Морская жизнь
![]() | Эта статья содержит слишком много изображений для ее общего объема . ( декабрь 2023 г. ) |
Часть серии обзоров по |
Морская жизнь |
---|
![]() |


Морская жизнь , морская жизнь или жизнь океана — это растения , животные и другие организмы , которые живут в соленой воде морей океанов или в или солоноватой воде прибрежных эстуариев . На фундаментальном уровне морская жизнь влияет на природу планеты. Морские организмы, в основном микроорганизмы , производят кислород и связывают углерод . Морская жизнь частично формирует и защищает береговую линию, а некоторые морские организмы даже помогают создавать новые суши (например, рифы ) коралловые .
Большинство форм жизни первоначально развилось в морской среде обитания . По объему океаны обеспечивают около 90% жизненного пространства на планете. [ 2 ] Первые позвоночные появились в виде рыб . [ 3 ] которые живут исключительно в воде. Некоторые из них превратились в амфибий , которые проводят часть своей жизни в воде, а часть — на суше. Одна группа амфибий превратилась в рептилий и млекопитающих, а несколько подгрупп каждой из них вернулись в океан в виде морских змей , морских черепах , тюленей , ламантинов и китов . Растительные формы, такие как ламинария и другие водоросли, растут в воде и являются основой некоторых подводных экосистем. Планктон океана образует общую основу пищевой цепи , особенно фитопланктон , который является ключевыми первичными продуцентами .
Морские беспозвоночные демонстрируют широкий спектр модификаций, позволяющих выжить в водах с низким содержанием кислорода, включая дыхательные трубки, такие как сифоны моллюсков . У рыб есть жабры вместо легких , хотя у некоторых видов рыб, например двоякодышащих , есть и то, и другое. Морским млекопитающим (например, дельфинам, китам, выдрам и тюленям) необходимо периодически всплывать на поверхность, чтобы подышать воздухом.
По состоянию на 2023 год [update]было зарегистрировано более 242 000 морских видов , и, возможно, два миллиона морских видов еще не зарегистрированы. В среднем описывается 2332 новых вида в год. [ 4 ] [ 5 ]
Размеры морских видов варьируются от микроскопических, таких как фитопланктон , размер которых может достигать 0,02 микрометра , до огромных китообразных, таких как синий кит – самое большое известное животное, достигающее 33 м (108 футов) в длину. [ 6 ] [ 7 ] Морские микроорганизмы, включая протистов , бактерии и связанные с ними вирусы , по разным оценкам составляют около 70% [ 8 ] или около 90% [ 9 ] [ 1 ] от общей морской биомассы . Морская жизнь изучается с научной точки зрения как в морской биологии , так и в биологической океанографии . Термин «морской» происходит от латинского mare , что означает «море» или «океан».
Вода
[ редактировать ]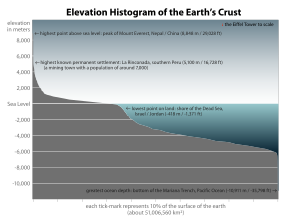
Без воды нет жизни. [ 10 ] Его называют универсальным растворителем из-за его способности растворять многие вещества. [ 11 ] [ 12 ] и как растворитель жизни . [ 13 ] Вода — единственное общее вещество, которое существует в твердом , жидком и газообразном состоянии в условиях, нормальных для жизни на Земле. [ 14 ] Лауреат Нобелевской премии Альберт Сент - Дьёрдьи называл воду «матерью и матрицей» : матерью и лоном жизни. [ 15 ]
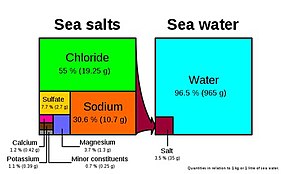
Обилие поверхностных вод на Земле является уникальной особенностью Солнечной системы . Земли Гидросфера состоит в основном из океанов, но технически включает в себя все водные поверхности мира, включая внутренние моря, озера, реки и подземные воды на глубину до 2000 метров (6600 футов). Самое глубокое подводное место — это Бездна Челленджера в Марианской впадине в Тихом океане , глубина которой составляет 10 900 метров (6,8 миль). [ примечание 1 ] [ 16 ]
Условно планета разделена на пять отдельных океанов, однако все эти океаны соединяются в единый мировой океан . [ 17 ] Масса этого мирового океана составляет 1,35 × 10 18 метрические тонны или около 1/4400 общей массы Земли. Мировой океан занимает площадь 3,618 × 10. 8 км 2 со средней глубиной 3682 м , что дает расчетный объем 1,332 × 10 9 км 3 . [ 18 ] Если бы вся поверхность земной коры находилась на одной высоте с гладкой сферой, глубина образовавшегося мирового океана составила бы около 2,7 километров (1,7 мили). [ 19 ] [ 20 ]

Около 97,5% воды на Земле солёная ; оставшиеся 2,5% — пресная вода . Большая часть пресной воды – около 69% – присутствует в виде льда в ледяных шапках и ледниках . [ 21 ] Средняя соленость океанов Земли составляет около 35 граммов (1,2 унции) соли на килограмм морской воды (3,5% соли). [ 22 ] Большая часть соли в океане образуется в результате выветривания и эрозии горных пород на суше. [ 23 ] Некоторые соли выделяются в результате вулканической деятельности или добываются из холодных магматических пород . [ 24 ]
Океаны также являются резервуаром растворенных атмосферных газов, которые необходимы для выживания многих водных форм жизни. [ 25 ] Морская вода оказывает важное влияние на мировой климат, а океаны служат большим резервуаром тепла . [ 26 ] Сдвиги в распределении температуры океана могут вызвать значительные изменения погоды, такие как Эль-Ниньо и Южное колебание . [ 27 ]

Всего океан занимает 71 процент мировой поверхности. [ 2 ] в среднем около 3,7 км (2,3 мили) в глубину. [ 28 ] По объему океан обеспечивает около 90 процентов жизненного пространства на планете. [ 2 ] Писатель-фантаст Артур Кларк отметил, что было бы более уместно называть планету Земля планетой Океан. [ 29 ] [ 30 ]
Однако вода встречается и в других частях Солнечной системы. Европа , одна из лун, вращающихся вокруг Юпитера , немного меньше земной Луны . Существует большая вероятность того, что под его ледяной поверхностью существует большой соленый океан. [ 31 ] Подсчитано, что внешняя кора твердого льда имеет толщину около 10–30 км (6–19 миль), а глубина жидкого океана под ней составляет около 100 км (60 миль). [ 32 ] Это сделало бы океан Европы в два раза больше земного океана. Высказывались предположения, что океан Европы может поддерживать жизнь . [ 33 ] [ 34 ] и может быть способен поддерживать многоклеточные микроорганизмы , если гидротермальные источники . на дне океана активны [ 35 ] Энцелад , небольшой ледяной спутник Сатурна, также имеет подземный океан , который активно выбрасывает теплую воду с поверхности Луны. [ 36 ]
Эволюция
[ редактировать ]−4500 — – — – −4000 — – — – −3500 — – — – −3000 — – — – −2500 — – — – −2000 — – — – −1500 — – — – −1000 — – — – −500 — – — – 0 — |
| |||||||||||||||||||||||||||||||||||||||||||||
Историческое развитие
[ редактировать ]Земле . около 4,54 миллиарда лет [ 37 ] [ 38 ] [ 39 ] Самые ранние неоспоримые доказательства существования жизни на Земле датируются по меньшей мере 3,5 миллиарда лет назад. [ 40 ] [ 41 ] во время Эоархейской эры после того, как геологическая кора начала затвердевать после более раннего расплавленного Гадейского эона. микробного мата Окаменелости возрастом 3,48 миллиарда лет были обнаружены в песчанике в Западной Австралии . [ 42 ] [ 43 ] Другим ранним физическим свидетельством биогенного вещества является графит возрастом 3,7 миллиарда лет, в метаосадочных породах обнаруженных в Западной Гренландии. [ 44 ] а также «остатки биотической жизни », обнаруженные в скалах возрастом 4,1 миллиарда лет в Западной Австралии. [ 45 ] [ 46 ] По мнению одного из исследователей, «Если бы жизнь возникла на Земле относительно быстро… то она могла бы быть обычным явлением во Вселенной ». [ 45 ]
Все организмы на Земле произошли от общего предка или генофонда предков . [ 47 ] [ 48 ] Считается, что высокоэнергетическая химия создала самовоспроизводящуюся молекулу около 4 миллиардов лет назад, а полмиллиарда лет спустя существовал последний общий предок всей жизни . [ 49 ] В настоящее время научный консенсус заключается в том, что сложная биохимия, из которой состоит жизнь, возникла в результате более простых химических реакций. [ 50 ] Начало жизни могло включать в себя самовоспроизводящиеся молекулы, такие как РНК. [ 51 ] и сборка простых клеток. [ 52 ] В 2016 году ученые сообщили о наборе из 355 генов от последнего универсального общего предка (LUCA) всей жизни , включая микроорганизмы, живущие на Земле . [ 53 ]
Современные виды представляют собой этап процесса эволюции, а их разнообразие является продуктом длинной серии событий видообразования и вымирания. [ 54 ] Общее происхождение организмов было впервые выведено из четырех простых фактов об организмах: во-первых, они имеют географическое распространение, которое нельзя объяснить местной адаптацией. Во-вторых, разнообразие жизни – это не набор уникальных организмов, а организмы, имеющие морфологическое сходство . В-третьих, рудиментарные черты без четкой цели напоминают функциональные наследственные черты и, наконец, на основе этих сходств организмы можно классифицировать в иерархию вложенных групп, подобную генеалогическому древу. [ 55 ] Однако современные исследования показали, что из-за горизонтального переноса генов это «дерево жизни» может быть более сложным, чем простое ветвящееся дерево, поскольку некоторые гены распространились независимо между отдаленно родственными видами. [ 56 ] [ 57 ]
Предыдущие виды также оставили записи своей эволюционной истории. Окаменелости, наряду со сравнительной анатомией современных организмов, составляют морфологическую или анатомическую летопись. [ 58 ] Сравнивая анатомию современных и вымерших видов, палеонтологи могут сделать вывод о происхождении этих видов. Однако этот подход наиболее успешен для организмов, у которых были твердые части тела, такие как раковины, кости или зубы. Кроме того, поскольку прокариоты, такие как бактерии и археи, имеют ограниченный набор общих морфологий, их окаменелости не предоставляют информации об их происхождении.

Совсем недавно доказательства общего происхождения были получены в результате изучения биохимического сходства между организмами. Например, все живые клетки используют один и тот же базовый набор нуклеотидов и аминокислот . [ 60 ] Развитие молекулярной генетики выявило записи эволюции, оставшиеся в геномах организмов: датирование момента, когда виды разошлись по молекулярным часам , вызванным мутациями. [ 61 ] Например, сравнение последовательностей ДНК показало, что люди и шимпанзе имеют общие геномы на 98%, а анализ тех немногих областей, где они различаются, помогает пролить свет на то, когда существовал общий предок этих видов. [ 62 ]
Прокариоты населяли Землю примерно 3–4 миллиарда лет назад. [ 63 ] [ 64 ] Никаких очевидных изменений в морфологии или клеточной организации у этих организмов в течение следующих нескольких миллиардов лет не произошло. [ 65 ] Эукариотические клетки возникли между 1,6 и 2,7 миллиарда лет назад. Следующее серьезное изменение в клеточной структуре произошло, когда бактерии были поглощены эукариотическими клетками, образовав кооперативную ассоциацию, называемую эндосимбиозом . [ 66 ] [ 67 ] Поглощенные бактерии и клетка-хозяин затем подверглись совместной эволюции, при этом бактерии эволюционировали либо в митохондрии, либо в гидрогеносомы . [ 68 ] Очередное поглощение цианобактериоподобных организмов привело к образованию хлоропластов у водорослей и растений. [ 69 ]

