-X, CHAMP, Sentinel-3,[122] and some cubesats, e.g., CubETH.
- Phasor measurements: GPS enables highly accurate timestamping of power system measurements, making it possible to compute phasors.
- Recreation: for example, Geocaching, Geodashing, GPS drawing, waymarking, and other kinds of location based mobile games such as Pokémon Go.
- Reference frames: realization and densification of the terrestrial reference frames[123] in the framework of Global Geodetic Observing System. Co-location in space between Satellite laser ranging[124] and microwave observations[125] for deriving global geodetic parameters.[126][127]
- Robotics: self-navigating, autonomous robots using GPS sensors,[128] which calculate latitude, longitude, time, speed, and heading.
- Sport: used in football and rugby for the control and analysis of the training load.[129]
- Surveying: surveyors use absolute locations to make maps and determine property boundaries.
- Tectonics: GPS enables direct fault motion measurement of earthquakes. Between earthquakes GPS can be used to measure crustal motion and deformation[130] to estimate seismic strain buildup for creating seismic hazard maps.
- Telematics: GPS technology integrated with computers and mobile communications technology in automotive navigation systems.
Restrictions on civilian use
[edit]The U.S. government controls the export of some civilian receivers. All GPS receivers capable of functioning above 60,000 ft (18 km) above sea level and 1,000 kn (500 m/s; 2,000 km/h; 1,000 mph), or designed or modified for use with unmanned missiles and aircraft, are classified as munitions (weapons)—which means they require State Department export licenses.[131] This rule applies even to otherwise purely civilian units that only receive the L1 frequency and the C/A (Coarse/Acquisition) code.
Disabling operation above these limits exempts the receiver from classification as a munition. Vendor interpretations differ. The rule refers to operation at both the target altitude and speed, but some receivers stop operating even when stationary. This has caused problems with some amateur radio balloon launches that regularly reach 30 km (100,000 feet).
These limits only apply to units or components exported from the United States. A growing trade in various components exists, including GPS units from other countries. These are expressly sold as ITAR-free.
Military
[edit]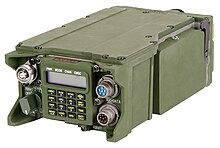


As of 2009, military GPS applications include:
- Navigation: Soldiers use GPS to find objectives, even in the dark or in unfamiliar territory, and to coordinate troop and supply movement. In the United States armed forces, commanders use the Commander's Digital Assistant and lower ranks use the Soldier Digital Assistant.[132]
- Frequency-Hopping Radio Clock Coordination: Military radio systems using frequency hopping modes, such as SINCGARS and HAVEQUICK, require all radios within a network to have the same time input to their internal clocks (+/-4 seconds in the case of SINCGARS) to be on the correct frequency at a given time. Military GPS receivers, such as the Precision Lightweight GPS Receiver (PLGR) and Defense Advanced GPS Receiver (DAGR), are used by radio operators within a radio network to properly input an accurate time to said radios internal clock. More modern military radios have internal GPS receivers that syncronize the internal clock automatically.
- Target tracking: Various military weapons systems use GPS to track potential ground and air targets before flagging them as hostile.[citation needed] These weapon systems pass target coordinates to precision-guided munitions to allow them to engage targets accurately. Military aircraft, particularly in air-to-ground roles, use GPS to find targets.
- Missile and projectile guidance: GPS allows accurate targeting of various military weapons including ICBMs, cruise missiles, precision-guided munitions and artillery shells. Embedded GPS receivers able to withstand accelerations of 12,000 g[133] or about 118 km/s2 (260,000 mph/s) have been developed for use in 155-millimeter (6.1 in) howitzer shells.[134]
- Search and rescue.
- Reconnaissance: Patrol movement can be managed more closely.
- GPS satellites carry a set of nuclear detonation detectors consisting of an optical sensor called a bhangmeter, an X-ray sensor, a dosimeter, and an electromagnetic pulse (EMP) sensor (W-sensor), that form a major portion of the United States Nuclear Detonation Detection System.[135][136] General William Shelton has stated that future satellites may drop this feature to save money.[137]
GPS type navigation was first used in war in the 1991 Persian Gulf War, before GPS was fully developed in 1995, to assist Coalition Forces to navigate and perform maneuvers in the war. The war also demonstrated the vulnerability of GPS to being jammed, when Iraqi forces installed jamming devices on likely targets that emitted radio noise, disrupting reception of the weak GPS signal.[138]
GPS's vulnerability to jamming is a threat that continues to grow as jamming equipment and experience grows.[139][140] GPS signals have been reported to have been jammed many times over the years for military purposes. Russia seems to have several objectives for this approach, such as intimidating neighbors while undermining confidence in their reliance on American systems, promoting their GLONASS alternative, disrupting Western military exercises, and protecting assets from drones.[141] China uses jamming to discourage US surveillance aircraft near the contested Spratly Islands.[142] North Korea has mounted several major jamming operations near its border with South Korea and offshore, disrupting flights, shipping and fishing operations.[143] Iranian Armed Forces disrupted the civilian airliner plane Flight PS752's GPS when it shot down the aircraft.[144][145]
In the Russo-Ukrainian War, GPS-guided munitions provided to Ukraine by NATO countries experienced significant failure rates as a result of Russian electronic warfare. Excalibur artillery shells efficiency rate hitting targets dropped from 70% to 6% as Russia adapted its electronic warfare activities.[146]
Timekeeping
[edit]Leap seconds
[edit]While most clocks derive their time from Coordinated Universal Time (UTC), the atomic clocks on the satellites are set to GPS time. The difference is that GPS time is not corrected to match the rotation of the Earth, so it does not contain new leap seconds or other corrections that are periodically added to UTC. GPS time was set to match UTC in 1980, but has since diverged. The lack of corrections means that GPS time remains at a constant offset with International Atomic Time (TAI) (TAI - GPS = 19 seconds). Periodic corrections are performed to the on-board clocks to keep them synchronized with ground clocks.[77]: Section 1.2.2
The GPS navigation message includes the difference between GPS time and UTC. As of January 2017,[update] GPS time is 18 seconds ahead of UTC because of the leap second added to UTC on December 31, 2016.[147] Receivers subtract this offset from GPS time to calculate UTC and specific time zone values. New GPS units may not show the correct UTC time until after receiving the UTC offset message. The GPS-UTC offset field can accommodate 255 leap seconds (eight bits).
Accuracy
[edit]GPS time is theoretically accurate to about 14 nanoseconds, due to the clock drift relative to International Atomic Time that the atomic clocks in GPS transmitters experience.[148] Most receivers lose some accuracy in their interpretation of the signals and are only accurate to about 100 nanoseconds.[149][150]
Relativistic corrections
[edit]The GPS implements two major corrections to its time signals for relativistic effects: one for relative velocity of satellite and receiver, using the special theory of relativity, and one for the difference in gravitational potential between satellite and receiver, using general relativity. The acceleration of the satellite could also be computed independently as a correction, depending on purpose, but normally the effect is already dealt with in the first two corrections.[151][152]
Format
[edit]As opposed to the year, month, and day format of the Gregorian calendar, the GPS date is expressed as a week number and a seconds-into-week number. The week number is transmitted as a ten-bit field in the C/A and P(Y) navigation messages, and so it becomes zero again every 1,024 weeks (19.6 years). GPS week zero started at 00:00:00 UTC (00:00:19 TAI) on January 6, 1980, and the week number became zero again for the first time at 23:59:47 UTC on August 21, 1999 (00:00:19 TAI on August 22, 1999). It happened the second time at 23:59:42 UTC on April 6, 2019. To determine the current Gregorian date, a GPS receiver must be provided with the approximate date (to within 3,584 days) to correctly translate the GPS date signal. To address this concern in the future the modernized GPS civil navigation (CNAV) message will use a 13-bit field that only repeats every 8,192 weeks (157 years), thus lasting until 2137 (157 years after GPS week zero).
Communication
[edit]The navigational signals transmitted by GPS satellites encode a variety of information including satellite positions, the state of the internal clocks, and the health of the network. These signals are transmitted on two separate carrier frequencies that are common to all satellites in the network. Two different encodings are used: a public encoding that enables lower resolution navigation, and an encrypted encoding used by the U.S. military.[153]
Message format
[edit]GPS message format Subframes Description 1 Satellite clock,
GPS time relationship2–3 Ephemeris
(precise satellite orbit)4–5 Almanac component
(satellite network synopsis,
error correction)
Each GPS satellite continuously broadcasts a navigation message on L1 (C/A and P/Y) and L2 (P/Y) frequencies at a rate of 50 bits per second (see bitrate). Each complete message takes 750 seconds (12+1⁄2 minutes) to complete. The message structure has a basic format of a 1500-bit-long frame made up of five subframes, each subframe being 300 bits (6 seconds) long. Subframes 4 and 5 are subcommutated 25 times each, so that a complete data message requires the transmission of 25 full frames. Each subframe consists of ten words, each 30 bits long. Thus, with 300 bits in a subframe times 5 subframes in a frame times 25 frames in a message, each message is 37,500 bits long. At a transmission rate of 50-bit/s, this gives 750 seconds to transmit an entire almanac message (GPS). Each 30-second frame begins precisely on the minute or half-minute as indicated by the atomic clock on each satellite.[154]
The first subframe of each frame encodes the week number and the time within the week,[155] as well as the data about the health of the satellite. The second and the third subframes contain the ephemeris – the precise orbit for the satellite. The fourth and fifth subframes contain the almanac, which contains coarse orbit and status information for up to 32 satellites in the constellation as well as data related to error correction. Thus, to obtain an accurate satellite location from this transmitted message, the receiver must demodulate the message from each satellite it includes in its solution for 18 to 30 seconds. To collect all transmitted almanacs, the receiver must demodulate the message for 732 to 750 seconds or 12+1⁄2 minutes.[156]
All satellites broadcast at the same frequencies, encoding signals using unique code-division multiple access (CDMA) so receivers can distinguish individual satellites from each other. The system uses two distinct CDMA encoding types: the coarse/acquisition (C/A) code, which is accessible by the general public, and the precise (P(Y)) code, which is encrypted so that only the U.S. military and other NATO nations who have been given access to the encryption code can access it.[157]
The ephemeris is updated every 2 hours and is sufficiently stable for 4 hours, with provisions for updates every 6 hours or longer in non-nominal conditions. The almanac is updated typically every 24 hours. Additionally, data for a few weeks following is uploaded in case of transmission updates that delay data upload.[citation needed]
Satellite frequencies
[edit]GPS frequency overview[158]: 607 Band Frequency Description L1 1575.42 MHz Coarse-acquisition (C/A) and encrypted precision (P(Y)) codes, plus the L1 civilian (L1C) and military (M) codes on Block III and newer satellites. L2 1227.60 MHz P(Y) code, plus the L2C and military codes on the Block IIR-M and newer satellites. L3 1381.05 MHz Used for nuclear detonation (NUDET) detection. L4 1379.913 MHz Being studied for additional ionospheric correction. L5 1176.45 MHz Used as a civilian safety-of-life (SoL) signal on Block IIF and newer satellites.
All satellites broadcast at the same two frequencies, 1.57542 GHz (L1 signal) and 1.2276 GHz (L2 signal). The satellite network uses a CDMA spread-spectrum technique[158]: 607 where the low-bitrate message data is encoded with a high-rate pseudo-random (PRN) sequence that is different for each satellite. The receiver must be aware of the PRN codes for each satellite to reconstruct the actual message data. The C/A code, for civilian use, transmits data at 1.023 million chips per second, whereas the P code, for U.S. military use, transmits at 10.23 million chips per second. The actual internal reference of the satellites is 10.22999999543 MHz to compensate for relativistic effects[159][160] that make observers on the Earth perceive a different time reference with respect to the transmitters in orbit. The L1 carrier is modulated by both the C/A and P codes, while the L2 carrier is only modulated by the P code.[86] The P code can be encrypted as a so-called P(Y) code that is only available to military equipment with a proper decryption key. Both the C/A and P(Y) codes impart the precise time-of-day to the user.
The L3 signal at a frequency of 1.38105 GHz is used to transmit data from the satellites to ground stations. This data is used by the United States Nuclear Detonation (NUDET) Detection System (USNDS) to detect, locate, and report nuclear detonations (NUDETs) in the Earth's atmosphere and near space.[161] One usage is the enforcement of nuclear test ban treaties.
The L4 band at 1.379913 GHz is being studied for additional ionospheric correction.[158]: 607
The L5 frequency band at 1.17645 GHz was added in the process of GPS modernization. This frequency falls into an internationally protected range for aeronautical navigation, promising little or no interference under all circumstances. The first Block IIF satellite that provides this signal was launched in May 2010.[162] On February 5, 2016, the 12th and final Block IIF satellite was launched.[163] The L5 consists of two carrier components that are in phase quadrature with each other. Each carrier component is bi-phase shift key (BPSK) modulated by a separate bit train. "L5, the third civil GPS signal, will eventually support safety-of-life applications for aviation and provide improved availability and accuracy."[164]
![]() | This section needs to be updated.(May 2021) |
In 2011, a conditional waiver was granted to LightSquared to operate a terrestrial broadband service near the L1 band. Although LightSquared had applied for a license to operate in the 1525 to 1559 band as early as 2003 and it was put out for public comment, the FCC asked LightSquared to form a study group with the GPS community to test GPS receivers and identify issues that might arise due to the larger signal power from the LightSquared terrestrial network. The GPS community had not objected to the LightSquared (formerly MSV and SkyTerra) applications until November 2010, when LightSquared applied for a modification to its Ancillary Terrestrial Component (ATC) authorization. This filing (SAT-MOD-20101118-00239) amounted to a request to run several orders of magnitude more power in the same frequency band for terrestrial base stations, essentially repurposing what was supposed to be a "quiet neighborhood" for signals from space as the equivalent of a cellular network. Testing in the first half of 2011 has demonstrated that the impact of the lower 10 MHz of spectrum is minimal to GPS devices (less than 1% of the total GPS devices are affected). The upper 10 MHz intended for use by LightSquared may have some impact on GPS devices. There is some concern that this may seriously degrade the GPS signal for many consumer uses.[165][166] Aviation Week magazine reports that the latest testing (June 2011) confirms "significant jamming" of GPS by LightSquared's system.[167]
Demodulation and decoding
[edit]
Because all of the satellite signals are modulated onto the same L1 carrier frequency, the signals must be separated after demodulation. This is done by assigning each satellite a unique binary sequence known as a Gold code. The signals are decoded after demodulation using addition of the Gold codes corresponding to the satellites monitored by the receiver.[168][169]
If the almanac information has previously been acquired, the receiver picks the satellites to listen for by their PRNs, unique numbers in the range 1 through 32. If the almanac information is not in memory, the receiver enters a search mode until a lock is obtained on one of the satellites. To obtain a lock, it is necessary that there be an unobstructed line of sight from the receiver to the satellite. The receiver can then acquire the almanac and determine the satellites it should listen for. As it detects each satellite's signal, it identifies it by its distinct C/A code pattern. There can be a delay of up to 30 seconds before the first estimate of position because of the need to read the ephemeris data.
Processing of the navigation message enables the determination of the time of transmission and the satellite position at this time. For more information see Demodulation and Decoding, Advanced.
Navigation equations
[edit]Problem statement
[edit]The receiver uses messages received from satellites to determine the satellite positions and time sent. The x, y, and z components of satellite position and the time sent (s) are designated as [xi, yi, zi, si] where the subscript i denotes the satellite and has the value 1, 2, ..., n, where n ≥ 4. When the time of message reception indicated by the on-board receiver clock is , the true reception time is , where b is the receiver's clock bias from the much more accurate GPS clocks employed by the satellites. The receiver clock bias is the same for all received satellite signals (assuming the satellite clocks are all perfectly synchronized). The message's transit time is , where si is the satellite time. Assuming the message traveled at the speed of light, c, the distance traveled is .
For n satellites, the equations to satisfy are:
where di is the geometric distance or range between receiver and satellite i (the values without subscripts are the x, y, and z components of receiver position):
Defining pseudoranges as , we see they are biased versions of the true range:
Since the equations have four unknowns [x, y, z, b]—the three components of GPS receiver position and the clock bias—signals from at least four satellites are necessary to attempt solving these equations. They can be solved by algebraic or numerical methods. Existence and uniqueness of GPS solutions are discussed by Abell and Chaffee.[73] When n is greater than four, this system is overdetermined and a fitting method must be used.
The amount of error in the results varies with the received satellites' locations in the sky, since certain configurations (when the received satellites are close together in the sky) cause larger errors. Receivers usually calculate a running estimate of the error in the calculated position. This is done by multiplying the basic resolution of the receiver by quantities called the geometric dilution of position (GDOP) factors, calculated from the relative sky directions of the satellites used.[172] The receiver location is expressed in a specific coordinate system, such as latitude and longitude using the WGS 84 geodetic datum or a country-specific system.[173]
Geometric interpretation
[edit]The GPS equations can be solved by numerical and analytical methods. Geometrical interpretations can enhance the understanding of these solution methods.
Spheres
[edit]
The measured ranges, called pseudoranges, contain clock errors. In a simplified idealization in which the ranges are synchronized, these true ranges represent the radii of spheres, each centered on one of the transmitting satellites. The solution for the position of the receiver is then at the intersection of the surfaces of these spheres; see trilateration (more generally, true-range multilateration). Signals from at minimum three satellites are required, and their three spheres would typically intersect at two points.[174] One of the points is the location of the receiver, and the other moves rapidly in successive measurements and would not usually be on Earth's surface.
In practice, there are many sources of inaccuracy besides clock bias, including random errors as well as the potential for precision loss from subtracting numbers close to each other if the centers of the spheres are relatively close together. This means that the position calculated from three satellites alone is unlikely to be accurate enough. Data from more satellites can help because of the tendency for random errors to cancel out and also by giving a larger spread between the sphere centers. But at the same time, more spheres will not generally intersect at one point. Therefore, a near intersection gets computed, typically via least squares. The more signals available, the better the approximation is likely to be.
Hyperboloids
[edit]
If the pseudorange between the receiver and satellite i and the pseudorange between the receiver and satellite j are subtracted, pi − pj, the common receiver clock bias (b) cancels out, resulting in a difference of distances di − dj. The locus of points having a constant difference in distance to two points (here, two satellites) is a hyperbola on a plane and a hyperboloid of revolution (more specifically, a two-sheeted hyperboloid) in 3D space (see Multilateration). Thus, from four pseudorange measurements, the receiver can be placed at the intersection of the surfaces of three hyperboloids each with foci at a pair of satellites. With additional satellites, the multiple intersections are not necessarily unique, and a best-fitting solution is sought instead.[73][74][175][176][177][178]
Inscribed sphere
[edit]
The receiver position can be interpreted as the center of an inscribed sphere (insphere) of radius bc, given by the receiver clock bias b (scaled by the speed of light c). The insphere location is such that it touches other spheres. The circumscribing spheres are centered at the GPS satellites, whose radii equal the measured pseudoranges pi. This configuration is distinct from the one described above, in which the spheres' radii were the unbiased or geometric ranges di.[177]: 36–37 [179]
Hypercones
[edit]The clock in the receiver is usually not of the same quality as the ones in the satellites and will not be accurately synchronized to them. This produces pseudoranges with large differences compared to the true distances to the satellites. Therefore, in practice, the time difference between the receiver clock and the satellite time is defined as an unknown clock bias b. The equations are then solved simultaneously for the receiver position and the clock bias. The solution space [x, y, z, b] can be seen as a four-dimensional spacetime, and signals from at minimum four satellites are needed. In that case each of the equations describes a hypercone (or spherical cone),[180] with the cusp located at the satellite, and the base a sphere around the satellite. The receiver is at the intersection of four or more of such hypercones.
Solution methods
[edit]Least squares
[edit]When more than four satellites are available, the calculation can use the four best, or more than four simultaneously (up to all visible satellites), depending on the number of receiver channels, processing capability, and geometric dilution of precision (GDOP).
Using more than four involves an over-determined system of equations with no unique solution; such a system can be solved by a least-squares or weighted least squares method.[170]
Iterative
[edit]Both the equations for four satellites, or the least squares equations for more than four, are non-linear and need special solution methods. A common approach is by iteration on a linearized form of the equations, such as the Gauss–Newton algorithm.
The GPS was initially developed assuming use of a numerical least-squares solution method—i.e., before closed-form solutions were found.
Closed-form
[edit]One closed-form solution to the above set of equations was developed by S. Bancroft.[171][181] Its properties are well known;[73][74][182] in particular, proponents claim it is superior in low-GDOP situations, compared to iterative least squares methods.[181]
Метод Бэнкрофта является алгебраическим, а не численным, и может использоваться для четырех и более спутников. При использовании четырех спутников ключевыми этапами являются обращение матрицы 4х4 и решение квадратного уравнения с одной переменной. Метод Бэнкрофта дает одно или два решения для неизвестных величин. Когда их два (обычно так), только один является разумным решением для околоземного пространства. [171]
Когда приемник использует для решения более четырех спутников, Бэнкрофт использует обобщенное обратное (т. е. псевдообратное) для поиска решения. Было доказано, что итерационные методы, такие как подход алгоритма Гаусса – Ньютона для решения переопределенных нелинейных задач наименьших квадратов, обычно обеспечивают более точные решения. [183]
Лейк и др. (2015) утверждает, что «решение Бэнкрофта (1985) является очень ранним, если не первым, решением в закрытой форме». [184] Позже были опубликованы и другие решения в закрытой форме: [185] [186] хотя их принятие на практике неясно.
Источники ошибок и анализ
[ редактировать ]Анализ ошибок GPS исследует источники ошибок в результатах GPS и ожидаемый размер этих ошибок. GPS корректирует ошибки часов приемника и другие эффекты, но некоторые остаточные ошибки остаются неисправленными. Источники ошибок включают измерения времени прибытия сигнала, численные расчеты, атмосферные эффекты (ионосферные/тропосферные задержки), данные эфемерид и часов, многолучевые сигналы, а также естественные и искусственные помехи. Величина остаточных ошибок от этих источников зависит от геометрического снижения точности. Искусственные ошибки могут возникнуть в результате постановки помех устройствам и представлять угрозу для кораблей и самолетов. [187] или из-за преднамеренного ухудшения сигнала из-за избирательной доступности, которая ограничивала точность до ≈ 6–12 м (20–40 футов), но была отключена с 1 мая 2000 года. [188] [189]
Повышение точности и съемка
[ редактировать ]![]() | Эта статья требует внимания специалиста по географии . Конкретная проблема заключается в следующем: возможно дублирование ссылок на один и тот же фазовый материал, особенно. между RTK и отслеживанием фазы несущей. ( март 2020 г. ) |
Улучшение GNSS относится к методам, используемым для повышения точности информации о местоположении, предоставляемой глобальной системой позиционирования или другими глобальными навигационными спутниковыми системами в целом, сетью спутников, используемых для навигации.