История жизни была историей одноклеточных период в океанах начали появляться многоклеточные организмы эукариот, прокариотов и архей примерно до 610 миллионов лет назад, когда в эдиакарский . [ 63 ] [ 70 ] Эволюция многоклеточности происходила в ходе множества независимых событий в таких разнообразных организмах, как губки , бурые водоросли , цианобактерии , слизевики и миксобактерии . [ 71 ] В 2016 году ученые сообщили, что около 800 миллионов лет назад незначительное генетическое изменение в одной молекуле под названием GK-PID, возможно, позволило организмам перейти от одноклеточного организма к одной из многих клеток. [ 72 ]
Вскоре после появления этих первых многоклеточных организмов в течение примерно 10 миллионов лет появилось значительное биологическое разнообразие в результате события, названного Кембрийским взрывом . Здесь в палеонтологической летописи появилось большинство типов современных животных, а также уникальные линии, впоследствии вымершие. [ 73 ] Были предложены различные причины кембрийского взрыва, в том числе накопление кислорода в атмосфере в результате фотосинтеза. [ 74 ]
Около 500 миллионов лет назад растения и грибы начали колонизировать землю. Свидетельства появления первых наземных растений встречаются в ордовике , около 450 миллионов лет назад , в виде ископаемых спор. [ 75 ] Наземные растения начали диверсифицироваться в позднем силуре , примерно 430 миллионов лет назад . [ 76 ] За колонизацией суши растениями вскоре последовали членистоногие и другие животные. [ 77 ] Насекомые добились особенного успеха и даже сегодня составляют большинство видов животных. [ 78 ] Земноводные впервые появились около 364 миллионов лет назад, за ними последовали ранние амниоты и птицы около 155 миллионов лет назад (оба из « рептилийных » линий), млекопитающие около 129 миллионов лет назад, гоминины около 10 миллионов лет назад и современные люди около 250 000 лет назад. назад. [ 79 ] [ 80 ] [ 81 ] Однако, несмотря на эволюцию этих крупных животных, более мелкие организмы, подобные тем типам, которые развились на ранних этапах этого процесса, продолжают быть весьма успешными и доминировать на Земле, причем большая часть как биомассы, так и видов является прокариотами. [ 82 ]
По оценкам, численность современных видов на Земле колеблется от 10 до 14 миллионов. [ 83 ] из них около 1,2 миллиона задокументированы, а более 86 процентов еще не описаны. [ 84 ]
Микроорганизмы
[ редактировать ]Микроорганизмы составляют около 70% морской биомассы . [ 8 ] Микроорганизм слишком маленький , или микроб — это микроскопический организм, чтобы его можно было распознать невооруженным глазом. Это может быть одноклеточный [ 85 ] или многоклеточные . Микроорганизмы разнообразны и включают все бактерии и археи , большинство простейших, таких как водоросли , грибы и некоторых микроскопических животных, таких как коловратки .
Многие макроскопические животные и растения имеют микроскопические ювенильные стадии . Некоторые микробиологи также относят вирусы (и вироиды ) к микроорганизмам, но другие считают их неживыми. [ 86 ] [ 87 ]
Микроорганизмы играют решающую роль в переработке питательных веществ в экосистемах, поскольку они действуют как разлагатели . Некоторые микроорганизмы являются патогенными , вызывая заболевания и даже смерть растений и животных. [ 88 ] Будучи обитателями крупнейшей окружающей среды на Земле, морские микробные системы вызывают изменения во всех глобальных системах. Микробы ответственны практически за весь фотосинтез , происходящий в океане, а также за круговорот углерода , азота , фосфора , других питательных веществ и микроэлементов. [ 89 ]
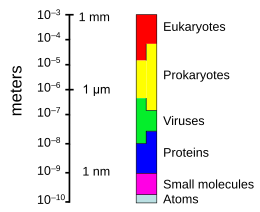

Подводная микроскопическая жизнь разнообразна и до сих пор плохо изучена, например, роль вирусов в морских экосистемах. [ 90 ] Большинство морских вирусов представляют собой бактериофаги , которые безвредны для растений и животных, но необходимы для регулирования морских и пресноводных экосистем. [ 91 ] : 5 Они заражают и уничтожают бактерии в водных микробных сообществах и являются важнейшим механизмом переработки углерода в морской среде. Органические молекулы, высвобождаемые из мертвых бактериальных клеток, стимулируют рост новых бактерий и водорослей. [ 91 ] : 593 Вирусная активность также может способствовать биологическому насосу — процессу, посредством которого в глубинах углерод улавливается океана. [ 92 ]


Поток переносимых по воздуху микроорганизмов кружит над планетой над погодными системами, но под коммерческими воздушными путями. [ 93 ] Некоторые странствующие микроорганизмы переносятся земными пыльными бурями, но большинство происходит из морских микроорганизмов, содержащихся в морских брызгах . В 2018 году ученые сообщили, что сотни миллионов вирусов и десятки миллионов бактерий ежедневно оседают на каждом квадратном метре планеты. [ 94 ] [ 95 ]
Микроскопические организмы обитают по всей биосфере . Масса прокариотных микроорганизмов, в которую входят бактерии и археи, но не ядросодержащие эукариотные микроорганизмы , может достигать 0,8 триллиона тонн углерода (от общей массы биосферы , оцениваемой от 1 до 4 триллионов тонн). [ 96 ] Одноклеточные барофильные морские микробы были обнаружены на глубине 10 900 м (35 800 футов) в Марианской впадине , самом глубоком месте мирового океана. [ 97 ] [ 98 ] Микроорганизмы живут внутри скал на глубине 580 м (1900 футов) ниже морского дна, на глубине 2590 м (8500 футов) океана у побережья северо-запада США. [ 97 ] [ 99 ] а также на глубине 2400 м (7900 футов; 1,5 мили) под морским дном у берегов Японии. [ 100 ] Самая высокая известная температура, при которой может существовать микробная жизнь, составляет 122 ° C (252 ° F) ( Methanopyrus kandleri ). [ 101 ] В 2014 году учёные подтвердили существование микроорганизмов, живущих на глубине 800 м (2600 футов) подо льдом Антарктиды . [ 102 ] [ 103 ] По словам одного исследователя: «Микробы можно найти повсюду — они чрезвычайно адаптируются к условиям и выживают, где бы они ни находились». [ 97 ]
Морские вирусы
[ редактировать ]Вирусы — мелкие инфекционные агенты , не имеющие собственного метаболизма и способные размножаться только внутри живых клеток других организмов . [ 104 ] Вирусы могут инфицировать все виды форм жизни , от животных и растений до микроорганизмов , включая бактерии и археи . [ 105 ] Линейный размер среднего вируса составляет примерно одну сотую размера средней бактерии . Большинство вирусов невозможно увидеть в оптический микроскоп , поэтому электронные микроскопы . вместо него используются [ 106 ]
Вирусы встречаются везде, где есть жизнь, и, вероятно, существовали с момента появления живых клеток. [ 107 ] Происхождение вирусов неясно, поскольку они не образуют окаменелостей, поэтому молекулярные методы использовались для сравнения ДНК или РНК вирусов и являются полезным средством изучения того, как они возникают. [ 108 ]
Сегодня вирусы признаны древними и имеют происхождение, предшествовавшее расколу жизни на три домена . [ 109 ] Но происхождение вирусов в эволюционной истории жизни неясно: некоторые, возможно, произошли от плазмид — фрагментов ДНК, которые могут перемещаться между клетками, — тогда как другие, возможно, произошли от бактерий. В эволюции вирусы являются важным средством горизонтального переноса генов , увеличивающего генетическое разнообразие . [ 110 ]

Мнения относительно того, являются ли вирусы формой жизни или органическими структурами, взаимодействующими с живыми организмами, расходятся. [ 111 ] Некоторые считают их формой жизни, поскольку они несут генетический материал, размножаются, создавая множество своих копий посредством самосборки, и развиваются посредством естественного отбора . Однако им не хватает ключевых характеристик, таких как клеточная структура, которая обычно считается необходимой для того, чтобы считаться жизнью. Поскольку вирусы обладают некоторыми, но не всеми такими качествами, их называют репликаторами. [ 111 ] и как «организмы на грани жизни». [ 112 ]

Бактериофаги , часто называемые просто фагами , представляют собой вирусы, паразитирующие на бактериях и археях. Морские фаги паразитируют на морских бактериях и археях, таких как цианобактерии . [ 113 ] Они представляют собой распространенную и разнообразную группу вирусов и являются наиболее распространенным биологическим объектом в морской среде, поскольку их хозяева, бактерии, обычно представляют собой численно доминирующую клеточную жизнь в море. Обычно в каждом мл морской воды содержится от 1 до 10 миллионов вирусов, или примерно в десять раз больше вирусов с двухцепочечной ДНК, чем клеточных организмов. [ 114 ] [ 115 ] хотя оценки численности вирусов в морской воде могут варьироваться в широком диапазоне. [ 116 ] [ 117 ] Хвостатые бактериофаги, по-видимому, доминируют в морских экосистемах по количеству и разнообразию организмов. [ 113 ] Бактериофаги, принадлежащие к семействам Corticoviridae , [ 118 ] Иновириды [ 119 ] и микровирусы [ 120 ] Также известно, что они заражают разнообразные морские бактерии.
Микроорганизмы составляют около 70% морской биомассы. [ 8 ] По оценкам, вирусы убивают 20% этой биомассы каждый день, а в океанах вирусов в 15 раз больше, чем бактерий и архей. Вирусы являются основными агентами, ответственными за быстрое уничтожение вредоносного цветения водорослей . [ 115 ] которые часто убивают других морских обитателей. [ 121 ] Число вирусов в океанах уменьшается по мере удаления от берега и глубже в воду, где меньше организмов-хозяев. [ 92 ]
Существуют также архейные вирусы , которые реплицируются внутри архей : это вирусы с двухцепочечной ДНК необычной, а иногда и уникальной формы. [ 122 ] [ 123 ] Наиболее подробно эти вирусы изучены на термофильных археях, в частности на порядках Sulfolobales и Thermoproteales . [ 124 ]
Вирусы являются важным естественным средством передачи генов между различными видами, что увеличивает генетическое разнообразие и стимулирует эволюцию. [ 110 ] Считается, что вирусы играли центральную роль в ранней эволюции, до появления бактерий, архей и эукариот, во времена последнего универсального общего предка жизни на Земле. [ 125 ] Вирусы по-прежнему являются одним из крупнейших резервуаров неизведанного генетического разнообразия на Земле. [ 92 ]
Морские бактерии
[ редактировать ]

Бактерии обширную область прокариотических микроорганизмов составляют . Обычно бактерии имеют длину несколько микрометров и имеют разную форму: от сфер до палочек и спиралей. Бактерии были одними из первых форм жизни, появившихся на Земле , и присутствуют в большинстве ее сред обитания . Бактерии обитают в почве, воде, кислых горячих источниках , радиоактивных отходах . [ 126 ] и глубокие части земной коры . Бактерии также живут в симбиотических и паразитических отношениях с растениями и животными.
Когда-то бактерии считались растениями класса шизомицетов , а теперь их относят к прокариотам . В отличие от клеток животных и других эукариот , бактериальные клетки не содержат ядра и редко содержат мембраносвязанные органеллы . Хотя термин «бактерии» традиционно включал в себя всех прокариотов, научная классификация изменилась после открытия в 1990-х годах того, что прокариоты состоят из двух очень разных групп организмов, которые произошли от одного древнего общего предка. Эти эволюционные домены называются Бактерии и Археи . [ 127 ]
Предками современных бактерий были одноклеточные микроорганизмы, которые были первыми формами жизни , появившимися на Земле около 4 миллиардов лет назад. На протяжении примерно 3 миллиардов лет большинство организмов были микроскопическими, а доминирующими формами жизни были бактерии и археи. [ 65 ] [ 128 ] Хотя бактериальные окаменелости существуют, такие как строматолиты , отсутствие у них отличительной морфологии не позволяет использовать их для изучения истории эволюции бактерий или для определения времени происхождения определенного вида бактерий. Однако последовательности генов могут быть использованы для реконструкции бактериальной филогении , и эти исследования показывают, что бактерии сначала отделились от архейной/эукариотической линии. [ 129 ] Бактерии также участвовали во втором великом эволюционном расхождении – археях и эукариотах. Здесь эукариоты возникли в результате вступления древних бактерий в эндосимбиотические ассоциации с предками эукариотических клеток, которые сами, возможно, были родственны археям . [ 67 ] [ 66 ] Это включало поглощение протоэукариотическими клетками альфапротеобактериальных симбионтов с образованием либо митохондрий , либо гидрогеносом , которые до сих пор встречаются у всех известных эукариев. Позже некоторые эукариоты, уже содержащие митохондрии, также поглотили цианобактериоподобные организмы. Это привело к образованию хлоропластов у водорослей и растений. Есть также некоторые водоросли, возникшие в результате еще более поздних эндосимбиотических событий. Здесь эукариоты поглотили эукариотические водоросли, которые развились в пластиду «второго поколения». [ 130 ] [ 131 ] Это известно как вторичный эндосимбиоз .
-
Морская Thiomargarita namibiensis , крупнейшая известная бактерия.
-
Хлоропласты эндосимбиотическом глаукофитов происхождении имеют слой пептидогликана что свидетельствует об их цианобактерий от , . [ 132 ]
-
Бактерии могут быть полезными. Этот червь Помпеи , экстремофил, встречающийся только в гидротермальных источниках , имеет защитную оболочку из бактерий.
Самая крупная известная бактерия, морская Thiomargarita namibiensis , видна невооруженным глазом и иногда достигает 0,75 мм (750 мкм). [ 133 ] [ 134 ]
Морские археи
[ редактировать ]
Архея ( по-гречески древний [ 136 ] составляют домен и царство одноклеточных микроорганизмов ) . Эти микробы являются прокариотами , то есть в их клетках нет клеточного ядра или каких-либо других мембраносвязанных органелл .
Первоначально архей относили к бактериям , но эта классификация устарела. [ 137 ] Клетки архей обладают уникальными свойствами, отличающими их от двух других областей жизни: бактерий и эукариот . Археи далее делятся на несколько признанных типов . Классификация сложна, поскольку большинство из них не были изолированы в лаборатории и были обнаружены только путем анализа их нуклеиновых кислот в образцах из окружающей среды.
Археи и бактерии в целом схожи по размеру и форме, хотя некоторые археи имеют очень странную форму, например, плоские и квадратные клетки Haloquadratum walsbyi . [ 138 ] Несмотря на это морфологическое сходство с бактериями, археи обладают генами и несколькими метаболическими путями , которые более тесно связаны с таковыми у эукариот, в частности, ферментами, участвующими в транскрипции и трансляции . Другие аспекты биохимии архей уникальны, например, их зависимость от эфирных липидов в клеточных мембранах , таких как археолы . Археи используют больше источников энергии, чем эукариоты: они варьируются от органических соединений , таких как сахара, до аммиака , ионов металлов или даже газообразного водорода . Солеустойчивые археи ( Haloarchaea ) используют солнечный свет в качестве источника энергии, а другие виды архей фиксируют углерод ; однако, в отличие от растений и цианобактерий , ни один известный вид архей не обладает обоими свойствами. Археи размножаются бесполым путем бинарным делением , фрагментацией или почкованием ; в отличие от бактерий и эукариотов, ни один известный вид не образует спор .
Археи особенно многочисленны в океанах, а археи в планктоне могут быть одной из самых многочисленных групп организмов на планете. Археи являются основной частью жизни на Земле и могут играть роль как в углеродном, так и в азотном цикле .
-
Галобактерии , обнаруженные в воде, близкой к насыщенной солью, теперь признаны архей.
-
Плоские квадратные клетки архей Haloquadratum walsbyi.
-
Methanosarcina barkeri — морская архея, производящая метан.
-
Термофилы , такие как Pyrolobus fumarii , выживают при температуре выше 100 °C.
-
Рисунок другого морского термофила, Pyrococcus Furiosus.
Морские протисты
[ редактировать ]Протисты — это эукариоты, которых нельзя отнести к растениям, грибам или животным. Обычно они одноклеточные и микроскопические. Жизнь зародилась как одноклеточные прокариоты ( бактерии и археи ), а позже развилась в более сложные эукариоты . Эукариоты — это более развитые формы жизни, известные как растения, животные, грибы и протисты. Термин «протист» исторически вошёл в употребление как удобный термин для обозначения эукариот, которых нельзя строго отнести к растениям, животным или грибам. Они не являются частью современной кладистики, поскольку являются парафилетическими (не имеют общего предка). Простейших можно разделить на четыре группы в зависимости от того, какое у них питание: растительное, животное, грибное или [ 139 ] или их смесь. [ 140 ]
Протисты по способу добывания пищи
| |||||||
---|---|---|---|---|---|---|---|
Тип простейшего | Описание | Пример | Другие примеры | ||||
Растительный | Автотрофные протисты, которые производят себе пищу без необходимости потреблять другие организмы, обычно с помощью фотосинтеза. | ![]() |
Красные водоросли Cyanidium sp. | Зеленые водоросли , бурые водоросли , диатомовые водоросли и некоторые динофлагелляты . Растительноподобные протисты являются важными компонентами фитопланктона, обсуждаемыми ниже . | |||
Животный | Гетеротрофные протисты, добывающие пищу, потребляя другие организмы. | ![]() |
Радиолярий- протист, рисунок Геккеля | Фораминиферы , а также некоторые морские амебы , инфузории и жгутиконосцы . | |||
Грибоподобный | Сапротрофные протисты, получающие пищу из останков разложившихся и разложившихся организмов. | ![]() |
Сети морской слизи образуют лабиринтные сети трубок, по которым могут перемещаться амебы без ложноножек. | Морские лишайники | |||
Миксотропы | Различный
|
Миксотрофные и осмотрофные протисты, которые получают пищу за счет комбинации вышеперечисленных веществ. | ![]() |
Эвглена мутабельная , фотосинтезирующий жгутиконосец. | Многие морские миксотропы встречаются среди простейших, в том числе среди инфузорий, ризарий и динофлагеллят. [ 141 ] |