Методы повышения точности основаны на интеграции внешней информации в процесс расчета. Существует множество таких систем, и они обычно называются или описываются в зависимости от того, как датчик GPS получает информацию. Некоторые системы передают дополнительную информацию об источниках ошибок (например, дрейф часов, эфемериды или ионосферная задержка ), другие обеспечивают прямые измерения того, насколько сигнал был отключен в прошлом, а третья группа предоставляет дополнительную навигационную информацию или информацию о транспортном средстве, которую необходимо интегрировать. в процесс расчета.Нормативные вопросы спектра, касающиеся GPS-приемников
[ редактировать ]В Соединенных Штатах приемники GPS регулируются правилами Федеральной комиссии по связи (FCC), часть 15 . Как указано в руководствах к устройствам с поддержкой GPS, продаваемым в США, как устройство, соответствующее Части 15, оно «должно принимать любые получаемые помехи, включая помехи, которые могут вызвать нежелательную работу». [190] Что касается, в частности, устройств GPS, FCC заявляет, что производители приемников GPS «должны использовать приемники, которые разумно дискриминируют прием сигналов за пределами выделенного им спектра». [191] В течение последних 30 лет приемники GPS работали в диапазоне мобильной спутниковой службы и без каких-либо проблем дискриминировали прием мобильных спутниковых служб, таких как Inmarsat.
Спектр, выделенный для использования GPS L1 FCC, составляет от 1559 до 1610 МГц, в то время как спектр, выделенный для использования в режиме «спутник-земля», принадлежащий Lightsquared, является диапазоном мобильной спутниковой службы. [192] С 1996 года FCC разрешила лицензировать использование спектра, соседнего с диапазоном GPS от 1525 до 1559 МГц, компании Вирджинии LightSquared из . 1 марта 2001 года FCC получила заявку от предшественника LightSquared, Motient Services, на использование выделенных им частот для интегрированной спутниково-наземной службы. [193] В 2002 году Совет отрасли GPS США заключил соглашение о внеполосных излучениях (OOBE) с LightSquared, чтобы предотвратить передачу сигналов наземных станций LightSquared в соседний диапазон GPS от 1559 до 1610 МГц. [194] В 2004 году FCC приняла соглашение OOBE, разрешив LightSquared развернуть вспомогательную наземную сеть для своей спутниковой системы, известную как вспомогательные компоненты башни (ATC): «Мы разрешим MSS ATC при условии, что добавленный наземный компонент остается вспомогательным к основному предложению MSS. Мы не намерены и не позволим, чтобы наземный компонент стал отдельной услугой». [195] Это разрешение было рассмотрено и одобрено Межведомственным консультативным комитетом по радио США, в который входят Министерство сельского хозяйства США , Космические силы США, Армия США, Береговая охрана США , Федеральное управление гражданской авиации , Национальное управление по аэронавтике и исследованию космического пространства аэронавтике и исследованию космического пространства (НАСА). (НАСА), Департамент США по внутренних дел и Министерство транспорта США . [196]
В январе 2011 года FCC условно разрешила оптовым клиентам LightSquared, таким как Best Buy , Sharp и C Spire , приобретать у LightSquared только интегрированные наземные спутниковые услуги и перепродавать эту интегрированную услугу на устройствах, оборудованных только для используйте наземный сигнал, используя выделенные LightSquared частоты от 1525 до 1559 МГц. [197] В декабре 2010 года производители GPS-приемников выразили Федеральной комиссии по связи обеспокоенность тем, что сигнал LightSquared будет мешать работе GPS-приемников. [165] хотя политические соображения FCC, приведшие к принятию приказа от января 2011 года, не касались каких-либо предлагаемых изменений максимального количества наземных станций LightSquared или максимальной мощности, на которой эти станции могли работать. Приказ от января 2011 года ставит окончательное разрешение в зависимость от исследований проблем помех GPS, проводимых рабочей группой под руководством LightSquared при участии отрасли GPS и федерального агентства. 14 февраля 2012 года FCC инициировала процедуру отмены постановления LightSquared об условном отказе на основании заключения NTIA о том, что в настоящее время не существует практического способа уменьшить потенциальные помехи GPS.
Производители GPS-приемников разрабатывают GPS-приемники для использования спектра за пределами выделенного GPS диапазона. В некоторых случаях приемники GPS рассчитаны на использование спектра до 400 МГц в любом направлении от частоты L1 1575,42 МГц, поскольку мобильные спутниковые службы в этих регионах ведут вещание из космоса на землю и на уровнях мощности, соизмеримых с мобильными спутниковыми службами. . [198] В соответствии с правилами Части 15 Федеральной комиссии по связи (FCC), на GPS-приемники не распространяется гарантия защиты от сигналов за пределами выделенного GPS спектра. [191] Вот почему GPS работает рядом с диапазоном мобильной спутниковой службы, а также почему диапазон мобильной спутниковой службы работает рядом с GPS. Симбиотическая взаимосвязь распределения спектра гарантирует, что пользователи обоих диапазонов могут работать совместно и свободно.
В феврале 2003 года FCC приняла правила, которые позволили лицензиатам мобильной спутниковой службы (MSS), таким как LightSquared, построить небольшое количество вспомогательных наземных вышек в своем лицензированном спектре, чтобы «способствовать более эффективному использованию спектра наземной беспроводной связи». [199] В этих правилах 2003 года FCC заявила: «Предварительно ожидается, что наземная [коммерческая служба мобильной радиосвязи ('CMRS')] и MSS ATC будут иметь разные цены, покрытие, прием и распространение продуктов; поэтому эти две услуги выглядят в лучшем случае являться несовершенными заменителями друг друга, работающими преимущественно в разных сегментах рынка... МСС УВД вряд ли будет напрямую конкурировать с наземными CMRS за одну и ту же клиентскую базу...". В 2004 году FCC уточнила, что наземные вышки будут вспомогательными, отметив: «Мы разрешим MSS ATC при условии, что добавленный наземный компонент останется вспомогательным по отношению к основному предложению MSS. Мы не намерены и не будем позвольте наземному компоненту стать отдельной услугой». [195] В июле 2010 года FCC заявила, что ожидает, что LightSquared будет использовать свои полномочия для предложения интегрированной спутниково-наземной услуги для «предоставления услуг мобильной широкополосной связи, аналогичных тем, которые предоставляются наземными провайдерами мобильной связи, и усиления конкуренции в секторе мобильной широкополосной связи». [200] Производители GPS-приемников утверждают, что лицензированный спектр LightSquared от 1525 до 1559 МГц никогда не предполагался для использования для высокоскоростного беспроводного широкополосного доступа на основании постановлений FCC ATC 2003 и 2004 годов, в которых ясно указано, что вспомогательный компонент башни (ATC) фактически будет , вспомогательный по отношению к основному спутниковому компоненту. [201] Чтобы заручиться общественной поддержкой усилий по продолжению разрешения FCC 2004 года на вспомогательный наземный компонент LightSquared вместо простой наземной службы LTE в диапазоне мобильной спутниковой службы, производитель GPS-приемников Trimble Navigation Ltd. сформировал «Коалицию за спасение нашего GPS». [202]
FCC и LightSquared публично обязались решить проблему помех GPS, прежде чем сети будет разрешено работать. [203] [204] По словам Криса Дэнси из Ассоциации владельцев самолетов и пилотов , пилоты авиакомпаний, системы которых будут затронуты, «могут сбиться с курса и даже не осознавать этого». [205] Проблемы могут также повлиять на модернизацию Федеральным управлением гражданской авиации системы управления воздушным движением , руководство Министерства обороны США и местные службы экстренной помощи , включая службу 911 . [205]
14 февраля 2012 года Федеральная комиссия связи приняла решение запретить создание запланированной национальной широкополосной сети LightSquared после того, как Национальное управление по телекоммуникациям и информации (NTIA), федеральное агентство, которое координирует использование спектра военными и другими федеральными правительственными организациями, проинформировало ее о том, что «существует в настоящее время нет практического способа смягчить потенциальное вмешательство». [206] [207] LightSquared оспаривает действия FCC. [ нужно обновить ]
Подобные системы
[ редактировать ]
После внедрения GPS в США другие страны также разработали свои собственные системы спутниковой навигации. Эти системы включают в себя:
- Российская глобальная навигационная спутниковая система ( ГЛОНАСС ) была разработана одновременно с GPS, но до середины 2000-х годов страдала от неполного покрытия земного шара. [208] Прием ГЛОНАСС в дополнение к GPS может быть объединен в приемнике, что позволяет использовать дополнительные спутники для более быстрого определения местоположения и повышения точности с точностью до двух метров (6,6 футов). [209] [210]
- Китайская навигационная спутниковая система BeiDou начала глобальное обслуживание в 2018 году и завершила свое полное развертывание в 2020 году. [211]
- — Навигационная спутниковая система Galileo глобальная система, разрабатываемая Европейским Союзом и другими странами-партнерами, начала работу в 2016 году. [212] и, как ожидается, будет полностью развернут к 2020 году. [ нужно обновить ]
- Японская спутниковая система «Квази-Зенит» (QZSS) представляет собой спутниковую дополняющую систему GPS для повышения точности GPS в Азии и Океании, а запуск спутниковой навигации, независимой от GPS, запланирован на 2023 год. [213]
- Индийская региональная навигационная спутниковая система , развернутая Индией.
См. также
[ редактировать ]- Список спутников GPS
- спутниковые блоки GPS
- GPS-сигналы
- Программное обеспечение спутниковой навигации
- GPS/ИНС
- GPS-спуфинг
- Система внутреннего позиционирования
- Локальная система расширения
- Локальная система позиционирования
- Военное изобретение
- Отслеживание мобильного телефона
- Навигационный парадокс
- Уведомление для пользователей Navstar
- S-GPS
- Геостационарный спутник-зонд
Примечания
[ редактировать ]- ^ На самом деле, маловероятно, что корабль окажется точно на высоте 0 м из-за приливов и других факторов, которые создают несоответствие между средним уровнем моря и фактическим уровнем моря. В открытом океане приливы и отливы обычно различаются примерно на 0,6 м, но есть места ближе к суше, где они могут отличаться более чем на 15 м. см. в разделе «Диапазон приливов» . Более подробную информацию и ссылки
- ^ Орбитальные периоды и скорости рассчитываются по соотношениям 4π 2 Р 3 = Т 2 ГМ и Ви 2 R = GM , где R — радиус орбиты в метрах; Т — орбитальный период в секундах; V – орбитальная скорость, м/с; G — гравитационная постоянная, примерно 6,673 × 10. −11 Нм 2 /кг 2 ; M — масса Земли, примерно 5,98 × 10. 24 кг (1,318 × 10 25 фунт).
- ^ Примерно в 8,6 раз, когда Луна находится ближе всего (т.е. 363 104 км / 42 164 км ) , в 9,6 раза, когда Луна находится дальше всего (т. е. 405 696 км / 42 164 км ) .
Ссылки
[ редактировать ]- ^ Перейти обратно: а б «Финансирование программы на 2023 финансовый год» . 27 апреля 2022 г. . Проверено 24 сентября 2023 г.
- ^ Министерство транспорта США ; Федеральное управление гражданской авиации (31 октября 2008 г.). «Стандарт производительности системы глобального позиционирования (WAAS)» (PDF) . п. Б-3. Архивировано (PDF) из оригинала 27 апреля 2017 г. Проверено 3 января 2012 г.
- ^ Министерство обороны США (сентябрь 2008 г.). «Стандарт качества услуг позиционирования глобальной системы позиционирования – 4-е издание» (PDF) . Архивировано (PDF) из оригинала 27 апреля 2017 г. Проверено 21 апреля 2017 г.
- ^ Справочно-научный раздел (19 ноября 2019 г.). «Что такое GPS? Как он работает?» . Повседневные тайны . Библиотека Конгресса . Архивировано из оригинала 12 апреля 2022 года . Проверено 12 апреля 2022 г.
- ^ Национальное координационное бюро по космическому позиционированию, навигации и времени (22 февраля 2021 г.). «Что такое GPS?» . Архивировано из оригинала 6 мая 2021 года . Проверено 5 мая 2021 г.
- ^ Макдаффи, Жукуай (19 июня 2017 г.). «Почему военные предоставили общественности GPS» . Популярная механика . Архивировано из оригинала 28 января 2020 года . Проверено 1 февраля 2020 г.
- ^ Перейти обратно: а б с Национальное координационное бюро по космическому позиционированию, навигации и времени (3 марта 2022 г.). «Точность GPS» . GPS.gov . Архивировано из оригинала 12 апреля 2022 года . Проверено 12 апреля 2022 г.
- ^ Перейти обратно: а б «Информационные бюллетени: Сегмент расширенного управления GPS (OCX)» . Лос-Анджелес.af.mil. 25 октября 2011. Архивировано из оригинала 3 мая 2012 года . Проверено 6 ноября 2011 г.
- ^ Кастренакес, Якоб (25 сентября 2017 г.). «В следующем году в некоторых телефонах GPS будет иметь точность в пределах одного фута» . Грань . Архивировано из оригинала 18 января 2018 года . Проверено 17 января 2018 г.
- ^ Мур, Сэмюэл К. (21 сентября 2017 г.). «Сверхточные GPS-чипы появятся в смартфонах в 2018 году» . IEEE-спектр . Архивировано из оригинала 18 января 2018 года . Проверено 17 января 2018 г.
- ^ «Как определить свое местоположение с помощью GPS?» . НИСТ . Национальный институт стандартов и технологий. 17 марта 2021 г. Проверено 7 марта 2022 г.
- ^ «Новые гражданские сигналы» . GPS.gov . Проверено 22 ноября 2023 г. .
- ^ Национальный исследовательский совет (США). Комитет по будущему системы глобального позиционирования; Национальная академия государственного управления (1995 год). Система глобального позиционирования: общее национальное достояние: рекомендации по техническим усовершенствованиям и усовершенствованиям . Пресса национальных академий. п. 16. ISBN 978-0-309-05283-2 . Проверено 16 августа 2013 г.
- ^ Энн Даррин; Бет Л. О'Лири (26 июня 2009 г.). Справочник по космической инженерии, археологии и наследию . ЦРК Пресс. стр. 239–240. ISBN 978-1-4200-8432-0 . Архивировано из оригинала 14 августа 2021 года . Проверено 28 июля 2021 г.
- ^ Баттерли, Амелия (20 мая 2018 г.). «100 женщин: Глэдис Уэст — «скрытая фигура» GPS» . Новости Би-би-си . Архивировано из оригинала 13 февраля 2019 года . Проверено 17 января 2019 г.
- ^ Мохдин, Аамна (19 ноября 2020 г.). «Глэдис Уэст: скрытая фигура, которая помогла изобрести GPS» . Хранитель . ISSN 0261-3077 . Проверено 29 ноября 2023 г.
- ^ Relativistische Zeitdilatation eines künstlichen Satelliten (Релятивистское замедление времени искусственного спутника . Astronautica Acta II (на немецком языке) (25). Проверено 19 октября 2014 года. Архивировано из оригинала 3 июля 2014 года . Проверено 20 октября 2014 года .
- ^ Гайер, Уильям Х.; Вайфенбах, Джордж К. (1997). «Генезис спутниковой навигации» (PDF) . Технический дайджест Johns Hopkins APL . 19 (1): 178–181. Архивировано из оригинала (PDF) 12 мая 2012 года . Проверено 9 апреля 2012 г.
- ^ Стивен Джонсон (2010), Откуда берутся хорошие идеи, естественная история инноваций , Нью-Йорк: Riverhead Books.
- ^ Хелен Э. Уорт; Мэйм Уоррен (2009). Транзит в Завтра. Пятьдесят лет космических исследований в Лаборатории прикладной физики Университета Джонса Хопкинса (PDF) . Архивировано (PDF) из оригинала 26 декабря 2020 г. Проверено 3 марта 2013 г.
- ^ Перейти обратно: а б Екатерина Александров (апрель 2008 г.). «История GPS» . Архивировано из оригинала 24 февраля 2013 года.
- ^ DARPA: 50 лет преодоления разрыва . Апрель 2008 г. Архивировано из оригинала 6 мая 2011 г.
- ^ Хауэлл, Элизабет. «Навстар: Спутниковая сеть GPS» . SPACE.com. Архивировано из оригинала 17 февраля 2013 года . Проверено 14 февраля 2013 г.
- ^ Джерри Прок. «Омега» . Jproc.ca. Архивировано из оригинала 5 января 2010 года . Проверено 8 декабря 2009 г.
- ^ «Почему Министерство обороны разработало GPS?» . Trimble Navigation Ltd. Архивировано из оригинала 18 октября 2007 года . Проверено 13 января 2010 г.
- ^ «Прокладывая курс на глобальную навигацию» . Аэрокосмическая корпорация. Архивировано из оригинала 1 ноября 2002 года . Проверено 14 октября 2013 г.
- ^ «Руководство по системе глобального позиционирования (GPS) – временная шкала GPS» . РадиоШак. Архивировано из оригинала 13 февраля 2010 года . Проверено 14 января 2010 г.
- ^ «Geodetic Explorer – Пресс-кит» (PDF) . НАСА. 29 октября 1965 года. Архивировано (PDF) из оригинала 11 февраля 2014 года . Проверено 20 октября 2015 г.
- ^ «СЕКОР Хронология» . Энциклопедия астронавтики Марка Уэйда . Архивировано из оригинала 16 января 2010 года . Проверено 19 января 2010 г.
- ^ Жюри, HL, 1973, Применение фильтра Калмана для навигации в реальном времени с использованием синхронных спутников, Труды 10-го Международного симпозиума по космическим технологиям и науке, Токио, 945–952.
- ^ «Пересмотр развертывания MX» . au.af.mil . Архивировано из оригинала 25 июня 2017 года . Проверено 7 июня 2013 г.
- ^ Дик, Стивен; Лауниус, Роджер (2007). Социальное влияние космических полетов (PDF) . Вашингтон, округ Колумбия: Типография правительства США. п. 331. ИСБН 978-0-16-080190-7 . Архивировано (PDF) из оригинала 3 марта 2013 г. Проверено 20 июля 2019 г.
- ^ Майкл Рассел Рип; Джеймс М. Хасик (2002). Революция точности: GPS и будущее воздушной войны . Издательство Военно-морского института. п. 65. ИСБН 978-1-55750-973-4 . Проверено 14 января 2010 г.
- ^ Перейти обратно: а б Хегарти, Кристофер Дж.; Чатр, Эрик (декабрь 2008 г.). «Эволюция глобальной навигационной спутниковой системы (ГНСС)». Труды IEEE . 96 (12): 1902–1917. дои : 10.1109/JPROC.2008.2006090 . ISSN 0018-9219 . S2CID 838848 .
- ^ «Сотрудник ION - г-н Джон А. Клобушар» . www.ion.org . Архивировано из оригинала 4 октября 2017 года . Проверено 17 июня 2017 г.
- ^ «Наука о GPS-сигналах» . harveycohen.net . Архивировано из оригинала 29 мая 2017 года.
- ^ «ИКАО завершает расследование по установлению фактов» . Международная организация гражданской авиации. Архивировано из оригинала 17 мая 2008 года . Проверено 15 сентября 2008 г.
- ^ «Соединенные Штаты обновляют технологию глобальной системы позиционирования» . America.gov. 3 февраля 2006. Архивировано из оригинала 9 октября 2013 года . Проверено 17 июня 2019 г.
- ^ Румерман, Джуди А. (2009). Сборник исторических данных НАСА, том VII (PDF) . НАСА. п. 136. Архивировано (PDF) из оригинала 25 декабря 2017 года . Проверено 12 июля 2017 г.
- ^ Глобальная система позиционированияОценка национальной политики, Скотт Пейс, Джеральд П. Фрост, Ирвинг Лачоу, Дэвид Р. Фрелингер, Донна Фоссум, Дон Вассем, Моника М. Пинто, Rand Corporation, 1995 г., Приложение B. Архивировано 4 марта 2016 г., в Wayback Machine . История GPS, хронология и бюджеты
- ^ «Вопросы и ответы о GPS и выборочной доступности» (PDF) . НОАА]. Архивировано из оригинала (PDF) 21 сентября 2005 г. Проверено 28 мая 2010 г.
- ^ Стейц, Дэвид Э. «Назван Национальный консультативный совет по позиционированию, навигации и времени» . Архивировано из оригинала 13 января 2010 года . Проверено 22 марта 2007 г.
- ^ GPS Wing достигает рубежа GPS III IBR. Архивировано 23 мая 2013 г., на Wayback Machine в Inside GNSS, 10 ноября 2008 г.
- ^ «Состояние GPS-созвездия на 26.08.2015» . Архивировано из оригинала 5 сентября 2015 года . Проверено 26 августа 2015 г.
- ^ «Краткий обзор: три успешных запуска Atlas 5 за один месяц» . 31 октября 2015 года. Архивировано из оригинала 1 ноября 2015 года . Проверено 31 октября 2015 г.
- ^ «GPS-альманахи» . Navcen.uscg.gov. Архивировано из оригинала 23 сентября 2010 года . Проверено 15 октября 2010 г.
- ^ «Происхождение системы глобального позиционирования (GPS)» . Перемонтируйте систему безопасности . Архивировано из оригинала 11 февраля 2017 года . Проверено 9 февраля 2017 г.
- ^ Дитрих Шрёер; Мирко Елена (2000). Трансфер технологий . Эшгейт. п. 80. ИСБН 978-0-7546-2045-7 . Проверено 25 мая 2008 г.
- ^ Майкл Рассел Рип; Джеймс М. Хасик (2002). Революция точности: GPS и будущее воздушной войны . Издательство Военно-морского института. ISBN 978-1-55750-973-4 . Проверено 25 мая 2008 г.