Протисты — это очень разнообразные организмы, которые в настоящее время разделены на 18 типов, но их нелегко классифицировать. [ 143 ] [ 144 ] Исследования показали, что большое разнообразие протистов существует в океанах, глубоких морских жерлах и речных отложениях, что позволяет предположить, что большое количество эукариотических микробных сообществ еще не обнаружено. [ 145 ] [ 146 ] протистов мало исследовали Миксотрофных , но недавние исследования в морской среде показали, что миксотрофные протесты составляют значительную часть биомассы протистов . [ 141 ]
- Одноклеточные и микроскопические протисты
-
Диатомовые водоросли — основная группа водорослей, производящая около 20% мирового производства кислорода. [ 147 ]
-
У диатомовых водорослей стеклянные клеточные стенки состоят из кремнезема и называются панцирями . [ 148 ]
-
Ископаемые панцири диатомей от 32 до 40 млн лет назад
-
Одноклеточная водоросль Gephyrocapsa Oceanica.
-
Две динофлагелляты
-
Зооксантеллы — это фотосинтезирующие водоросли, живущие внутри хозяев, таких как кораллы .
-
Эта инфузория переваривает цианобактерии . Цитостом . или рот находится внизу справа
В отличие от клеток прокариот клетки эукариот высокоорганизованы. Растения, животные и грибы обычно многоклеточные и обычно макроскопические . Большинство протистов одноклеточные и микроскопические. Но есть исключения. Некоторые одноклеточные морские простейшие макроскопичны. Некоторые морские слизевики имеют уникальный жизненный цикл, включающий переключение между одноклеточными, колониальными и многоклеточными формами. [ 149 ] Другие морские протисты не являются ни одноклеточными, ни микроскопическими, например морские водоросли .
- Макроскопические протисты (см. также одноклеточные макроводоросли → )
-
Одноклеточная гигантская амеба имеет до 1000 ядер и достигает длины 5 мм (0,20 дюйма).
-
Gromia sphaerica — крупная сферическая раковинная амеба , оставляющая грязевые следы. Его диаметр составляет до 3,8 см (1,5 дюйма). [ 150 ]
-
Spiculosiphon Oceana — одноклеточный фораминифер , внешним видом и образом жизни имитирующий губку , вырастает до 5 см в длину.
-
Ксенофиофор абиссальных , еще один одноклеточный фораминифер, обитает в зонах . У него гигантская раковина диаметром до 20 см (7,9 дюйма). [ 151 ]
-
Гигантская ламинария , бурая водоросль , не является настоящим растением, однако она многоклеточная и может вырастать до 50 метров в высоту.
Протистов описывают как таксономическую сумку, куда все, что не вписывается ни в одно из основных биологических царств . можно поместить [ 152 ] Некоторые современные авторы предпочитают исключать многоклеточные организмы из традиционного определения простейшего, ограничивая простейших одноклеточными организмами. [ 153 ] [ 154 ] Это более ограниченное определение исключает морские водоросли и слизевики . [ 155 ]
Морские микроживотные
[ редактировать ]Внешние видео | |
---|---|
![]() |
В молодом возрасте животные развиваются на микроскопических стадиях, которые могут включать споры , яйца и личинки . По крайней мере, одна микроскопическая группа животных, паразитические книдарии Myxozoa , во взрослой форме являются одноклеточными и включают морские виды. Другие взрослые морские микроживотные многоклеточные. Микроскопические взрослые членистоногие чаще встречаются внутри страны, в пресной воде, но есть и морские виды. К микроскопическим взрослым морским ракообразным относятся некоторые копеподы , ветвистоусые и тихоходки (водяные медведи). Некоторые морские нематоды и коловратки слишком малы, чтобы их можно было распознать невооруженным глазом, как и многие лорициферы , включая недавно обнаруженные анаэробные виды, которые проводят свою жизнь в бескислородной среде. [ 156 ] [ 157 ] Веслоногие раконогие вносят больший вклад во вторичную продуктивность и поглощение углерода Мирового океана, чем любая другая группа организмов. [ 158 ] [ 159 ] Хотя клещей обычно не считают морскими организмами, большинство видов семейства Halacaridae обитают в море. [ 160 ]
- Морские микроживотные
-
Более 10 000 морских видов относятся к веслоногим ракообразным , маленьким, часто микроскопическим ракообразным.
-
Фотография в темном поле гастротриха . , червеобразного животного, живущего между частицами отложений
-
Панцирные Pliciloricus enigmaticus длиной около 0,2 мм обитают в пространствах между морским гравием.
-
Рисунок тихоходки (водяного медведя) на песчинке
-
Коловушки , обычно длиной 0,1–0,5 мм, могут выглядеть как протисты, но имеют много клеток и принадлежат к отряду Animalia.
Fungi
[edit]

Over 1500 species of fungi are known from marine environments.[161] These are parasitic on marine algae or animals, or are saprobes feeding on dead organic matter from algae, corals, protozoan cysts, sea grasses, wood and other substrata.[162] Spores of many species have special appendages which facilitate attachment to the substratum.[163] Marine fungi can also be found in sea foam and around hydrothermal areas of the ocean.[164] A diverse range of unusual secondary metabolites is produced by marine fungi.[165]
Mycoplankton are saprotropic members of the plankton communities of marine and freshwater ecosystems.[166][167] They are composed of filamentous free-living fungi and yeasts associated with planktonic particles or phytoplankton.[168] Similar to bacterioplankton, these aquatic fungi play a significant role in heterotrophic mineralization and nutrient cycling.[169] Mycoplankton can be up to 20 mm in diameter and over 50 mm in length.[170]
A typical milliliter of seawater contains about 103 to 104 fungal cells.[171] This number is greater in coastal ecosystems and estuaries due to nutritional runoff from terrestrial communities. A higher diversity of mycoplankton is found around coasts and in surface waters down to 1000 metres, with a vertical profile that depends on how abundant phytoplankton is.[172][173] This profile changes between seasons due to changes in nutrient availability.[174] Marine fungi survive in a constant oxygen deficient environment, and therefore depend on oxygen diffusion by turbulence and oxygen generated by photosynthetic organisms.[175]
Marine fungi can be classified as:[175]
- Lower fungi - adapted to marine habitats (zoosporic fungi, including mastigomycetes: oomycetes and chytridiomycetes)
- Higher fungi - filamentous, modified to planktonic lifestyle (hyphomycetes, ascomycetes, basidiomycetes). Most mycoplankton species are higher fungi.[172]
Lichens are mutualistic associations between a fungus, usually an ascomycete, and an alga or a cyanobacterium. Several lichens are found in marine environments.[176] Many more occur in the splash zone, where they occupy different vertical zones depending on how tolerant they are to submersion.[177] Some lichens live a long time; one species has been dated at 8,600 years.[178] However their lifespan is difficult to measure because what defines the same lichen is not precise.[179] Lichens grow by vegetatively breaking off a piece, which may or may not be defined as the same lichen, and two lichens of different ages can merge, raising the issue of whether it is the same lichen.[179] The sea snail Littoraria irrorata damages plants of Spartina in the sea marshes where it lives, which enables spores of intertidal ascomycetous fungi to colonise the plant. The snail then eats the fungal growth in preference to the grass itself.[180]
According to fossil records, fungi date back to the late Proterozoic era 900-570 million years ago. Fossil marine lichens 600 million years old have been discovered in China.[181] It has been hypothesized that mycoplankton evolved from terrestrial fungi, likely in the Paleozoic era (390 million years ago).[182]
Origin of animals
[edit]
The earliest animals were marine invertebrates, that is, vertebrates came later. Animals are multicellular eukaryotes,[note 2] and are distinguished from plants, algae, and fungi by lacking cell walls.[183] Marine invertebrates are animals that inhabit a marine environment apart from the vertebrate members of the chordate phylum; invertebrates lack a vertebral column. Some have evolved a shell or a hard exoskeleton.
The earliest animal fossils may belong to the genus Dickinsonia,[184] 571 million to 541 million years ago.[185] Individual Dickinsonia typically resemble a bilaterally symmetrical ribbed oval. They kept growing until they were covered with sediment or otherwise killed,[186] and spent most of their lives with their bodies firmly anchored to the sediment.[187] Their taxonomic affinities are presently unknown, but their mode of growth is consistent with a bilaterian affinity.[188]
Apart from Dickinsonia, the earliest widely accepted animal fossils are the rather modern-looking cnidarians (the group that includes coral, jellyfish, sea anemones and Hydra), possibly from around 580 Ma[189] The Ediacara biota, which flourished for the last 40 million years before the start of the Cambrian,[190] were the first animals more than a very few centimetres long. Like Dickinsonia, many were flat with a "quilted" appearance, and seemed so strange that there was a proposal to classify them as a separate kingdom, Vendozoa.[191] Others, however, have been interpreted as early molluscs (Kimberella[192][193]), echinoderms (Arkarua[194]), and arthropods (Spriggina,[195] Parvancorina[196]). There is still debate about the classification of these specimens, mainly because the diagnostic features which allow taxonomists to classify more recent organisms, such as similarities to living organisms, are generally absent in the Ediacarans. However, there seems little doubt that Kimberella was at least a triploblastic bilaterian animal, in other words, an animal significantly more complex than the cnidarians.[197]
Small shelly fauna are a very mixed collection of fossils found between the Late Ediacaran and Middle Cambrian periods. The earliest, Cloudina, shows signs of successful defense against predation and may indicate the start of an evolutionary arms race. Some tiny Early Cambrian shells almost certainly belonged to molluscs, while the owners of some "armor plates," Halkieria and Microdictyon, were eventually identified when more complete specimens were found in Cambrian lagerstätten that preserved soft-bodied animals.[198]
Body plans and phyla
[edit]
Invertebrates are grouped into different phyla. Informally phyla can be thought of as a way of grouping organisms according to their body plan.[199][200]: 33 A body plan refers to a blueprint which describes the shape or morphology of an organism, such as its symmetry, segmentation and the disposition of its appendages. The idea of body plans originated with vertebrates, which were grouped into one phylum. But the vertebrate body plan is only one of many, and invertebrates consist of many phyla or body plans. The history of the discovery of body plans can be seen as a movement from a worldview centred on vertebrates, to seeing the vertebrates as one body plan among many. Among the pioneering zoologists, Linnaeus identified two body plans outside the vertebrates; Cuvier identified three; and Haeckel had four, as well as the Protista with eight more, for a total of twelve. For comparison, the number of phyla recognised by modern zoologists has risen to 35.[200]