- ^ Доре, Ричард (16 сентября 1979 г.). «Навстар-Глобальная система предоставит точные данные для навигации» . Ежедневный Бриз . Торранс, Калифорния . п. 91. Архивировано из оригинала 23 мая 2023 года . Проверено 23 мая 2023 г. - через Newspapers.com.
- ^ Перейти обратно: а б Доре, Ричард (16 сентября 1979 г.). «Спутниковые технологии – ключ к GPS» . Ежедневный Бриз . Торранс, Калифорния . п. 97. Архивировано из оригинала 23 мая 2023 года . Проверено 23 мая 2023 г. - через Newspapers.com.
- ^ «Хронология космического командования ВВС» . Космическое командование ВВС США. Архивировано из оригинала 17 августа 2011 года . Проверено 20 июня 2011 г.
- ^ «Информационный бюллетень: 2-я эскадрилья космических операций» . Космическое командование ВВС США. Архивировано из оригинала 11 июня 2011 года . Проверено 20 июня 2011 г.
- ^ Система глобального позиционирования: оценка национальной политики. Архивировано 30 декабря 2015 г., в Wayback Machine , стр. 245. РЭНД корпорация
- ^ Перейти обратно: а б «Система глобального позиционирования USNO NAVSTAR» . Военно-морская обсерватория США. Архивировано из оригинала 26 января 2011 года . Проверено 7 января 2011 г.
- ^ Национальное управление архивов и документации . Политика США в отношении системы глобального позиционирования. Архивировано 6 апреля 2006 г. в Wayback Machine . 29 марта 1996 г.
- ^ «Национальный исполнительный комитет по космическому позиционированию, навигации и времени» . Pnt.gov. Архивировано из оригинала 28 мая 2010 года . Проверено 15 октября 2010 г.
- ^ «Тестовые вызовы с поддержкой GPS для сетей 3G WCDMA» . 3g.co.uk. 10 ноября 2004 года. Архивировано из оригинала 27 ноября 2010 года . Проверено 24 ноября 2010 г.
- ^ «Пресс-релиз: Первый модернизированный спутник GPS, построенный компанией Lockheed Martin, успешно запущен ВВС США - 26 сентября 2005 г.» . Локхид Мартин. Архивировано из оригинала 10 августа 2017 года . Проверено 9 августа 2017 г.
- ^ «losangeles.af.mil» . losangeles.af.mil. 17 сентября 2007 года. Архивировано из оригинала 11 мая 2011 года . Проверено 15 октября 2010 г.
- ^ Джонсон, Бобби (19 мая 2009 г.). «Система GPS «близка к поломке» » . Хранитель . Лондон. Архивировано из оригинала 26 сентября 2013 года . Проверено 8 декабря 2009 г.
- ^ Курси, Дэвид (21 мая 2009 г.). «ВВС реагируют на проблемы с отключением GPS» . Новости АВС . Архивировано из оригинала 23 мая 2009 года . Проверено 22 мая 2009 г.
- ^ Эллиотт, Дэн (1 июня 2010 г.). «Проблема GPS ВВС: сбой показывает, насколько военные США полагаются на GPS» . Хаффингтон Пост . Архивировано из оригинала 11 мая 2011 года . Проверено 15 октября 2010 г.
- ^ «Объявлено о заключении контракта на сегмент GPS-контроля следующего поколения» . База ВВС Лос-Анджелеса . 25 февраля 2010. Архивировано из оригинала 23 июля 2013 года . Проверено 14 декабря 2012 г.
- ^ «Президент объявляет Роджера Истона лауреатом Национальной медали технологий» . ЭврекАлерт! . Лаборатория военно-морских исследований США . 22 ноября 2005 г. Архивировано из оригинала 11 октября 2007 г.
- ^ «Индуктивные технологии / 1998: Система глобального позиционирования (GPS)» . Зал славы космических технологий . Архивировано из оригинала 12 июня 2012 года.
- ^ Уильямс-младший, Ричард А. (5 октября 2011 г.). «Программа GPS получила международную награду» . GPS.gov . Архивировано из оригинала 13 мая 2017 года . Проверено 24 декабря 2018 г.
- ^ «Математик введен в Зал славы пионеров космоса и ракетостроения» . Космическое командование ВВС . 7 декабря 2018 года. Архивировано из оригинала 3 июня 2019 года . Проверено 3 августа 2021 г.
- ^ Амос, Джонатан (12 февраля 2019 г.). «Премия королевы Елизаветы в области инженерии: восхваление пионеров GPS» . Новости Би-би-си . Архивировано из оригинала 6 апреля 2019 года . Проверено 6 апреля 2019 г.
- ^ Нельсон, Джон (19 июня 2019 г.). «Что такое атомные часы?» . НАСА . Архивировано из оригинала 5 апреля 2023 года . Проверено 4 апреля 2023 г.
- ^ «Радиоволна | Примеры, использование, факты и диапазон» . Британника . Проверено 4 апреля 2023 г.
- ^ «JAXA | Позиционирование, чтобы узнать ваше местоположение и время» . global.jaxa.jp . Проверено 4 апреля 2023 г.
- ^ Перейти обратно: а б с д Абель, Дж.С.; Чаффи, JW (1991). «Существование и уникальность GPS-решений». Транзакции IEEE по аэрокосмическим и электронным системам . 27 (6). Институт инженеров по электротехнике и электронике (IEEE): 952–956. Бибкод : 1991ITAES..27..952A . дои : 10.1109/7.104271 . ISSN 0018-9251 .
- ^ Перейти обратно: а б с Фанг, БТ (1992). «Комментарии Дж. С. Абеля и Дж. У. Чаффи к статье «Существование и уникальность решений GPS». Транзакции IEEE по аэрокосмическим и электронным системам . 28 (4). Институт инженеров по электротехнике и электронике (IEEE): 1163. doi : 10.1109/7.165379 . ISSN 0018-9251 .
- ^ Гревал, Мохиндер С.; Вейл, Лоуренс Р.; Эндрюс, Ангус П. (2007). Системы глобального позиционирования, инерциальная навигация и интеграция (2-е изд.). Джон Уайли и сыновья. стр. 92–93 . ISBN 978-0-470-09971-1 .
- ^ Георг цур Бонсен; Дэниел Амманн; Майкл Амманн; Этьен Фави; Паскаль Фламман (1 апреля 2005 г.). «Непрерывная навигация, сочетающая GPS с счислением пути на основе датчиков» . GPS мир. Архивировано из оригинала 11 ноября 2006 года.
- ^ Перейти обратно: а б «Введение в пользовательское оборудование NAVSTAR GPS» (PDF) . Правительство Соединенных Штатов. Архивировано (PDF) из оригинала 10 сентября 2008 г. Проверено 22 августа 2008 г. Глава 7
- ^ «Примечания по поддержке GPS» (PDF) . 19 января 2007 г. Архивировано из оригинала (PDF) 27 марта 2009 г. . Проверено 10 ноября 2008 г.
- ^ Перейти обратно: а б «Глобальная система позиционирования» . GPS.gov. Архивировано из оригинала 30 июля 2010 года . Проверено 26 июня 2010 г.
- ^ Дейли, П. (декабрь 1993 г.). «Навстар GPS и ГЛОНАСС: глобальные спутниковые навигационные системы». Журнал электроники и техники связи . 5 (6): 349–357. дои : 10.1049/ecej:19930069 .
- ^ Дана, Питер Х. (8 августа 1996 г.). «Орбитальные самолеты GPS» . Архивировано из оригинала (GIF) 26 января 2018 года . Проверено 27 февраля 2006 г.
- ^ Обзор GPS из офиса совместной программы NAVSTAR. Архивировано 16 ноября 2007 г. в Wayback Machine . Проверено 15 декабря 2006 г.
- ^ Что глобальная система позиционирования говорит нам о теории относительности. Архивировано 4 января 2007 года в Wayback Machine . Проверено 2 января 2007 г.
- ^ «Созвездие спутников GPS» . gmat.unsw.edu.au. Архивировано из оригинала 22 октября 2011 года . Проверено 27 октября 2011 г.
- ^ «USCG Navcen: Часто задаваемые вопросы по GPS» . Архивировано из оригинала 30 апреля 2011 года . Проверено 31 января 2007 г.
- ^ Перейти обратно: а б Томассен, Кейт. «Как работает GPS» . avionicswest.com. Архивировано из оригинала 30 марта 2016 года . Проверено 22 апреля 2014 г.
- ^ Самама, Нел (2008). Глобальное позиционирование: технологии и производительность . Джон Уайли и сыновья. п. 65 . ISBN 978-0-470-24190-5 . ,
- ^ Агнью, округ Колумбия; Ларсон, К.М. (2007). «Определение времени повторения созвездия GPS». GPS-решения . 11 (1): 71–76. дои : 10.1007/s10291-006-0038-4 . S2CID 59397640 . Эта статья с веб-сайта автора. Архивировано 16 февраля 2008 г. в Wayback Machine , с небольшими исправлениями.
- ^ «Космический сегмент» . GPS.gov. Архивировано из оригинала 18 июля 2019 года . Проверено 27 июля 2019 г.
- ^ Массатт, Пол; Уэйн Брэди (лето 2002 г.). «Оптимизация производительности посредством управления группировкой» (PDF) . Перекрестная связь : 17–21. Архивировано из оригинала 25 января 2012 года.
- ^ Общие новости GPS береговой охраны США 9–9–05
- ↑ USNO Система глобального позиционирования NAVSTAR. Архивировано 8 февраля 2006 г., в Wayback Machine . Проверено 14 мая 2006 г.
- ^ «Решение Министерства обороны вдохнуло новую жизнь в важнейшую спутниковую программу OCX» . Министерство обороны США . Проверено 26 ноября 2023 г.
- ^ «GPS.gov: Система оперативного управления следующего поколения (OCX)» . www.gps.gov . Проверено 26 ноября 2023 г.
- ^ «Спутники GPS-III США» . Ежедневник оборонной промышленности. 13 октября 2011 года. Архивировано из оригинала 18 октября 2011 года . Проверено 27 октября 2011 г.
- ^ «GPS завершает разработку системы оперативного управления нового поколения PDR» . Служба новостей космического командования ВВС. 14 сентября 2011 г. Архивировано из оригинала 2 октября 2011 г.
- ^ «ГЛОБАЛЬНАЯ СИСТЕМА ПОЗИЦИОНИРОВАНИЯ: обновленная оценка расписания может помочь лицам, принимающим решения, устранить вероятные задержки, связанные с новой наземной системой управления» (PDF) . Счетная палата правительства США. Май 2019 г. Архивировано (PDF) из оригинала 10 сентября 2019 г. . Проверено 24 августа 2019 г.
- ^ «GPS-станции Raytheon стоимостью 7 миллиардов долларов работают на 73% выше оценок» . Bloomberg.com . 21 июня 2023 г. . Проверено 26 ноября 2023 г.
- ^ Албон, Кортни (9 июня 2023 г.). «Космические силы видят дальнейшие задержки в работе «проблемного» наземного сегмента GPS» . C4ISRNet . Проверено 26 ноября 2023 г.
- ^ Хитченс, Тереза (7 ноября 2023 г.). «Наземная система GPS следующего поколения, как ожидается, появится в сети этим летом: Calvelli» . Прорыв защиты . Проверено 26 ноября 2023 г.
- ^ «Публикации и стандарты Национальной ассоциации морской электроники (NMEA)» . Национальная ассоциация морской электроники. Архивировано из оригинала 4 августа 2009 года . Проверено 27 июня 2008 г.
- ^ Хадас, Т.; Крипяк-Грегорчик А.; Эрнандес-Пахарес, М.; Каплон, Дж.; Пазиевски Дж.; Вельгош, П.; Гарсия-Риго, А.; Казмирский, К.; Сосница, К.; Квасняк, Д.; Сирни, Дж.; Боси, Дж.; Пуциловский, М.; Шишко, Р.; Портасяк, К.; Оливарес-Пулидо, Г.; Гуляева Т.; Орус-Перес, Р. (ноябрь 2017 г.). «Воздействие и реализация ионосферных эффектов высшего порядка в точных приложениях GNSS: ионосферные эффекты высшего порядка в GNSS». Журнал геофизических исследований: Solid Earth . 122 (11): 9420–9436. дои : 10.1002/2017JB014750 . hdl : 2117/114538 . S2CID 54069697 .
- ^ Сосьница, Кшиштоф; Таллер, Даниэла; Дах, Рольф; Ягги, Адриан; Бойтлер, Герхард (август 2013 г.). «Влияние смещений нагрузки на параметры, полученные от SLR, и на согласованность результатов GNSS и SLR» (PDF) . Журнал геодезии . 87 (8): 751–769. Бибкод : 2013JGeod..87..751S . дои : 10.1007/s00190-013-0644-1 . S2CID 56017067 . Архивировано (PDF) из оригинала 15 марта 2021 г. Проверено 2 марта 2021 г.
- ^ Бери, Грегори; Сосьница, Кшиштоф; Зайдел, Радослав (декабрь 2019 г.). «МультиГНСС-орбита с использованием лазерной локации спутникового определения» . Журнал геодезии . 93 (12): 2447–2463. Бибкод : 2019JGeod..93.2447B . дои : 10.1007/s00190-018-1143-1 .
- ^ «Общий вид передачи времени по GPS» . nist.gov. Архивировано из оригинала 28 октября 2012 года . Проверено 23 июля 2011 г.
- ^ «Использование GPS для улучшения прогнозов тропических циклонов» . ucar.edu . Архивировано из оригинала 28 мая 2015 года . Проверено 28 мая 2015 г.
- ^ Зайдел, Радослав; Сосьница, Кшиштоф; Бери, Грегори; Крыша, Рольф; Прейндж, Ларс; Казмирский, Камиль (январь 2021 г.). «Субсуточное движение полюсов по данным GPS, ГЛОНАСС и Галилео» . Журнал геодезии . 95 (1): 3. Бибкод : 2021JGeod..95....3Z . дои : 10.1007/s00190-020-01453-w . ISSN 0949-7714 .
- ^ Зайдел, Радослав; Сосьница, Кшиштоф; Бури, Гжегож; Дах, Рольф; Пранге, Ларс (июль 2020 г.). «Системные систематические ошибки параметров вращения Земли, полученные по данным GPS, ГЛОНАСС и Галилео» . GPS-решения . 24 (3): 74. Бибкод : 2020GPSS...24...74Z . дои : 10.1007/s10291-020-00989-w .
- ^ Зайдел, Радослав; Сосьница, Кшиштоф; Бури, Гжегож (январь 2021 г.). «Координаты геоцентра, полученные с помощью нескольких GNSS: взгляд на роль моделирования давления солнечной радиации» . GPS-решения . 25 (1): 1. Цифровой код : 2021GPSS...25....1Z . дои : 10.1007/s10291-020-01037-3 .
- ^ Глейзер, Сюзанна; Фриче, Матиас; Сосьница, Кшиштоф; Родригес-Солано, Карлос Хавьер; Ван, Кан; Дах, Рольф; Хугентоблер, Урс; Ротачер, Маркус; Дитрих, Рейнхард (декабрь 2015 г.). «Последовательное сочетание GNSS и SLR с минимальными ограничениями» . Журнал геодезии . 89 (12): 1165–1180. Бибкод : 2015JGeod..89.1165G . дои : 10.1007/s00190-015-0842-0 . S2CID 118344484 .
- ^ Роуз, Маргарет (декабрь 2016 г.). «Что такое геофенсинг (геофенсинг)?» . WhatIs.com . Ньютон, Массачусетс: TechTarget . Проверено 26 января 2020 г.
- ^ Серп, Ян Ван (10 октября 2011 г.). GPS для геодезистов (3-е изд.). Бока-Ратон: CRC Press. дои : 10.4324/9780203305225 . ISBN 978-0-429-14911-5 .
- ^ Веше, Кристина; Эйзен, Олаф; Ортер, Ганс; Шульте, Дэниел; Штайнхаге, Дэниел (январь 2007 г.). «Топография поверхности и ледоход в районе площадки глубокого бурения EDML, Антарктида» . Журнал гляциологии . 53 (182): 442–448. Бибкод : 2007JGlac..53..442W . дои : 10.3189/002214307783258512 . ISSN 0022-1430 .
- ^ Хетарпаул, С.; Чаухан, Р.; Гупта, Словакия; Субраманиам, Л.В.; Намбияр, У. (2011). «Извлечение данных GPS для определения интересных мест». Материалы 8-го международного семинара по интеграции информации в сети .
- ^ Шивалингам, Прахаладхан; Асирватам, Дэвид; Марджани, Мохсен; Сайед Масуд, Джафар Али Ибрагим; Чакраварти, Н.С. Калян; Вирисетти, Гопинатх; Лестари, Марта Три (1 апреля 2024 г.). «Обзор моделей поведения в поездках с использованием набора данных GPS: систематический обзор литературы» . Измерение: Датчики . 32 : 101031. Бибкод : 2024MeasS..3201031S . дои : 10.1016/j.measen.2024.101031 . ISSN 2665-9174 .
- ^ Накадзима, Юу; Сиина, Хиронори; Ямане, Сёхей; Исида, Тору; Ямаки, Хирофуми (январь 2007 г.). «Руководство по эвакуации при стихийных бедствиях: использование многоагентного сервера и мобильных телефонов с GPS» . 2007 Международный симпозиум по приложениям и Интернету . п. 2. дои : 10.1109/SAINT.2007.13 .
- ^ Чжао, Силэй; Сюй, Имин; Ловреглио, Руджеро; Кулиговский, Эрика; Нильссон, Дэниел; Кова, Томас Дж.; Ву, Алекс; Ян, Сян (1 июня 2022 г.). «Оценка решения об эвакуации из-за лесного пожара и времени отбытия с использованием крупномасштабных данных GPS» . Транспортные исследования, часть D: Транспорт и окружающая среда . 107 : 103277. arXiv : 2109.07745 . Бибкод : 2022ТРПД..10703277Z . дои : 10.1016/j.trd.2022.103277 . ISSN 1361-9209 .
- ^ Ян, Чжо; Франц, Марк Л.; Чжу, Шаньцзян; Махмуди, Джина; Насри, Арефе; Чжан, Лэй (1 января 2018 г.). «Анализ спроса на такси в Вашингтоне, округ Колумбия, с использованием GPS и данных о землепользовании» . Журнал транспортной географии . 66 : 35–44. Бибкод : 2018JTGeo..66...35Y . дои : 10.1016/j.jtrangeo.2017.10.021 . ISSN 0966-6923 .
- ^ Браунд, Тейлор А.; Зин, Мэй; Бунстра, Тьерд В.; Вонг, Куинси Джей-Джей; Ларсен, Марк Э.; Кристенсен, Хелен; Тиллман, Габриэль; О'Ди, Бридианна (4 мая 2022 г.). «Данные датчиков смартфонов для выявления и мониторинга симптомов расстройств настроения: продольное наблюдательное исследование» . JMIR психического здоровья . 9 (5): e35549. дои : 10.2196/35549 . ПМЦ 9118091 . ПМИД 35507385 .
- ^ Казмирский, Камиль; Зайдель, Радослав; Сосьница, Кшиштоф (октябрь 2020 г.). «Эволюция орбиты и качества синхронизации для мульти-ГНСС-решений реального времени» . GPS-решения . 24 (4): 111. Бибкод : 2020GPSS...24..111K . дои : 10.1007/s10291-020-01026-6 .
- ^ Стругарек, Дариуш; Сосьница, Кшиштоф; Ягги, Адриан (январь 2019 г.). «Характеристики орбит GOCE на основе спутниковой лазерной локации». Достижения в космических исследованиях . 63 (1): 417–431. Бибкод : 2019AdSpR..63..417S . дои : 10.1016/j.asr.2018.08.033 . S2CID 125791718 .
- ^ Стругарек, Дариуш; Сосьница, Кшиштоф; Арнольд, Дэниел; Ягги, Адриан; Зайдел, Радослав; Бери, Грегори; Дрожджевский, Матеуш (30 сентября 2019 г.). «Определение глобальных геодезических параметров с использованием спутниковых измерений лазерной локации на спутниках Sentinel-3» . Дистанционное зондирование . 11 (19): 2282. Бибкод : 2019RemS...11.2282S . дои : 10.3390/rs11192282 .
- ^ Зайдел, Р.; Сосьница, К.; Дач, Р.; Бери, Г.; Прейндж, Л.; Ягги, А. (июнь 2019 г.). «Сетевые эффекты и обработка движения геоцентра при обработке нескольких GNSS» . Журнал геофизических исследований: Solid Earth . 124 (6): 5970–5989. Бибкод : 2019JGRB..124.5970Z . дои : 10.1029/2019JB017443 .
- ^ Сосьница, Кшиштоф; Таллер, Даниэла; Крыша, Рольф; Штайгенбергер, Питер; Бойтлер, Герхард; Арнольд, Дэниел; Ягги, Адриан (июль 2015 г.). «Спутниковая лазерная дальнометрия GPS и ГЛОНАСС» . Журнал геодезии . 89 (7): 725–743. Бибкод : 2015JGeod..89..725S . дои : 10.1007/s00190-015-0810-8 .
- ^ Бури, Гжегож; Сосьница, Кшиштоф; Зайдел, Радослав; Стругарек, Дариуш; Хугентоблер, Урс (январь 2021 г.). «Определение точных орбит Галилео с использованием комбинированных ГНСС и зеркальных наблюдений» . GPS-решения . 25 (1): 11. Бибкод : 2021GPSS...25...11B . дои : 10.1007/s10291-020-01045-3 .
- ^ Сосьница, К.; Бери, Г.; Зайдел Р. (16 марта 2018 г.). «Вклад группировки мульти-GNSS в наземную систему отсчета, полученную с помощью SLR». Письма о геофизических исследованиях . 45 (5): 2339–2348. Бибкод : 2018GeoRL..45.2339S . дои : 10.1002/2017GL076850 . S2CID 134160047 .