Historically body plans were thought of as having evolved rapidly during the Cambrian explosion,[204] but a more nuanced understanding of animal evolution suggests a gradual development of body plans throughout the early Palaeozoic and beyond.[205] More generally a phylum can be defined in two ways: as described above, as a group of organisms with a certain degree of morphological or developmental similarity (the phenetic definition), or a group of organisms with a certain degree of evolutionary relatedness (the phylogenetic definition).[205]
In the 1970s there was already a debate about whether the emergence of the modern phyla was "explosive" or gradual but hidden by the shortage of Precambrian animal fossils.[198] A re-analysis of fossils from the Burgess Shale lagerstätte increased interest in the issue when it revealed animals, such as Opabinia, which did not fit into any known phylum. At the time these were interpreted as evidence that the modern phyla had evolved very rapidly in the Cambrian explosion and that the Burgess Shale's "weird wonders" showed that the Early Cambrian was a uniquely experimental period of animal evolution.[206] Later discoveries of similar animals and the development of new theoretical approaches led to the conclusion that many of the "weird wonders" were evolutionary "aunts" or "cousins" of modern groups[207]—for example that Opabinia was a member of the lobopods, a group which includes the ancestors of the arthropods, and that it may have been closely related to the modern tardigrades.[208] Nevertheless, there is still much debate about whether the Cambrian explosion was really explosive and, if so, how and why it happened and why it appears unique in the history of animals.[209]
Earliest animals
[edit]The deepest-branching animals — the earliest animals that appeared during evolution — are marine non-vertebrate organisms. The earliest animal phyla are the Porifera, Ctenophora, Placozoa and Cnidaria. No member of these clades exhibit body plans with bilateral symmetry.
| ||||||||||||||||||||||||||||||||||
Marine sponges
[edit]
Sponges are animals of the phylum Porifera (from Modern Latin for bearing pores[213]). They are multicellular organisms that have bodies full of pores and channels allowing water to circulate through them, consisting of jelly-like mesohyl sandwiched between two thin layers of cells. They have unspecialized cells that can transform into other types and that often migrate between the main cell layers and the mesohyl in the process. Sponges do not have nervous, digestive or circulatory systems. Instead, most rely on maintaining a constant water flow through their bodies to obtain food and oxygen and to remove wastes.
Sponges are similar to other animals in that they are multicellular, heterotrophic, lack cell walls and produce sperm cells. Unlike other animals, they lack true tissues and organs, and have no body symmetry. The shapes of their bodies are adapted for maximal efficiency of water flow through the central cavity, where it deposits nutrients, and leaves through a hole called the osculum. Many sponges have internal skeletons of spongin and/or spicules of calcium carbonate or silicon dioxide. All sponges are sessile aquatic animals. Although there are freshwater species, the great majority are marine (salt water) species, ranging from tidal zones to depths exceeding 8,800 m (5.5 mi). Some sponges live to great ages; there is evidence of the deep-sea glass sponge Monorhaphis chuni living about 11,000 years.[214][215]
While most of the approximately 5,000–10,000 known species feed on bacteria and other food particles in the water, some host photosynthesizing micro-organisms as endosymbionts and these alliances often produce more food and oxygen than they consume. A few species of sponge that live in food-poor environments have become carnivores that prey mainly on small crustaceans.[216]
-
Sponge biodiversity. There are four sponge species in this photo.
-
Venus' flower basket at a depth of 2572 meters
-
The long-living Monorhaphis chuni
Linnaeus mistakenly identified sponges as plants in the order Algae.[217] For a long time thereafter sponges were assigned to a separate subkingdom, Parazoa (meaning beside the animals).[218] They are now classified as a paraphyletic phylum from which the higher animals have evolved.[219]
Ctenophores
[edit]Ctenophores (from Greek for carrying a comb), commonly known as comb jellies, are a phylum that live worldwide in marine waters. They are the largest non-colonial animals to swim with the help of cilia (hairs or combs).[220] Coastal species need to be tough enough to withstand waves and swirling sediment, but some oceanic species are so fragile and transparent that it is very difficult to capture them intact for study.[221] In the past ctenophores were thought to have only a modest presence in the ocean, but it is now known they are often significant and even dominant parts of the planktonic biomass.[222]: 269
The phylum has about 150 known species with a wide range of body forms. Sizes range from a few millimeters to 1.5 m (4 ft 11 in). Cydippids are egg-shaped with their cilia arranged in eight radial comb rows, and deploy retractable tentacles for capturing prey. The benthic platyctenids are generally combless and flat. The coastal beroids have gaping mouths and lack tentacles. Most adult ctenophores prey on microscopic larvae and rotifers and small crustaceans but beroids prey on other ctenophores.
-
Light diffracting along the comb rows of a cydippid, left tentacle deployed, right retracted
-
Deep-sea ctenophore trailing tentacles studded with tentilla (sub-tentacles)
-
Egg-shaped cydippid ctenophore
-
Group of small benthic creeping comb jellies streaming tentacles and living symbiotically on a starfish.
-
Lobata sp. with paired thick lobes
-
The sea walnut has a transient anus which forms only when it needs to defecate.[223]
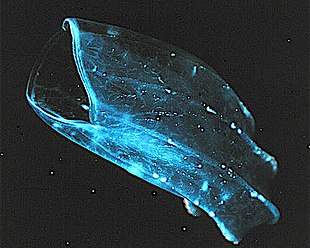
Early writers combined ctenophores with cnidarians. Ctenophores resemble cnidarians in relying on water flow through the body cavity for both digestion and respiration, as well as in having a decentralized nerve net rather than a brain. Also like cnidarians, the bodies of ctenophores consist of a mass of jelly, with one layer of cells on the outside and another lining the internal cavity. In ctenophores, however, these layers are two cells deep, while those in cnidarians are only a single cell deep. While cnidarians exhibit radial symmetry, ctenophores have two anal canals which exhibit biradial symmetry (half-turn rotational symmetry).[224] The position of the ctenophores in the evolutionary family tree of animals has long been debated, and the majority view at present, based on molecular phylogenetics, is that cnidarians and bilaterians are more closely related to each other than either is to ctenophores.[222]: 222
External videos | |
---|---|
![]() |
Placozoa
[edit]Placozoa (from Greek for flat animals) have the simplest structure of all animals. They are a basal form of free-living (non-parasitic) multicellular organism[225] that do not yet have a common name.[226] They live in marine environments and form a phylum containing so far only three described species, of which the first, the classical Trichoplax adhaerens, was discovered in 1883.[227] Two more species have been discovered since 2017,[228][229] and genetic methods indicate this phylum has a further 100 to 200 undescribed species.[230]

Trichoplax is a small, flattened, animal about one mm across and usually about 25 μm thick. Like the amoebae they superficially resemble, they continually change their external shape. In addition, spherical phases occasionally form which may facilitate movement. Trichoplax lacks tissues and organs. There is no manifest body symmetry, so it is not possible to distinguish anterior from posterior or left from right. It is made up of a few thousand cells of six types in three distinct layers.[231] The outer layer of simple epithelial cells bear cilia which the animal uses to help it creep along the seafloor.[232] Trichoplax feed by engulfing and absorbing food particles – mainly microbes and organic detritus – with their underside.
Marine cnidarians
[edit]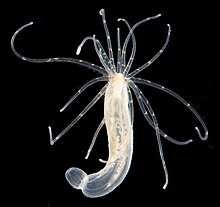
Cnidarians (from Greek for nettle) are distinguished by the presence of stinging cells, specialized cells that they use mainly for capturing prey. Cnidarians include corals, sea anemones, jellyfish and hydrozoans. They form a phylum containing over 10,000[233] species of animals found exclusively in aquatic (mainly marine) environments. Their bodies consist of mesoglea, a non-living jelly-like substance, sandwiched between two layers of epithelium that are mostly one cell thick. They have two basic body forms: swimming medusae and sessile polyps, both of which are radially symmetrical with mouths surrounded by tentacles that bear cnidocytes. Both forms have a single orifice and body cavity that are used for digestion and respiration.
Fossil cnidarians have been found in rocks formed about 580 million years ago. Fossils of cnidarians that do not build mineralized structures are rare. Scientists currently think cnidarians, ctenophores and bilaterians are more closely related to calcareous sponges than these are to other sponges, and that anthozoans are the evolutionary "aunts" or "sisters" of other cnidarians, and the most closely related to bilaterians.
Cnidarians are the simplest animals in which the cells are organised into tissues.[234] The starlet sea anemone is used as a model organism in research.[235] It is easy to care for in the laboratory and a protocol has been developed which can yield large numbers of embryos on a daily basis.[236] There is a remarkable degree of similarity in the gene sequence conservation and complexity between the sea anemone and vertebrates.[236] In particular, genes concerned in the formation of the head in vertebrates are also present in the anemone.[237][238]
-
Sea anemones are common in tidepools.
-
Their tentacles sting and paralyse small fish.
-
If an island sinks below the sea, coral growth can keep up with rising water and form an atoll.
-
The mantle of the red paper lantern jellyfish crumples and expands like a paper lantern.[239]
-
Marrus orthocanna another colonial siphonophore, assembled from two types of zooids.
-
Turritopsis dohrnii achieves biological immortality by transferring its cells back to childhood.[242][243]
Bilateral invertebrate animals
[edit]
Some of the earliest bilaterians were wormlike, and the original bilaterian may have been a bottom dwelling worm with a single body opening.[245] A bilaterian body can be conceptualized as a cylinder with a gut running between two openings, the mouth and the anus. Around the gut it has an internal body cavity, a coelom or pseudocoelom.[a] Animals with this bilaterally symmetric body plan have a head (anterior) end and a tail (posterior) end as well as a back (dorsal) and a belly (ventral); therefore they also have a left side and a right side.[246][247]
Having a front end means that this part of the body encounters stimuli, such as food, favouring cephalisation, the development of a head with sense organs and a mouth.[248] The body stretches back from the head, and many bilaterians have a combination of circular muscles that constrict the body, making it longer, and an opposing set of longitudinal muscles, that shorten the body;[247] these enable soft-bodied animals with a hydrostatic skeleton to move by peristalsis.[249] They also have a gut that extends through the basically cylindrical body from mouth to anus. Many bilaterian phyla have primary larvae which swim with cilia and have an apical organ containing sensory cells. However, there are exceptions to each of these characteristics; for example, adult echinoderms are radially symmetric (unlike their larvae), and certain parasitic worms have extremely simplified body structures.[246][247]
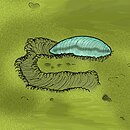
← bilaterians |
| |||||||||||||||||||||
Protostomes
[edit]Protostomes (from Greek for first mouth) are a superphylum of animals. It is a sister clade of the deuterostomes (from Greek for second mouth), with which it forms the Nephrozoa clade. Protostomes are distinguished from deuterostomes by the way their embryos develop. In protostomes the first opening that develops becomes the mouth, while in deuterostomes it becomes the anus.[251][252]
← Protostomes |
| ||||||||||||||||||
(extant) |
Marine worms
[edit]
Worms (Old English for serpents) form a number of phyla. Different groups of marine worms are related only distantly, so they are found in several different phyla such as the Annelida (segmented worms), Chaetognatha (arrow worms), Phoronida (horseshoe worms), and Hemichordata. All worms, apart from the Hemichordata, are protostomes. The Hemichordata are deuterostomes and are discussed in their own section below.
The typical body plan of a worm involves long cylindrical tube-like bodies and no limbs. Marine worms vary in size from microscopic to over 1 metre (3.3 ft) in length for some marine polychaete worms (bristle worms)[253] and up to 58 metres (190 ft) for the marine nemertean worm (bootlace worm).[254] Some marine worms occupy a small variety of parasitic niches, living inside the bodies of other animals, while others live more freely in the marine environment or by burrowing underground. Many of these worms have specialized tentacles used for exchanging oxygen and carbon dioxide and also may be used for reproduction. Some marine worms are tube worms, such as the giant tube worm which lives in waters near underwater volcanoes and can withstand temperatures up to 90 degrees Celsius. Platyhelminthes (flatworms) form another worm phylum which includes a class of parasitic tapeworms. The marine tapeworm Polygonoporus giganticus, found in the gut of sperm whales, can grow to over 30 m (100 ft).[255][256]
Nematodes (roundworms) constitute a further worm phylum with tubular digestive systems and an opening at both ends.[257][258] Over 25,000 nematode species have been described,[259][260] of which more than half are parasitic. It has been estimated another million remain undescribed.[261] They are ubiquitous in marine, freshwater and terrestrial environments, where they often outnumber other animals in both individual and species counts. They are found in every part of the Earth's lithosphere, from the top of mountains to the bottom of oceanic trenches.[262] By count they represent 90% of all animals on the ocean floor.[263] Their numerical dominance, often exceeding a million individuals per square meter and accounting for about 80% of all individual animals on Earth, their diversity of life cycles, and their presence at various trophic levels point at an important role in many ecosystems.[264]
-
Giant tube worms cluster around hydrothermal vents.
-
Nematodes are ubiquitous pseudocoelomates which can parasite marine plants and animals.
-
Bloodworms are typically found on the bottom of shallow marine waters.
Marine molluscs
[edit]Molluscs (Latin for soft) form a phylum with about 85,000 extant recognized species.[267] They are the largest marine phylum in terms of species count, containing about 23% of all the named marine organisms.[268] Molluscs have more varied forms than other invertebrate phyla. They are highly diverse, not just in size and in anatomical structure, but also in behaviour and in habitat.