- ^ Сосьница, К.; Бери, Г.; Зайдел, Р.; Стругарек, Д.; Дрожджевский, М.; Казмирски, К. (декабрь 2019 г.). «Оценка глобальных геодезических параметров с использованием зеркальных наблюдений Galileo, ГЛОНАСС, BeiDou, GPS и QZSS» . Земля, планеты и космос . 71 (1): 20. Бибкод : 2019EP&S...71...20S . дои : 10.1186/s40623-019-1000-3 .
- ^ «GPS помогает роботам выполнять работу» . www.asme.org . Архивировано из оригинала 3 августа 2021 года . Проверено 3 августа 2021 г.
- ^ «Использование технологии GPS-слежения в австралийском футболе» . 6 сентября 2012. Архивировано из оригинала 27 сентября 2016 года . Проверено 25 сентября 2016 г.
- ^ «Тихоокеанский северо-западный геодезический массив» . cwu.edu . Архивировано из оригинала 11 сентября 2014 года . Проверено 10 октября 2014 г.
- ^ Ассоциация по контролю над вооружениями. Режим контроля за ракетными технологиями. Архивировано 16 сентября 2008 года в Wayback Machine . Проверено 17 мая 2006 г.
- ^ Синха, Вандана (24 июля 2003 г.). «Командирские и солдатские GPS-приемники» . Gcn.com. Архивировано из оригинала 21 сентября 2009 года . Проверено 13 октября 2009 г.
- ^ «Семейство артиллерийских снарядов Экскалибур». Годовой отчет за 2003 финансовый год (PDF) (Отчет). Директор по эксплуатационным испытаниям и оценке . 2003. с. 69. Архивировано (PDF) из оригинала 18 октября 2021 года . Проверено 23 мая 2023 г.
- ^ «Точные боевые снаряды Excalibur XM982». Годовой отчет за 2010 финансовый год (PDF) (Отчет). Директор по эксплуатационным испытаниям и оценке . Декабрь 2010 г. стр. 65–66. Архивировано (PDF) из оригинала 15 ноября 2022 г. Проверено 23 мая 2023 г.
- ↑ Сандианской национальной лаборатории. Программы нераспространения и технологии контроля над вооружениями Архивировано 28 сентября 2006 г., в Wayback Machine.
- ^ Деннис Д. Маккрейди (август 1994 г.). W-сенсор GPS-детектора всплесков (отчет). Сандианские национальные лаборатории. ОСТИ 10176800 .
- ^ «ВВС США видят изменения в спутниковых программах национальной безопасности» . Aviationweek.com. 18 января 2013. Архивировано из оригинала 22 сентября 2013 года . Проверено 28 сентября 2013 г.
- ^ Гринмайер, Ларри. «GPS и первая в мире «космическая война» » . Научный американец . Архивировано из оригинала 8 февраля 2016 года . Проверено 8 февраля 2016 г.
- ^ «Помехи GPS представляют собой растущую угрозу для спутниковой навигации, позиционирования и точного времени» . www.militaryaerospace.com . 28 июня 2016. Архивировано из оригинала 6 марта 2019 года . Проверено 3 марта 2019 г.
- ^ Брункер, Майк (8 августа 2016 г.). «GPS подвергается атаке: мошенники и рабочие-мошенники ведут электронную войну» . Новости Эн-Би-Си . Архивировано из оригинала 6 марта 2019 года . Проверено 15 декабря 2021 г.
- ^ «Россия подрывает доверие мира к GPS» . 30 апреля 2018 года. Архивировано из оригинала 6 марта 2019 года . Проверено 3 марта 2019 г.
- ^ «Китай глушит GPS американских сил» . 26 сентября 2016. Архивировано из оригинала 6 марта 2019 года . Проверено 3 марта 2019 г.
- ^ Мизоками, Кайл (5 апреля 2016 г.). «Северная Корея глушит сигналы GPS» . Популярная механика . Архивировано из оригинала 6 марта 2019 года . Проверено 3 марта 2019 г.
- ^ «Представитель Ирана подтверждает загадочное нарушение сигналов GPS в Тегеране» . Иранский международный . 29 декабря 2020 года. Архивировано из оригинала 12 июля 2021 года . Проверено 12 июля 2021 г.
- ^ «Факты показывают, что Иран «намеренно» сбил украинский самолет | AvaToday» . 12 июля 2021 года. Архивировано из оригинала 12 июля 2021 года . Проверено 12 июля 2021 г.
- ^ Панелла, Крис (30 апреля 2024 г.). «Российские силы нашли дешевый способ помешать высокоточному оружию США в Украине» . Бизнес-инсайдер .
- ^ «Информационное уведомление для пользователей Navstar (NANU) 2016069» . Операционный центр GPS. Архивировано из оригинала 25 мая 2017 года . Проверено 25 июня 2017 г.
- ^ Дэвид В. Аллан; Нил Эшби; Клиффорд К. Ходж (1997). Наука хронометража (PDF) . Hewlett Packard – через проект памяти HP.
- ^ Питер Х. Дана; Брюс М. Пенрод (июль – август 1990 г.). «Роль GPS в точном распространении времени и частоты» (PDF) . GPS мир . Архивировано (PDF) из оригинала 15 декабря 2012 г. Проверено 27 апреля 2014 г. - через P Dana.
- ^ «Время GPS с точностью до 100 наносекунд» . Галеон. Архивировано из оригинала 14 мая 2012 года . Проверено 12 октября 2012 г.
- ^ Флигель, Генри Ф.; ДиЭспости, Раймонд С. (декабрь 1996 г.), Обзор GPS и теории относительности (PDF) , Эль-Сегундо, Калифорния: Аэрокосмическая корпорация, заархивировано из оригинала (PDF) 6 марта 2023 г. , получено 7 декабря 2022 г.
- ^ Эшби, Нил (2003). «Относительность в системе глобального позиционирования» . Живые обзоры в теории относительности . 6 (1): 1. Бибкод : 2003LRR.....6....1A . дои : 10.12942/lrr-2003-1 . ISSN 1433-8351 . ПМЦ 5253894 . ПМИД 28163638 .
- ^ «GPS.gov: Стандарты производительности и спецификации» . www.gps.gov . Проверено 21 июня 2024 г.
- ^ «Формат спутникового сообщения» . Gpsinformation.net. Архивировано из оригинала 1 ноября 2010 года . Проверено 15 октября 2010 г.
- ^ Питер Х. Дана. «Проблемы переноса номера недели GPS» . Архивировано из оригинала 25 февраля 2013 года . Проверено 12 августа 2013 г.
- ^ «Спецификация интерфейса IS-GPS-200, редакция D: Пользовательские интерфейсы космического сегмента GPS/навигации Navstar» (PDF) . Офис совместной программы Navstar GPS. п. 103. Архивировано из оригинала (PDF) 8 сентября 2012 года.
- ^ Ричхария, Мадхавендра; Уэстбрук, Лесли Дэвид (2011). Спутниковые системы индивидуального применения: концепции и технологии . Джон Уайли и сыновья. п. 443. ИСБН 978-1-119-95610-5 . Архивировано из оригинала 4 июля 2014 года . Проверено 28 февраля 2017 г.
- ^ Перейти обратно: а б с Пенттинен, Юрки Т.Дж. (2015). Справочник по телекоммуникациям: Инженерные рекомендации для фиксированных, мобильных и спутниковых систем . Джон Уайли и сыновья. ISBN 978-1-119-94488-1 .
- ^ Мишра, Пратап; Энге, Пер (2006). Глобальная система позиционирования. Сигналы, измерения и производительность (2-е изд.). Ганга-Джамуна Пресс. п. 115. ИСБН 978-0-9709544-1-1 . Проверено 16 августа 2013 г.
- ^ Борре, Кай; М. Акос, Деннис; Бертельсен, Николай; Риндер, Питер; Йенсен, Сорен Холдт (2007). Программно-определяемый приемник GPS и Galileo. Одночастотный подход . Спрингер. стр. 18. ISBN 978-0-8176-4390-4 .
- ^ «Система обнаружения ядерного взрыва США (USNDS)» . Фас.орг . Архивировано из оригинала 10 октября 2011 года . Проверено 6 ноября 2011 г.
- ^ «Запущен первый GPS-спутник Block 2F, необходимый для предотвращения сбоя системы» . ДейлиТех . Архивировано из оригинала 30 мая 2010 года . Проверено 30 мая 2010 г.
- ^ «United Launch Alliance успешно запускает спутник GPS IIF-12 для ВВС США» . www.ulalaunch.com . Архивировано из оригинала 28 февраля 2018 года . Проверено 27 февраля 2018 г.
- ^ «ВВС успешно передают сигнал L5 со спутника GPS IIR-20(M)» . Пресс-релиз авиабазы Лос-Анджелеса. Архивировано из оригинала 21 мая 2011 года . Проверено 20 июня 2011 г.
- ^ Перейти обратно: а б «Система: тестовые данные предсказывают катастрофические помехи GPS вещательной компанией, уполномоченной FCC» . GPS мир. 1 марта 2011 года. Архивировано из оригинала 11 октября 2011 года . Проверено 6 ноября 2011 г.
- ^ «Коалиция за спасение нашей GPS» . Saveourgps.org. Архивировано из оригинала 30 октября 2011 года . Проверено 6 ноября 2011 г.
- ^ «Тесты LightSquared подтверждают глушение GPS» . Авиационная неделя . Архивировано из оригинала 12 августа 2011 года . Проверено 20 июня 2011 г.
- ^ «Альманахи GPS, NANUS и рекомендации по эксплуатации (включая архивы)» . Информация GPS-альманаха . Береговая охрана США. Архивировано из оригинала 12 июля 2010 года . Проверено 9 сентября 2009 г.
- ^ «Джордж М., Хамид М. и Миллер А. Генераторы кода Голда в устройствах Virtex в Интернет-архиве PDF».
- ^ Перейти обратно: а б раздел 4, начало на странице 15. Джеффри Блюитт: Основы техники GPS. Архивировано 22 сентября 2013 г., в Wayback Machine.
- ^ Перейти обратно: а б с «Системы глобального позиционирования» (PDF) . Архивировано из оригинала (PDF) 19 июля 2011 года . Проверено 15 октября 2010 г.
- ^ Дана, Питер Х. «Геометрическое снижение точности (GDOP) и наглядность» . Университет Колорадо в Боулдере. Архивировано из оригинала 23 августа 2005 года . Проверено 7 июля 2008 г.
- ^ Питер Х. Дана. «Положение приемника, скорость и время» . Университет Колорадо в Боулдере. Архивировано из оригинала 23 августа 2005 года . Проверено 7 июля 2008 г.
- ^ «Современная навигация» . math.nus.edu.sg. Архивировано из оригинала 26 декабря 2017 года . Проверено 4 декабря 2018 г.
- ^ Гилберт Стрэнг; Кай Борре (1997). Линейная алгебра, геодезия и GPS . СИАМ. стр. 448–449. ISBN 978-0-9614088-6-2 . Архивировано из оригинала 10 октября 2021 года . Проверено 22 мая 2018 г.
- ^ Аудун Холм (2010). Геометрия: наше культурное наследие . Springer Science & Business Media. п. 338. ИСБН 978-3-642-14441-7 . Архивировано из оригинала 10 октября 2021 года . Проверено 22 мая 2018 г.
- ^ Перейти обратно: а б Б. Хофманн-Велленхоф; К. Легат; М. Визер (2003). Навигация . Springer Science & Business Media. п. 36. ISBN 978-3-211-00828-7 . Архивировано из оригинала 10 октября 2021 года . Проверено 22 мая 2018 г.
- ^ Гроувс, П.Д. (2013). Принципы ГНСС, инерциальных и мультисенсорных интегрированных навигационных систем, второе издание . ГНСС/GPS. Артех Хаус. ISBN 978-1-60807-005-3 . Архивировано из оригинала 15 марта 2021 года . Проверено 19 февраля 2021 г.
- ^ Хошен Дж (1996). «Уравнения GPS и проблема Аполлония». Транзакции IEEE по аэрокосмическим и электронным системам . 32 (3): 1116–1124. Бибкод : 1996ITAES..32.1116H . дои : 10.1109/7.532270 . S2CID 30190437 .
- ^ Графаренд, Эрик В. (2002). «Решения GPS: закрытые формы, критические и специальные конфигурации P4P». GPS-решения . 5 (3): 29–41. Бибкод : 2002GPSS....5...29G . дои : 10.1007/PL00012897 . S2CID 121336108 .
- ^ Перейти обратно: а б Бэнкрофт, С. (январь 1985 г.). «Алгебраическое решение уравнений GPS». Транзакции IEEE по аэрокосмическим и электронным системам . АЭС-21(1): 56–59. Бибкод : 1985ITAES..21...56B . дои : 10.1109/TAES.1985.310538 . S2CID 24431129 .
- ^ Чаффи, Дж. и Абель, Дж., «О точных решениях уравнений псевдодальности», IEEE Transactions on Aerospace and Electronic Systems , том: 30, №: 4, стр: 1021–1030, 1994.
- ^ Сирола, Ниило (март 2010 г.). «Алгоритмы закрытой формы в мобильном позиционировании: мифы и заблуждения». 7-й семинар по позиционированию, навигации и связи . WPNC 2010. стр. 38–44. CiteSeerX 10.1.1.966.9430 . дои : 10.1109/WPNC.2010.5653789 .
- ^ «Подходы к позиционированию GNSS». Подходы к позиционированию GNSS – Спутниковая съемка GPS, четвертое издание – Leick . Интернет-библиотека Уайли. 2015. С. 257–399. дои : 10.1002/9781119018612.ch6 . ISBN 9781119018612 .
- ^ Альфред Клейсберг, «Аналитическое решение GPS-навигации», Сборник исследований Штутгартского университета , 1994 г.
- ^ Ощак, Б., «Новый алгоритм позиционирования GNSS с использованием системы линейных уравнений», Материалы 26-го международного технического совещания спутникового отдела Института навигации (ION GNSS + 2013) , Нэшвилл, Теннесси, сентябрь 2013 г., стр. 3560–3563.
- ^ Аттевилл, Фред. (13 февраля 2013 г.) Транспортные средства, использующие глушители GPS, представляют большую угрозу для самолетов. Архивировано 16 февраля 2013 г. в Wayback Machine . Metro.co.uk. Проверено 2 августа 2013 г.
- ^ «Часто задаваемые вопросы об выборочной доступности» . Национальное координационное бюро космического позиционирования, навигации и времени (PNT). Октябрь 2001. Архивировано из оригинала 16 июня 2015 года . Проверено 13 июня 2015 г.
Выборочная доступность закончилась через несколько минут после полуночи по восточному времени после окончания 1 мая 2000 года. Изменение произошло одновременно во всей группировке спутников.
- ^ «Доска» (PDF) .
- ^ «Руководство по эксплуатации John Deere StarFire 3000 2011 г.» (PDF) . Джон Дир. Архивировано из оригинала (PDF) 5 января 2012 года . Проверено 13 ноября 2011 г.
- ^ Перейти обратно: а б «Отчет и приказ Федеральной комиссии по связи в отношении фиксированных и мобильных служб в диапазонах мобильной спутниковой службы 1525–1559 МГц и 1626,5–1660,5 МГц» (PDF) . Федеральная комиссия по связи. 6 апреля 2011 г. Архивировано из оригинала (PDF) 16 декабря 2011 г. . Проверено 13 декабря 2011 г.
- ^ «Таблица распределения частот Федеральной комиссии по связи» (PDF) . Федеральная комиссия по связи. 18 ноября 2011 г. Архивировано (PDF) из оригинала 16 декабря 2011 г. . Проверено 13 декабря 2011 г.
- ^ «Номер файла в реестре FCC: SATASG2001030200017, «Заявление компании Mobile Satellite Ventures LLC на присвоение и изменение лицензий, а также на получение разрешения на запуск и эксплуатацию мобильной спутниковой системы нового поколения » . Федеральная комиссия по связи. 1 марта 2001. с. 9. Архивировано из оригинала 14 января 2012 года . Проверено 14 декабря 2011 г.
- ^ «Петиция Промышленного совета GPS США в Федеральную комиссию по связи о принятии ограничений OOBE, совместно предложенная MSV и Промышленным советом» . Федеральная комиссия по связи. 4 сентября 2003 года . Проверено 13 декабря 2011 г. [ мертвая ссылка ]
- ^ Перейти обратно: а б «Приказ о пересмотре» (PDF) . 3 июля 2003 г. Архивировано (PDF) из оригинала 20 октября 2011 г. . Проверено 20 октября 2015 г.
- ^ «Заявление Юлиуса П. Кнаппа, руководителя Управления инженерии и технологий Федеральной комиссии по связи» (PDF) . gps.gov. 15 сентября 2011 г. с. 3. Архивировано (PDF) из оригинала 16 декабря 2011 г. Проверено 13 декабря 2011 г.
- ^ «Приказ FCC предоставил дочерней компании LightSquared LLC, лицензиату мобильной спутниковой службы в L-диапазоне, условный отказ от правила «интегрированной службы» вспомогательного наземного компонента» (PDF) . Федеральная комиссия по связи . Федеральная комиссия по связи (FCC) 26 января 2011 г. Архивировано (PDF) из оригинала 16 декабря 2011 г. . Проверено 13 декабря 2011 г.
- ^ «Вебинар Javad Ashjaee GPS World» . gpsworld.com. 8 декабря 2011. Архивировано из оригинала 26 ноября 2011 года . Проверено 13 декабря 2011 г.
- ^ «Приказ FCC, разрешающий поставщикам мобильных спутниковых услуг предоставлять вспомогательный наземный компонент (ATC) для своих спутниковых систем» (PDF) . Федеральная комиссия по связи . 10 февраля 2003 г. Архивировано (PDF) из оригинала 16 декабря 2011 г. . Проверено 13 декабря 2011 г.
- ^ «Федеральная комиссия связи по услугам фиксированной и подвижной связи в мобильной спутниковой службе» . Федеральная комиссия по связи . 15 июля 2010 года. Архивировано из оригинала 27 мая 2012 года . Проверено 13 декабря 2011 г.
- ^ [1] Архивировано 13 декабря 2012 г., в Wayback Machine.
- ^ «Коалиция за спасение нашей GPS» . Saveourgps.org. Архивировано из оригинала 24 октября 2011 года . Проверено 6 ноября 2011 г.
- ^ Джефф Карлайл (23 июня 2011 г.). «Свидетельство Джеффа Карлайла, исполнительного вице-президента LightSquared по вопросам регулирования и государственной политики в Подкомитете Палаты представителей США по авиации и Подкомитете по береговой охране и морскому транспорту» (PDF) . Архивировано из оригинала (PDF) 29 сентября 2011 года . Проверено 13 декабря 2011 г.
- ^ Юлиус Генаховский (31 мая 2011 г.). «Письмо председателя FCC Генаховски сенатору Чарльзу Грассли» (PDF) . Архивировано из оригинала (PDF) 13 января 2012 года . Проверено 13 декабря 2011 г.
- ^ Перейти обратно: а б Тесслер, Джоэль (7 апреля 2011 г.). «Интернет-сеть может блокировать работу GPS в автомобилях и самолетах» . Новости Солнца . Архивировано из оригинала 1 мая 2011 года . Проверено 7 апреля 2011 г.
- ^ Пресс-релиз FCC «Заявление представителя по поводу письма NTIA – LightSquared и GPS». Архивировано 23 апреля 2012 г., в Wayback Machine . 14 февраля 2012 г. По состоянию на 3 марта 2013 г.
- ^ Пол Риглер, FBT. «План широкополосной сети FCC Bars LightSquared». Архивировано 22 сентября 2013 г. в Wayback Machine . 14 февраля 2012 г. Проверено 14 февраля 2012 г.
- ^ «Россия запустила еще три космических аппарата ГЛОНАСС-М» . Внутри ГНСС . Архивировано из оригинала 6 февраля 2009 года . Проверено 26 декабря 2008 г.
- ^ Джон (10 января 2012 г.). «ГЛОНАСС – будущее всех смартфонов?» . Блог о гвоздике . Архивировано из оригинала 10 марта 2016 года . Проверено 29 октября 2016 г.
- ^ Хведчук, Катажина; Циенкош, Даниэль; Аполлон, Михал; Боровский, Лукаш; Левинска, Паулина; Гимарайнш Сантуш, Селсу Аугусто; Эборка, Кеннеди; Кулшрешта, Сандип; Ромеро-Андраде, Розендо; Седик, Ахмед; Любуск, Айве; Малюк, Камил (2022). «Задачи, связанные с определением высот горных вершин, представленных по картографическим источникам» . Геодетский вестник . 66 : 49–59. doi : 10.15292/geodetski-vestnik.2022.01.49-59 . S2CID 247985456 .
- ^ «Китай запускает последний спутник в системе Beidou, подобной GPS» . физ.орг . Ассошиэйтед Пресс. 23 июня 2020 года. Архивировано из оригинала 24 июня 2020 года . Проверено 24 июня 2020 г.
- ^ «Навигационная спутниковая система Galileo заработала» . dw.com. Архивировано из оригинала 18 октября 2017 года . Проверено 17 декабря 2016 г.
- ^ Крининг, Торстен (23 января 2019 г.). «Япония готовится к отказу GPS со спутниками квазизенита» . SpaceWatch.Global . Архивировано из оригинала 19 апреля 2019 года . Проверено 10 августа 2019 г.
Дальнейшее чтение
[ редактировать ]- «Введение в пользовательское оборудование NAVSTAR GPS» (PDF) . Береговая охрана США. Сентябрь 1996 г. Архивировано из оригинала (PDF) 21 октября 2013 г. Проверено 22 августа 2008 г.
- Паркинсон; Спилкер (1996). Глобальная система позиционирования . Американский институт аэронавтики и астронавтики. ISBN 978-1-56347-106-3 .
- Джаизки Мендизабал; Рок Беренгер; Хуан Мелендес (2009). GPS и Галилео . МакГроу Хилл. ISBN 978-0-07-159869-9 .
- Натаниэль Боудич (2002). . Правительство Соединенных Штатов.
- Открытые курсы по системе глобального позиционирования от Массачусетского технологического института , 2012 г.
- Грег Милнер (2016). Pinpoint: Как GPS меняет технологии, культуру и наше мышление . WW Нортон. ISBN 978-0-393-08912-7 .
Внешние ссылки
[ редактировать ]
- Часто задаваемые вопросы по GPS ФАУ
- GPS.gov – веб-сайт общего образования, созданный правительством США.