The mollusc phylum is divided into 9 or 10 taxonomic classes. These classes include gastropods, bivalves and cephalopods, as well as other lesser-known but distinctive classes. Gastropods with protective shells are referred to as snails, whereas gastropods without protective shells are referred to as slugs. Gastropods are by far the most numerous molluscs in terms of species.[269] Bivalves include clams, oysters, cockles, mussels, scallops, and numerous other families. There are about 8,000 marine bivalves species (including brackish water and estuarine species). A deep sea ocean quahog clam has been reported as having lived 507 years[270] making it the longest recorded life of all animals apart from colonial animals, or near-colonial animals like sponges.[214]
- Gastropods and bivalves
-
The sea snail Syrinx aruanus has a shell up to 91 cm long, the largest of any living gastropod.
-
Molluscs usually have eyes. Bordering the edge of the mantle of a scallop, a bivalve mollusc, can be over 100 simple eyes.
-
Common mussel, another bivalve
Cephalopods include octopus, squid and cuttlefish. About 800 living species of marine cephalopods have been identified,[271] and an estimated 11,000 extinct taxa have been described.[272] They are found in all oceans, but there are no fully freshwater cephalopods.[273]
- Cephalopods
-
The nautilus is a living fossil little changed since it evolved 500 million years ago as one of the first cephalopods.[274][275][276]
-
Colossal squid, the largest of all invertebrates[277]
Molluscs have such diverse shapes that many textbooks base their descriptions of molluscan anatomy on a generalized or hypothetical ancestral mollusc. This generalized mollusc is unsegmented and bilaterally symmetrical with an underside consisting of a single muscular foot. Beyond that it has three further key features. Firstly, it has a muscular cloak called a mantle covering its viscera and containing a significant cavity used for breathing and excretion. A shell secreted by the mantle covers the upper surface. Secondly (apart from bivalves) it has a rasping tongue called a radula used for feeding. Thirdly, it has a nervous system including a complex digestive system using microscopic, muscle-powered hairs called cilia to exude mucus. The generalized mollusc has two paired nerve cords (three in bivalves). The brain, in species that have one, encircles the esophagus. Most molluscs have eyes and all have sensors detecting chemicals, vibrations, and touch.[278][279]
Good evidence exists for the appearance of marine gastropods, cephalopods and bivalves in the Cambrian period 538.8 to 485.4 million years ago.
Marine arthropods
[edit]Arthropods (Greek for jointed feet) have an exoskeleton (external skeleton), a segmented body, and jointed appendages (paired appendages). They form a phylum which includes insects, arachnids, myriapods, and crustaceans. Arthropods are characterized by their jointed limbs and cuticle made of chitin, often mineralised with calcium carbonate. The arthropod body plan consists of segments, each with a pair of appendages. The rigid cuticle inhibits growth, so arthropods replace it periodically by moulting. Their versatility has enabled them to become the most species-rich members of all ecological guilds in most environments.
The evolutionary ancestry of arthropods dates back to the Cambrian period and is generally regarded as monophyletic. However, basal relationships of arthropods with extinct phyla such as lobopodians have recently been debated.[282][283]
Panarthropoda |
| ||||||||||||
- Arthropod fossils and living fossils
-
Fossil trilobite. Trilobites first appeared about 521 Ma. They were highly successful and were found everywhere in the ocean for 270 Ma.[285]
-
The Anomalocaris ("abnormal shrimp") was one of the first apex predators and first appeared about 515 Ma.
-
The largest known arthropod, the sea scorpion Jaekelopterus rhenaniae, has been found in estuarine strata from about 390 Ma. It was up to 2.5 m (8.2 ft) long.[286][287]
Extant marine arthropods range in size from the microscopic crustacean Stygotantulus to the Japanese spider crab. Arthropods' primary internal cavity is a hemocoel, which accommodates their internal organs, and through which their haemolymph - analogue of blood - circulates; they have open circulatory systems. Like their exteriors, the internal organs of arthropods are generally built of repeated segments. Their nervous system is "ladder-like", with paired ventral nerve cords running through all segments and forming paired ganglia in each segment. Their heads are formed by fusion of varying numbers of segments, and their brains are formed by fusion of the ganglia of these segments and encircle the esophagus. The respiratory and excretory systems of arthropods vary, depending as much on their environment as on the subphylum to which they belong.
- Modern crustaceans
-
Many crustaceans are very small, like this tiny amphipod, and make up a significant part of the ocean's zooplankton.
-
The Japanese spider crab has the longest leg span of any arthropod, reaching 5.5 metres (18 ft) from claw to claw.[289]
-
The Tasmanian giant crab is long-lived and slow-growing, making it vulnerable to overfishing.[290]
-
Mantis shrimp have the most advanced eyes in the animal kingdom,[291] and smash prey by swinging their club-like raptorial claws.[292]
Arthropod vision relies on various combinations of compound eyes and pigment-pit ocelli: in most species the ocelli can only detect the direction from which light is coming, and the compound eyes are the main source of information. Arthropods also have a wide range of chemical and mechanical sensors, mostly based on modifications of the many setae (bristles) that project through their cuticles. Arthropod methods of reproduction are diverse: terrestrial species use some form of internal fertilization while marine species lay eggs using either internal or external fertilization. Arthropod hatchlings vary from miniature adults to grubs that lack jointed limbs and eventually undergo a total metamorphosis to produce the adult form.
Deuterostomes
[edit]In deuterostomes the first opening that develops in the growing embryo becomes the anus, while in protostomes it becomes the mouth. Deuterostomes form a superphylum of animals and are the sister clade of the protostomes.[251][252] It is once considered that the earliest known deuterostomes are Saccorhytus fossils from about 540 million years ago.[293] However, another study considered that Saccorhytus is more likely to be an ecdysozoan.[294]
← deuterostomes | |
(extant) |
Echinoderms
[edit]
Echinoderms (Greek for spiny skin) is a phylum which contains only marine invertebrates. The phylum contains about 7000 living species,[295] making it the second-largest grouping of deuterostomes, after the chordates.
Adult echinoderms are recognizable by their radial symmetry (usually five-point) and include starfish, sea urchins, sand dollars, and sea cucumbers, as well as the sea lilies.[296] Echinoderms are found at every ocean depth, from the intertidal zone to the abyssal zone. They are unique among animals in having bilateral symmetry at the larval stage, but fivefold symmetry (pentamerism, a special type of radial symmetry) as adults.[297]
Echinoderms are important both biologically and geologically. Biologically, there are few other groupings so abundant in the biotic desert of the deep sea, as well as shallower oceans. Most echinoderms are able to regenerate tissue, organs, limbs, and reproduce asexually; in some cases, they can undergo complete regeneration from a single limb. Geologically, the value of echinoderms is in their ossified skeletons, which are major contributors to many limestone formations, and can provide valuable clues as to the geological environment. They were the most used species in regenerative research in the 19th and 20th centuries.
-
Echinoderm literally means "spiny skin", as this water melon sea urchin illustrates.
-
The ochre sea star was the first keystone predator to be studied. They limit mussels which can overwhelm intertidal communities.[298]
-
Colorful sea lilies in shallow waters
-
Sea cucumbers filter feed on plankton and suspended solids.
-
The sea pig, a deep water sea cucumber, is the only echinoderm that uses legged locomotion.
-
A benthopelagic and bioluminescent swimming sea cucumber, 3200 metres deep
It is held by some scientists that the radiation of echinoderms was responsible for the Mesozoic Marine Revolution. Aside from the hard-to-classify Arkarua (a Precambrian animal with echinoderm-like pentamerous radial symmetry), the first definitive members of the phylum appeared near the start of the Cambrian.
Hemichordates
[edit]Hemichordates form a sister phylum to the echinoderms. They are solitary worm-shaped organisms rarely seen by humans because of their lifestyle. They include two main groups, the acorn worms and the Pterobranchia. Pterobranchia form a class containing about 30 species of small worm-shaped animals that live in secreted tubes on the ocean floor. Acorn worms form a class containing about 111 species that generally live in U-shaped burrows on the seabed, from the shoreline to a depth of 3000 metres. The worms lie there with the proboscis sticking out of one opening in the burrow, subsisting as deposit feeders or suspension feeders. It is supposed the ancestors of acorn worms used to live in tubes like their relatives, the Pterobranchia, but eventually started to live a safer and more sheltered existence in sediment burrows.[302] Some of these worms may grow to be very long; one particular species may reach a length of 2.5 metres (8 ft 2 in), although most acorn worms are much smaller.
Acorn worms are more highly specialised and advanced than other worm-like organisms. They have a circulatory system with a heart that also functions as a kidney. Acorn worms have gill-like structures they use for breathing, similar to the gills of fish. Therefore, acorn worms are sometimes said to be a link between classical invertebrates and vertebrates. Acorn worms continually form new gill slits as they grow in size, and some older individuals have more than a hundred on each side. Each slit consists of a branchial chamber opening to the pharynx through a U-shaped cleft. Cilia push water through the slits, maintaining a constant flow, just as in fish.[303] Some acorn worms also have a postanal tail which may be homologous to the post-anal tail of vertebrates.
The three-section body plan of the acorn worm is no longer present in the vertebrates, except in the anatomy of the frontal neural tube, later developed into a brain divided into three parts. This means some of the original anatomy of the early chordate ancestors is still present in vertebrates even if it is not always visible. One theory is the three-part body originated from an early common ancestor of the deuterostomes, and maybe even from a common bilateral ancestor of both deuterostomes and protostomes. Studies have shown the gene expression in the embryo share three of the same signaling centers that shape the brains of all vertebrates, but instead of taking part in the formation of their neural system,[304] they are controlling the development of the different body regions.[305]
Marine chordates
[edit]
The chordate phylum has three subphyla, one of which is the vertebrates (see below). The other two subphyla are marine invertebrates: the tunicates (salps and sea squirts) and the cephalochordates (such as lancelets). Invertebrate chordates are close relatives to vertebrates. In particular, there has been discussion about how closely some extinct marine species, such as Pikaiidae, Palaeospondylus, Zhongxiniscus and Vetulicolia, might relate ancestrally to vertebrates.
- Invertebrate chordates are close relatives of vertebrates
-
The lancelet, a small translucent fish-like cephalochordate, is one of the closest living invertebrate relative of the vertebrates.[307][308]
-
Tunicates, like these fluorescent-colored sea squirts, may provide clues to vertebrate and therefore human ancestry.[309]
-
Pyrosomes are free-floating bioluminescent tunicates made up of hundreds of individuals.
-
Salp chain
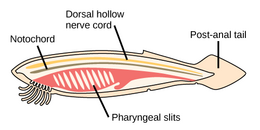
Vertebrate animals
[edit]Vertebrates (Latin for joints of the spine) are a subphylum of chordates. They are chordates that have a vertebral column (backbone). The vertebral column provides the central support structure for an internal skeleton which gives shape, support, and protection to the body and can provide a means of anchoring fins or limbs to the body. The vertebral column also serves to house and protect the spinal cord that lies within the vertebral column.
Marine vertebrates can be divided into marine fish and marine tetrapods.
Marine fish
[edit]Fish typically breathe by extracting oxygen from water through gills and have a skin protected by scales and mucous. They use fins to propel and stabilise themselves in the water, and usually have a two-chambered heart and eyes well adapted to seeing underwater, as well as other sensory systems. Over 33,000 species of fish have been described as of 2017,[310] of which about 20,000 are marine fish.[311]
← vertebrates | |
(extant) |
Jawless fish
[edit]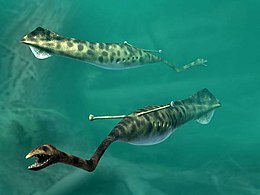
Early fish had no jaws. Most went extinct when they were outcompeted by jawed fish (below), but two groups survived: hagfish and lampreys. Hagfish form a class of about 20 species of eel-shaped, slime-producing marine fish. They are the only known living animals that have a skull but no vertebral column. Lampreys form a superclass containing 38 known extant species of jawless fish.[312] The adult lamprey is characterized by a toothed, funnel-like sucking mouth. Although they are well known for boring into the flesh of other fish to suck their blood,[313] only 18 species of lampreys are actually parasitic.[314] Together hagfish and lampreys are the sister group to vertebrates. Living hagfish remain similar to hagfish from around 300 million years ago.[315] The lampreys are a very ancient lineage of vertebrates, though their exact relationship to hagfishes and jawed vertebrates is still a matter of dispute.[316] Molecular analysis since 1992 has suggested that hagfish are most closely related to lampreys,[317] and so also are vertebrates in a monophyletic sense. Others consider them a sister group of vertebrates in the common taxon of craniata.[318]
The Tully monster is an extinct genus of soft-bodied bilaterians that lived in tropical estuaries about 300 million years ago. Since 2016 there has been controversy over whether this animal was a vertebrate or an invertebrate.[319][320] In 2020 researchers found "strong evidence" that the Tully monster was a vertebrate, and was a jawless fish in the lineage of the lamprey,[321][322] while in 2023 other researchers found 3D fossils scans did not support those conclusions.[323]
-
Lampreys are often parasitic and have a toothed, funnel-like sucking mouth.
-
The extinct Pteraspidomorphi, ancestral to jawed vertebrates
Pteraspidomorphi is an extinct class of early jawless fish ancestral to jawed vertebrates. The few characteristics they share with the latter are now considered as primitive for all vertebrates.
Around the start of the Devonian, fish started appearing with a deep remodelling of the vertebrate skull that resulted in a jaw.[324] All vertebrate jaws, including the human jaw, have evolved from these early fish jaws. The appearance of the early vertebrate jaw has been described as "perhaps the most profound and radical evolutionary step in vertebrate history".[325][326] Jaws make it possible to capture, hold, and chew prey. Fish without jaws had more difficulty surviving than fish with jaws, and most jawless fish became extinct during the Triassic period.
Cartilaginous fish
[edit]Jawed fish fall into two main groups: fish with bony internal skeletons and fish with cartilaginous internal skeletons. Cartilaginous fish, such as sharks and rays, have jaws and skeletons made of cartilage rather than bone. Megalodon is an extinct species of shark that lived about 28 to 1.5 Ma. It may looked much like a stocky version of the great white shark, but was much larger with estimated lengths reaching 20.3 metres (67 ft).[327] Found in all oceans[328] it was one of the largest and most powerful predators in vertebrate history,[327] and probably had a profound impact on marine life.[329] The Greenland shark has the longest known lifespan of all vertebrates, about 400 years.[330] Some sharks such as the great white are partially warm blooded and give live birth. The manta ray, largest ray in the world, has been targeted by fisheries and is now vulnerable.[331]
- Cartilaginous fishes
-
Cartilaginous fishes may have evolved from spiny sharks.
-
Manta ray, the largest ray
-
The extinct megalodon resembled a giant great white shark.
-
The Greenland shark lives longer than any other vertebrate.
Bony fish
[edit]


Bony fish have jaws and skeletons made of bone rather than cartilage. Bony fish also have hard, bony plates called operculum which help them respire and protect their gills, and they often possess a swim bladder which they use for better control of their buoyancy. Bony fish can be further divided into those with lobe fins and those with ray fins. The approximate dates in the phylogenetic tree are from Near et al., 2012[333] and Zhu et al., 2009.[334]
← bony fish |
| ||||||||||||||||||
(extant) |
Lobe fins have the form of fleshy lobes supported by bony stalks which extend from the body.[335] Guiyu oneiros, the earliest-known bony fish, lived during the Late Silurian 419 million years ago. It has the combination of both ray-finned and lobe-finned features, although analysis of the totality of its features place it closer to lobe-finned fish.[334] Lobe fins evolved into the legs of the first tetrapod land vertebrates, so by extension an early ancestor of humans was a lobe-finned fish. Apart from the coelacanths and the lungfishes, lobe-finned fishes are now extinct.
The remaining bony fish have ray fins. These are made of webs of skin supported by bony or horny spines (rays) which can be erected to control the fin stiffness.
- The main distinguishing feature of the chondrosteans (sturgeon, paddlefish, bichir and reedfish) is the cartilaginous nature of their skeletons. The ancestors of the chondrosteans are thought to be bony fish, but the characteristic of an ossified skeleton was lost in later evolutionary development, resulting in a lightening of the frame.[336]
- Neopterygians (from Greek for new fins) appeared sometime in the Late Permian, before dinosaurs. They were a very successful group of fish, because they could move more rapidly than their ancestors. Their scales and skeletons began to lighten during their evolution, and their jaws became more powerful and efficient.[337]
Teleosts
[edit]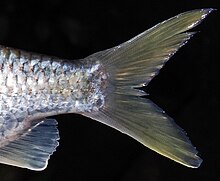
About 96% of all modern fish species are teleosts,[338] of which about 14,000 are marine species.[339] Teleosts can be distinguished from other bony fish by their possession of a homocercal tail, a tail where the upper half mirrors the lower half.[340] Another difference lies in their jaw bones – teleosts have modifications in the jaw musculature which make it possible for them to protrude their jaws. This enables them to grab prey and draw it into their mouth.[340] In general, teleosts tend to be quicker and more flexible than more basal bony fishes. Their skeletal structure has evolved towards greater lightness. While teleost bones are well calcified, they are constructed from a scaffolding of struts, rather than the dense cancellous bones of holostean fish.[341]
Teleosts are found in almost all marine habitats.[342] They have enormous diversity, and range in size from adult gobies 8mm long [343] to ocean sunfish weighing over 2,000 kg.[344] The following images show something of the diversity in the shape and colour of modern marine teleosts...
Nearly half of all extant vertebrate species are teleosts.[345]
Marine tetrapods
[edit]
A tetrapod (Greek for four feet) is a vertebrate with limbs (feet). Tetrapods evolved from ancient lobe-finned fishes about 400 million years ago during the Devonian Period when their earliest ancestors emerged from the sea and adapted to living on land.[346] This change from a body plan for breathing and navigating in gravity-neutral water to a body plan with mechanisms enabling the animal to breath in air without dehydrating and move on land is one of the most profound evolutionary changes known.[347][348] Tetrapods can be divided into four classes: amphibians, reptiles, birds and mammals.
← tetrapods |
| ||||||||||||||||||
Marine tetrapods are tetrapods that returned from land back to the sea again. The first returns to the ocean may have occurred as early as the Carboniferous Period[349] whereas other returns occurred as recently as the Cenozoic, as in cetaceans, pinnipeds,[350] and several modern amphibians.[351] Amphibians (from Greek for both kinds of life) live part of their life in water and part on land. They mostly require fresh water to reproduce. A few inhabit brackish water, but there are no true marine amphibians.[352] There have been reports, however, of amphibians invading marine waters, such as a Black Sea invasion by the natural hybrid Pelophylax esculentus reported in 2010.[353]
Reptiles
[edit]Reptiles (Late Latin for creeping or crawling) do not have an aquatic larval stage, and in this way are unlike amphibians. Most reptiles are oviparous, although several species of squamates are viviparous, as were some extinct aquatic clades[354] — the fetus develops within the mother, contained in a placenta rather than an eggshell. As amniotes, reptile eggs are surrounded by membranes for protection and transport, which adapt them to reproduction on dry land. Many of the viviparous species feed their fetuses through various forms of placenta analogous to those of mammals, with some providing initial care for their hatchlings.
Some reptiles are more closely related to birds than other reptiles, and many scientists prefer to make Reptilia a monophyletic group which includes the birds.[355][356][357][358] Extant non-avian reptiles which inhabit or frequent the sea include sea turtles, sea snakes, terrapins, the marine iguana, and the saltwater crocodile. Currently, of the approximately 12,000 extant reptile species and sub-species, only about 100 of are classed as marine reptiles.[359]
Except for some sea snakes, most extant marine reptiles are oviparous and need to return to land to lay their eggs. Apart from sea turtles, the species usually spend most of their lives on or near land rather than in the ocean. Sea snakes generally prefer shallow waters nearby land, around islands, especially waters that are somewhat sheltered, as well as near estuaries.[360][361] Unlike land snakes, sea snakes have evolved flattened tails which help them swim.[362]
-
Marine snakes have flattened tails.
-
The ancient Ichthyosaurus communis independently evolved flippers similar to dolphins.
Some extinct marine reptiles, such as ichthyosaurs, evolved to be viviparous and had no requirement to return to land. Ichthyosaurs resembled dolphins. They first appeared about 245 million years ago and disappeared about 90 million years ago. The terrestrial ancestor of the ichthyosaur had no features already on its back or tail that might have helped along the evolutionary process. Yet the ichthyosaur developed a dorsal and tail fin which improved its ability to swim.[363] The biologist Stephen Jay Gould said the ichthyosaur was his favourite example of convergent evolution.[364] The earliest marine reptiles arose in the Permian. During the Mesozoic many groups of reptiles became adapted to life in the seas, including ichthyosaurs, plesiosaurs, mosasaurs, nothosaurs, placodonts, sea turtles, thalattosaurs and thalattosuchians. Marine reptiles were less numerous after mass extinction at the end of the Cretaceous.
Birds
[edit]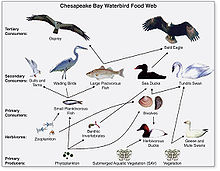
Marine birds are adapted to life within the marine environment. They are often called seabirds. While marine birds vary greatly in lifestyle, behaviour and physiology, they often exhibit striking convergent evolution, as the same environmental problems and feeding niches have resulted in similar adaptations. Examples include albatross, penguins, gannets, and auks.
In general, marine birds live longer, breed later and have fewer young than terrestrial birds do, but they invest a great deal of time in their young. Most species nest in colonies, which can vary in size from a few dozen birds to millions. Many species are famous for undertaking long annual migrations, crossing the equator or circumnavigating the Earth in some cases. They feed both at the ocean's surface and below it, and even feed on each other. Marine birds can be highly pelagic, coastal, or in some cases spend a part of the year away from the sea entirely. Some marine birds plummet from heights, plunging through the water leaving vapour-like trails, similar to that of fighter planes.[365] Gannets plunge into the water at up to 100 kilometres per hour (60 mph). They have air sacs under their skin in their face and chest which act like bubble-wrap, cushioning the impact with the water.
-
European herring gull attack herring schools from above.
-
Gentoo penguin swimming underwater
-
Albatrosses range over huge areas of ocean and some even circle the globe.
-
Gannets "divebomb" at high speed
The first marine birds evolved in the Cretaceous period, and modern marine bird families emerged in the Paleogene.
Mammals
[edit]
Mammals (from Latin for breast) are characterised by the presence of mammary glands which in females produce milk for feeding (nursing) their young. There are about 130 living and recently extinct marine mammal species such as seals, dolphins, whales, manatees, sea otters and polar bears.[366] They do not represent a distinct taxon or systematic grouping, but are instead unified by their reliance on the marine environment for feeding. Both cetaceans and sirenians are fully aquatic and therefore are obligate water dwellers. Seals and sea-lions are semiaquatic; they spend the majority of their time in the water, but need to return to land for important activities such as mating, breeding and molting. In contrast, both otters and the polar bear are much less adapted to aquatic living. Their diet varies considerably as well: some may eat zooplankton; others may eat fish, squid, shellfish, and sea-grass; and a few may eat other mammals.
In a process of convergent evolution, marine mammals, especially cetaceans such as dolphins and whales, redeveloped their body plan to parallel the streamlined fusiform body plan of pelagic fish. Front legs became flippers and back legs disappeared, a dorsal fin reappeared and the tail morphed into a powerful horizontal fluke. This body plan is an adaptation to being an active predator in a high drag environment. A parallel convergence occurred with the now extinct marine reptile ichthyosaur.[367]
-
Endangered blue whale, the largest living animal[368]
Primary producers
[edit]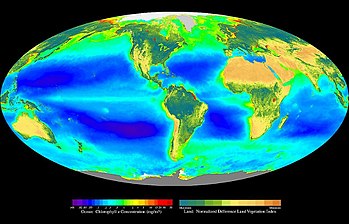
Primary producers are the autotroph organisms that make their own food instead of eating other organisms. This means primary producers become the starting point in the food chain for heterotroph organisms that do eat other organisms. Some marine primary producers are specialised bacteria and archaea which are chemotrophs, making their own food by gathering around hydrothermal vents and cold seeps and using chemosynthesis. However most marine primary production comes from organisms which use photosynthesis on the carbon dioxide dissolved in the water. This process uses energy from sunlight to convert water and carbon dioxide[370]: 186–187 into sugars that can be used both as a source of chemical energy and of organic molecules that are used in the structural components of cells.[370]: 1242 Marine primary producers are important because they underpin almost all marine animal life by generating most of the oxygen and food that provide other organisms with the chemical energy they need to exist.
The principal marine primary producers are cyanobacteria, algae and marine plants. The oxygen released as a by-product of photosynthesis is needed by nearly all living things to carry out cellular respiration. In addition, primary producers are influential in the global carbon and water cycles. They stabilize coastal areas and can provide habitats for marine animals. The term division has been traditionally used instead of phylum when discussing primary producers, but the International Code of Nomenclature for algae, fungi, and plants now accepts both terms as equivalents.[371]
Cyanobacteria
[edit]Cyanobacteria were the first organisms to evolve an ability to turn sunlight into chemical energy. They form a phylum (division) of bacteria which range from unicellular to filamentous and include colonial species. They are found almost everywhere on earth: in damp soil, in both freshwater and marine environments, and even on Antarctic rocks.[372] In particular, some species occur as drifting cells floating in the ocean, and as such were amongst the first of the phytoplankton.
The first primary producers that used photosynthesis were oceanic cyanobacteria about 2.3 billion years ago.[373][374] The release of molecular oxygen by cyanobacteria as a by-product of photosynthesis induced global changes in the Earth's environment. Because oxygen was toxic to most life on Earth at the time, this led to the near-extinction of oxygen-intolerant organisms, a dramatic change which redirected the evolution of the major animal and plant species.[375]
The tiny marine cyanobacterium Prochlorococcus, discovered in 1986, forms today part of the base of the ocean food chain and accounts for much of the photosynthesis of the open ocean[376] and an estimated 20% of the oxygen in the Earth's atmosphere.[377] It is possibly the most plentiful genus on Earth: a single millilitre of surface seawater may contain 100,000 cells or more.[378]
Originally, biologists classified cyanobacteria as algae, and referred to it as "blue-green algae". The more recent view is that cyanobacteria are bacteria, and hence are not even in the same Kingdom as algae. Most authorities today exclude all prokaryotes, and hence cyanobacteria from the definition of algae.[379][380]
Algae
[edit]Algae is an informal term for a widespread and diverse group of photosynthetic protists which are not necessarily closely related and are thus polyphyletic. Marine algae can be divided into six groups:
- green algae, an informal group containing about 8,000 recognised species.[381] Many species live most of their lives as single cells or are filamentous, while others form colonies made up from long chains of cells, or are highly differentiated macroscopic seaweeds.
- red algae, a (disputed) phylum containing about 7,000 recognised species,[382] mostly multicellular and including many notable seaweeds.[382][383]
- brown algae, a class containing about 2,000 recognised species,[384] mostly multicellular and including many seaweeds, including kelp
- diatoms, a (disputed) phylum containing about 100,000 recognised species of mainly unicellular algae. Diatoms generate about 20 percent of the oxygen produced on the planet each year,[147] take in over 6.7 billion metric tons of silicon each year from the waters in which they live,[385] and contribute nearly half of the organic material found in the oceans. The shells (frustules) of dead diatoms can reach as much as half a mile deep on the ocean floor.[386]
- dinoflagellates, a phylum of unicellular flagellates with about 2,000 marine species.[387] Many dinoflagellates are known to be photosynthetic, but a large fraction of these are in fact mixotrophic, combining photosynthesis with ingestion of prey (phagotrophy).[388] Some species are endosymbionts of marine animals and play an important part in the biology of coral reefs. Others predate other protozoa, and a few forms are parasitic.
- euglenophytes, a phylum of unicellular flagellates with only a few marine members
Unlike higher plants, algae lack roots, stems, or leaves. They can be classified by size as microalgae or macroalgae.
Microalgae are the microscopic types of algae, not visible to the naked eye. They are mostly unicellular species which exist as individuals or in chains or groups, though some are multicellular. Microalgae are important components of the marine protists (discussed above), as well as the phytoplankton (discussed below). They are very diverse. It has been estimated there are 200,000-800,000 species of which about 50,000 species have been described.[389] Depending on the species, their sizes range from a few micrometers (μm) to a few hundred micrometers. They are specially adapted to an environment dominated by viscous forces.
-
Chlamydomonas globosa, a unicellular green alga with two flagella just visible at bottom left
-
Centric diatom
-
Dinoflagellates
Macroalgae are the larger, multicellular and more visible types of algae, commonly called seaweeds. Seaweeds usually grow in shallow coastal waters where they are anchored to the seafloor by a holdfast. Seaweed that becomes adrift can wash up on beaches. Kelp is a large brown seaweed that forms large underwater forests covering about 25% of the world coastlines.[391] They are among the most productive and dynamic ecosystems on Earth.[392] Some Sargassum seaweeds are planktonic (free-floating). Like microalgae, macroalgae (seaweeds) are technically marine protists since they are not true plants.
-
Sargassum seaweed is a planktonic brown alga with air bladders that help it float.
-
Sargassum fish are camouflaged to live among drifting Sargassum seaweed.
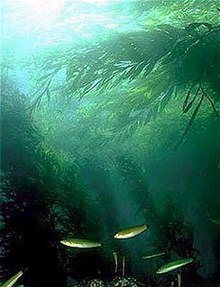
- Unicellular macroalgae (see also macroscopic protists ←)
-
The unicellular mermaid's wineglass are mushroom-shaped algae that grow up to 10 cm high.
-
Killer algae are single-celled organisms, but look like ferns and grow stalks up to 80 cm long.[394]
Unicellular organisms are usually microscopic, less than one tenth of a millimeter long. There are exceptions. Mermaid's wineglass, a genus of subtropical green algae, is single-celled but remarkably large and complex in form with a single large nucleus, making it a model organism for studying cell biology.[395] Another single celled algae, Caulerpa taxifolia, has the appearance of a vascular plant including "leaves" arranged neatly up stalks like a fern. Selective breeding in aquariums to produce hardier strains resulted in an accidental release into the Mediterranean where it has become an invasive species known colloquially as killer algae.[396]
Origin of plants
[edit]
Back in the Silurian, some phytoplankton evolved into red, brown and green algae. These algae then invaded the land and started evolving into the land plants we know today. Later, in the Cretaceous, some of these land plants returned to the sea as marine plants, such as mangroves and seagrasses.[397]
Marine plants can be found in intertidal zones and shallow waters, such as seagrasses like eelgrass and turtle grass, Thalassia. These plants have adapted to the high salinity of the ocean environment. Plant life can also flourish in the brackish waters of estuaries, where mangroves or cordgrass or beach grass beach grass might grow.
-
Sea dragons camouflaged to look like floating seaweed live in kelp forests and seagrass meadows.[398]
The total world area of mangrove forests was estimated in 2010 as 134,257 square kilometres (51,837 sq mi) (based on satellite data).[399][400] The total world area of seagrass meadows is more difficult to determine, but was conservatively estimated in 2003 as 177,000 square kilometres (68,000 sq mi).[401]
Mangroves and seagrasses provide important nursery habitats for marine life, acting as hiding and foraging places for larval and juvenile forms of larger fish and invertebrates.[402]
Plankton and trophic interactions
[edit]
Plankton (from Greek for wanderers) are a diverse group of organisms that live in the water column of large bodies of water but cannot swim against a current. As a result, they wander or drift with the currents.[403] Plankton are defined by their ecological niche, not by any phylogenetic or taxonomic classification. They are a crucial source of food for many marine animals, from forage fish to whales. Plankton can be divided into a plant-like component and an animal component.
Phytoplankton
[edit]Phytoplankton are the plant-like components of the plankton community ("phyto" comes from the Greek for plant). They are autotrophic (self-feeding), meaning they generate their own food and do not need to consume other organisms.
Phytoplankton consist mainly of microscopic photosynthetic eukaryotes which inhabit the upper sunlit layer in all oceans. They need sunlight so they can photosynthesize. Most phytoplankton are single-celled algae, but other phytoplankton are bacteria and some are protists.[404] Phytoplankton groups include cyanobacteria (above), diatoms, various other types of algae (red, green, brown, and yellow-green), dinoflagellates, euglenoids, coccolithophorids, cryptomonads, chrysophytes, chlorophytes, prasinophytes, and silicoflagellates. They form the base of the primary production that drives the ocean food web, and account for half of the current global primary production, more than the terrestrial forests.[405]
- Phytoplankton
-
Phytoplankton are the foundation of the ocean food chain.
-
Phytoplankton come in many shapes and sizes.
-
Diatoms are one of the most common types of phytoplankton.
-
Colonial phytoplankton
-
The cyanobacterium Prochlorococcus accounts for much of the ocean's primary production.
-
Green cyanobacteria scum washed up on a rock in California
-
Gyrodinium, one of the few naked dinoflagellates which lack armour
-
Zoochlorellae (green) living inside the ciliate Stichotricha secunda
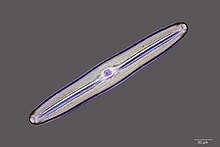
-
Coccolithophores named after the BBC documentary series. The Blue Planet
-
Algae bloom of Emiliania huxleyi off the southern coast of England
-
Guinardia delicatula, a diatom responsible for algal blooms in the North Sea and the English Channel[407]
Zooplankton
[edit]Zooplankton are the animal component of the planktonic community ("zoo" comes from the Greek for animal). They are heterotrophic (other-feeding), meaning they cannot produce their own food and must consume instead other plants or animals as food. In particular, this means they eat phytoplankton.
Zooplankton are generally larger than phytoplankton, mostly still microscopic but some can be seen with the naked eye. Many protozoans (single-celled protists that prey on other microscopic life) are zooplankton, including zooflagellates, foraminiferans, radiolarians and some dinoflagellates. Other dinoflagellates are mixotrophic and could also be classified as phytoplankton; the distinction between plants and animals often breaks down in very small organisms. Other zooplankton include pelagic cnidarians, ctenophores, molluscs, arthropods and tunicates, as well as planktonic arrow worms and bristle worms.
Radiolarians are unicellular protists with elaborate silica shells
Microzooplankton: major grazers of the plankton
-
Radiolarians come in many shapes.
-
Group of planktic foraminiferans
-
Copepods eat phytoplankton. This one is carrying eggs.
-
The dinoflagellate, Protoperidinium extrudes a large feeding veil to capture prey.
Larger zooplankton can be predatory on smaller zooplankton.
Macrozooplankton
-
Marine amphipod
-
Pelagic sea cucumber
External videos | |
---|---|
![]() |
Many marine animals begin life as zooplankton in the form of eggs or larvae, before they develop into adults. These are meroplanktic, that is, they are planktonic for only part of their life.
- Larvae and juveniles
-
Salmon larva hatching from its egg
-
Ocean sunfish larva
-
Juvenile planktonic squid
-
Larva stage of a spiny lobster
Mixotrophic plankton
[edit]Dinoflagellates are often mixotrophic or live in symbiosis with other organisms.
- Mixoplankton
-
Tintinnid ciliate Favella
-
Euglena mutabilis, a photosynthetic flagellate
-
Noctiluca scintillans, a bioluminescence dinoflagellate
Some dinoflagellates are bioluminescent. At night, ocean water can light up internally and sparkle with blue light because of these dinoflagellates.[411][412] Bioluminescent dinoflagellates possess scintillons, individual cytoplasmic bodies which contain dinoflagellate luciferase, the main enzyme involved in the luminescence. The luminescence, sometimes called the phosphorescence of the sea, occurs as brief (0.1 sec) blue flashes or sparks when individual scintillons are stimulated, usually by mechanical disturbances from, for example, a boat or a swimmer or surf.[413]
Marine food web
[edit]
Compared to terrestrial environments, marine environments have biomass pyramids which are inverted at the base. In particular, the biomass of consumers (copepods, krill, shrimp, forage fish) is larger than the biomass of primary producers. This happens because the ocean's primary producers are tiny phytoplankton which tend to be r-strategists that grow and reproduce rapidly, so a small mass can have a fast rate of primary production. In contrast, terrestrial primary producers, such as mature forests, are often K-strategists that grow and reproduce slowly, so a much larger mass is needed to achieve the same rate of primary production.
Because of this inversion, it is the zooplankton that make up most of the marine animal biomass. As primary consumers, they are the crucial link between the primary producers (mainly phytoplankton) and the rest of the marine food web (secondary consumers).[414]
If phytoplankton dies before it is eaten, it descends through the euphotic zone as part of the marine snow and settles into the depths of sea. In this way, phytoplankton sequester about 2 billion tons of carbon dioxide into the ocean each year, causing the ocean to become a sink of carbon dioxide holding about 90% of all sequestered carbon.[415]
In 2010 researchers found whales carry nutrients from the depths of the ocean back to the surface using a process they called the whale pump.[416] Whales feed at deeper levels in the ocean where krill is found, but return regularly to the surface to breathe. There whales defecate a liquid rich in nitrogen and iron. Instead of sinking, the liquid stays at the surface where phytoplankton consume it. In the Gulf of Maine the whale pump provides more nitrogen than the rivers.[417]
Other interactions
[edit]Biogeochemical cycles
[edit]Taken as a whole, the oceans form a single marine system where water – the "universal solvent" [418] – dissolves nutrients and substances containing elements such as oxygen, carbon, nitrogen and phosphorus. These substances are endlessly cycled and recycled, chemically combined and then broken down again, dissolved and then precipitated or evaporated, imported from and exported back to the land and the atmosphere and the ocean floor. Powered both by the biological activity of marine organisms and by the natural actions of the sun and tides and movements within the Earth's crust, these are the marine biogeochemical cycles.[419][420]
-
Marine phosphorus cycle
Sediments and biogenic ooze
[edit]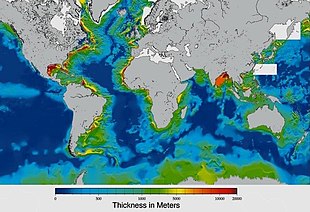
Sediments at the bottom of the ocean have two main origins, terrigenous and biogenous. Terrigenous sediments account for about 45% of the total marine sediment, and originate in the erosion of rocks on land, transported by rivers and land runoff, windborne dust, volcanoes, or grinding by glaciers.
Biogenous sediments account for the other 55% of the total sediment, and originate in the skeletal remains of marine protists (single-celled plankton and benthos organisms). Much smaller amounts of precipitated minerals and meteoric dust can also be present. Ooze, in the context of a marine sediment, does not refer to the consistency of the sediment but to its biological origin. The term ooze was originally used by John Murray, the "father of modern oceanography", who proposed the term radiolarian ooze for the silica deposits of radiolarian shells brought to the surface during the Challenger Expedition.[422] A biogenic ooze is a pelagic sediment containing at least 30 percent from the skeletal remains of marine organisms.
Main types of biogenic ooze
| |||||||
---|---|---|---|---|---|---|---|
type | mineral forms |
protist responsible |
name of skeleton |
description | |||
Siliceous ooze | SiO2 quartz glass opal chert |
diatoms | ![]() |
frustule | Individual diatoms range in size from 0.002 to 0.2 mm.[423] | ||
radiolarians | ![]() |
skeleton | Radiolarians are protozoa with diameters typically between 0.1 and 0.2 mm that produce intricate mineral skeletons, usually made of silica | ||||
Calcareous ooze | CaCO3 calcite aragonite limestone chalk |
foraminiferans | ![]() |
test | There are about 10,000 living species of foraminiferans,[424] usually under 1 mm in size. | ||
coccolithophores | ![]() |
coccolith | Coccolithophores are spherical cells usually less than 0.1 mm across, enclosed by calcareous plates called coccoliths.[425] Coccoliths are important microfossils. They are the largest global source of biogenic calcium carbonate, and make significant contributions to the global carbon cycle.[426] They are the main constituent of chalk deposits such as the white cliffs of Dover. |
-
An elaborate mineral skeleton of a radiolarian made of silica.
-
Diatoms, major components of marine plankton, also have silica skeletons called frustules.
-
Coccolithophores have plates or scales made with calcium carbonate called coccoliths
-
A diatom microfossil from 40 million years ago
-
Diatomaceous earth is a soft, siliceous, sedimentary rock made up of microfossils in the form of the frustules (shells) of single cell diatoms (click to magnify).
-
Illustration of a Globigerina ooze
-
Shells (tests), usually made of calcium carbonate, from a foraminiferal ooze on the deep ocean floor
Land interactions
[edit]
Land interactions impact marine life in many ways. Coastlines typically have continental shelves extending some way from the shore. These provide extensive shallows sunlit down to the seafloor, allowing for photosynthesis and enabling habitats for seagrass meadows, coral reefs, kelp forests and other benthic life. Further from shore the continental shelf slopes towards deep water. Wind blowing at the ocean surface or deep ocean currents can result in cold and nutrient rich waters from abyssal depths moving up the continental slopes. This can result in upwellings along the outer edges of continental shelves, providing conditions for phytoplankton blooms.
Water evaporated by the sun from the surface of the ocean can precipitate on land and eventually return to the ocean as runoff or discharge from rivers, enriched with nutrients as well as pollutants. As rivers discharge into estuaries, freshwater mixes with saltwater and becomes brackish. This provides another shallow water habitat where mangrove forests and estuarine fish thrive. Overall, life in inland lakes can evolve with greater diversity than happens in the sea, because freshwater habitats are themselves diverse and compartmentalised in a way marine habitats are not. Some aquatic life, such as salmon and eels, migrate back and forth between freshwater and marine habitats. These migrations can result in exchanges of pathogens and have impacts on the way life evolves in the ocean.
Anthropogenic impacts
[edit]
Human activities affect marine life and marine habitats through overfishing, pollution, acidification and the introduction of invasive species. These impact marine ecosystems and food webs and may result in consequences as yet unrecognised for the biodiversity and continuation of marine life forms.[428]
Biodiversity and extinction events
[edit]
Biodiversity is the result of over three billion years of evolution. Until approximately 600 million years ago, all life consisted of archaea, bacteria, protozoans and similar single-celled organisms. The history of biodiversity during the Phanerozoic (the last 540 million years), starts with rapid growth during the Cambrian explosion – a period during which nearly every phylum of multicellular organisms first appeared. Over the next 400 million years or so, invertebrate diversity showed little overall trend and vertebrate diversity shows an overall exponential trend.[430]
However, more than 99 percent of all species that ever lived on Earth, amounting to over five billion species,[431] are estimated to be extinct.[432][433] These extinctions occur at an uneven rate. The dramatic rise in diversity has been marked by periodic, massive losses of diversity classified as mass extinction events.[430] Mass extinction events occur when life undergoes precipitous global declines. Most diversity and biomass on earth is found among the microorganisms, which are difficult to measure. Recorded extinction events are therefore based on the more easily observed changes in the diversity and abundance of larger multicellular organisms, rather than the total diversity and abundance of life.[434] Marine fossils are mostly used to measure extinction rates because of their superior fossil record and stratigraphic range compared to land organisms.
Based on the fossil record, the background rate of extinctions on Earth is about two to five taxonomic families of marine animals every million years. The Great Oxygenation Event was perhaps the first major extinction event. Since the Cambrian explosion five major mass extinctions have significantly exceeded the background extinction rate.[435] The worst was the Permian-Triassic extinction event, 251 million years ago. One generally estimates that the Big Five mass extinctions of the Phanerozoic (the last 540 million years) wiped out more than 40% of marine genera and probably more than 70% of marine species.[436] The current Holocene extinction caused by human activity, and now referred to as the "sixth extinction", may prove ultimately more devastating.
Investigating and Exploring Marine Life
[edit]Research and study
[edit]In order to perform research and enrich Marine Life knowledge, Scientists use various methods in-order to reach and explore the depths of the ocean. several Hi-tech instruments and vehicles are used for this purpose. [437]
- Autonomous Underwater Vehicles (AUVs)- Underwater robots used to explore the ocean. AUVs are independent robots and can explore unmanned. They are released from a ship and are operated from the surface. [438]
- Deep-Towed Vehicles (DTVs)- vehicles towed behind research vessels, offering a simpler alternative to more advanced underwater vehicles. They serve as versatile platforms for deploying oceanographic instruments to measure various ocean parameters, with specific models like the DTV BRIDGET used for studying hydrothermal vent plumes by moving near the ocean floor.[439]
- Manned Submersibles- an manned underwater vehicle used for exploring, experimenting and is often used by army. [437]
- Research vessels (R/Vs)- a boat or ship used to conduct research over a ling period of time. It is capable of transporting a diverse range of sampling and surveying equipment. Research vessels typically feature on-board laboratory space, allowing researchers to promptly analyze the materials collected during cruises.
- Remotely Operated Vehicles (ROVs)- unmanned vehicles. able to reach greater depths under water in order to collect a wider variety of information. [437][440]
See also
[edit]- Blue Planet – 2001 British nature documentary television series - David Attenborough
- Blue Planet II – 2017 British nature documentary television series
- Census of Marine Life – 10 year international marine biological program
- Colonization of land
- Marine larval ecology
- Taxonomy of invertebrates – System of classification of animals with emphasis on the invertebrates
Notes
[edit]- ^ This is the measurement taken by the vessel Kaikō in March 1995 and is considered the most accurate measurement to date. See the Challenger Deep article for more details.
- ^ Myxozoa were thought to be an exception, but are now thought to be heavily modified members of the Cnidaria. Jiménez-Guri E, Philippe H, Okamura B, Holland PW (July 2007). "Buddenbrockia is a cnidarian worm". Science. 317 (5834): 116–8. Bibcode:2007Sci...317..116J. doi:10.1126/science.1142024. PMID 17615357. S2CID 5170702.
References
[edit]- ^ Jump up to: a b Cavicchioli R, Ripple WJ, Timmis KN, Azam F, Bakken LR, Baylis M, et al. (September 2019). "Scientists' warning to humanity: microorganisms and climate change". Nature Reviews. Microbiology. 17 (9): 569–586. doi:10.1038/s41579-019-0222-5. PMC 7136171. PMID 31213707.
Modified text was copied from this source, which is available under a Creative Commons Attribution 4.0 International License.
- ^ Jump up to: a b c "National Oceanic and Atmospheric Administration – Ocean". NOAA. Retrieved 20 February 2019.
- ^ "Tiny Fish May Be Ancestor of Nearly All Living Vertebrates". Live Science. 11 June 2014.
- ^ Drogin, B (2 August 2009). "Mapping an ocean of species". Los Angeles Times. Retrieved 18 August 2009.
- ^ Bouchet, Philippe; Decock, Wim; Lonneville, Britt; Vanhoorne, Bart; Vandepitte, Leen (June 2023). "Marine biodiversity discovery: the metrics of new species descriptions". Frontiers in Marine Science. 10 (3389). doi:10.3389/fmars.2023.929989 – via ResearchGate.
- ^ Paul, GS (2010). "The Evolution of Dinosaurs and their World". The Princeton Field Guide to Dinosaurs. Princeton: Princeton University Press. p. 19. ISBN 978-0-691-13720-9.
- ^ Bortolotti, Dan (2008). Wild blue: a natural history of the world's largest animal. New York: Thomas Dunn Books. ISBN 978-0-312-38387-9. OCLC 213451450.
- ^ Jump up to: a b c Bar-On YM, Phillips R, Milo R (June 2018). "The biomass distribution on Earth". Proceedings of the National Academy of Sciences of the United States of America. 115 (25): 6506–6511. Bibcode:2018PNAS..115.6506B. doi:10.1073/pnas.1711842115. PMC 6016768. PMID 29784790.
- ^ "Census Of Marine Life". Smithsonian. 30 April 2018. Retrieved 29 October 2020.
- ^ Xiao-Feng P (2014). "Chapter 5". Water: Molecular Structure And Properties. World Scientific. pp. 390–461. ISBN 9789814440448.
- ^ Greenwood NN, Earnshaw A (1997). Chemistry of the Elements (2nd ed.). Butterworth-Heinemann. p. 620. ISBN 978-0-08-037941-8.
- ^ "Water, the Universal Solvent". USGS. Archived from the original on 9 July 2017. Retrieved 27 June 2017.
- ^ Reece JB (31 October 2013). Campbell Biology (10 ed.). Pearson. p. 48. ISBN 9780321775658.
- ^ Reece JB (31 October 2013). Campbell Biology (10 ed.). Pearson. p. 44. ISBN 9780321775658.
- ^ Collins JC (1991). The Matrix of Life: A View of Natural Molecules from the Perspective of Environmental Water. Molecular Presentations. ISBN 978-0-9629719-0-7.
- ^ "7,000 m Class Remotely Operated Vehicle KAIKO 7000". Japan Agency for Marine-Earth Science and Technology (JAMSTEC). Archived from the original on 10 April 2020. Retrieved 7 June 2008.
- ^ "How many oceans are there?". NOAA. 9 April 2020.
- ^ Charette MA, Smith WH (June 2010). "The Volume of Earth's Ocean". Oceanography. 23 (2): 112–14. doi:10.5670/oceanog.2010.51. hdl:1912/3862.
- ^ Duxbury AC, Cenedese C (7 May 2021). "Sphere depth of the ocean – hydrology". Encyclopædia Britannica. Retrieved 12 April 2015.
- ^ "Third rock from the Sun – restless Earth". NASA's Cosmos. Retrieved 12 April 2015.
- ^ Perlman H (17 March 2014). "The World's Water". USGS Water-Science School. Retrieved 12 April 2015.
- ^ Kennish KJ (2001). Practical handbook of marine science. Marine science series (3rd ed.). CRC Press. p. 35. ISBN 978-0-8493-2391-1.
- ^ "Why is the ocean salty?".
- ^ Mullen L (11 June 2002). "Salt of the Early Earth". NASA Astrobiology Magazine. Archived from the original on 30 June 2007. Retrieved 14 March 2007.
- ^ Morris RM. "Oceanic Processes". NASA Astrobiology Magazine. Archived from the original on 15 April 2009. Retrieved 14 March 2007.
- ^ Scott M (24 April 2006). "Earth's Big heat Bucket". NASA Earth Observatory. Retrieved 14 March 2007.
- ^ Sample S (21 June 2005). "Sea Surface Temperature". NASA. Archived from the original on 6 April 2013. Retrieved 21 April 2007.
- ^ "Volumes of the World's Oceans from ETOPO1". NOAA. Archived from the original on 11 March 2015. Retrieved 20 February 2019.
{{cite web}}
: CS1 maint: bot: original URL status unknown (link) - ^ "Planet "Earth": We Should Have Called It "Sea"". Quote Invertigator. 25 January 2017.
- ^ "Unveiling Planet Ocean". pNASA Science. 14 March 2002. Archived from the original on 8 October 2022. Retrieved 19 February 2019.
- ^ Dyches P, Brown D (12 May 2015). "NASA Research Reveals Europa's Mystery Dark Material Could Be Sea Salt". NASA. Retrieved 12 May 2015.
- ^ Adamu Z (1 October 2012). "Water near surface of a Jupiter moon only temporary". CNN Light Years. Archived from the original on 5 October 2012. Retrieved 24 April 2019.
- ^ Tritt CS (2002). "Possibility of Life on Europa". Milwaukee School of Engineering. Archived from the original on 9 June 2007. Retrieved 10 August 2007.
- ^ Schulze-Makuch D, Irwin LN (2001). "Alternative Energy Sources Could Support Life on Europa" (PDF). Departments of Geological and Biological Sciences, University of Texas at El Paso. Archived from the original (PDF) on 3 July 2006. Retrieved 21 December 2007.
- ^ Friedman L (14 December 2005). "Projects: Europa Mission Campaign". The Planetary Society. Archived from the original on 11 August 2011. Retrieved 8 August 2011.
- ^ "Ocean Within Enceladus May Harbor Hydrothermal Activity". NASA Press Release. 11 March 2015.
- ^ "Age of the Earth". United States Geological Survey. 9 July 2007. Retrieved 31 May 2015.
- ^ Dalrymple GB (January 2001). "The age of the Earth in the twentieth century: a problem (mostly) solved". Special Publications. 190 (1). London: Geological Society: 205–21. Bibcode:2001GSLSP.190..205D. doi:10.1144/GSL.SP.2001.190.01.14. S2CID 130092094.
- ^ Manhes G, Allègre CJ, Dupré B, Hamelin B (May 1980). "Lead isotope study of basic-ultrabasic layered complexes: Speculations about the age of the earth and primitive mantle characteristics". Earth and Planetary Science Letters. 47 (3): 370–382. Bibcode:1980E&PSL..47..370M. doi:10.1016/0012-821X(80)90024-2. ISSN 0012-821X.
- ^ Schopf JW, Kudryavtsev AB, Czaja AD, Tripathi AB (5 October 2007). "Evidence of Archean life: Stromatolites and microfossils". Precambrian Research. 158 (3–4): 141–155. Bibcode:2007PreR..158..141S. doi:10.1016/j.precamres.2007.04.009. ISSN 0301-9268.
- ^ Raven PH, Johnson GB (2002). Biology (6th ed.). Boston: McGraw-Hill. p. 68. ISBN 978-0-07-112261-0.
- ^ Baumgartner RJ, Van Kranendonk MJ, Wacey D, Fiorentini ML, Saunders M, Caruso S, et al. (2019). "Nano−porous pyrite and organic matter in 3.5-billion-year-old stromatolites record primordial life" (PDF). Geology. 47 (11): 1039–1043. Bibcode:2019Geo....47.1039B. doi:10.1130/G46365.1. S2CID 204258554.
- ^ "Earliest signs of life: Scientists find microbial remains in ancient rocks". Phys.org. 26 September 2019.
- ^ Ohtomo Y, Kakegawa T, Ishida A, Nagase T, Rosing MT (January 2014). "Evidence for biogenic graphite in early Archaean Isua metasedimentary rocks". Nature Geoscience. 7 (1): 25–28. Bibcode:2014NatGe...7...25O. doi:10.1038/ngeo2025. ISSN 1752-0894.
- ^ Jump up to: a b Borenstein S (19 October 2015). "Hints of life on what was thought to be desolate early Earth". Associated Press. Retrieved 9 October 2018.
- ^ Bell EA, Boehnke P, Harrison TM, Mao WL (November 2015). "Potentially biogenic carbon preserved in a 4.1 billion-year-old zircon". Proceedings of the National Academy of Sciences of the United States of America. 112 (47): 14518–21. Bibcode:2015PNAS..11214518B. doi:10.1073/pnas.1517557112. PMC 4664351. PMID 26483481.
- ^ Penny D, Poole A (December 1999). "The nature of the last universal common ancestor". Current Opinion in Genetics & Development. 9 (6): 672–7. doi:10.1016/S0959-437X(99)00020-9. PMID 10607605.
- ^ Theobald DL (May 2010). "A formal test of the theory of universal common ancestry". Nature. 465 (7295): 219–22. Bibcode:2010Natur.465..219T. doi:10.1038/nature09014. PMID 20463738. S2CID 4422345.
- ^ Doolittle WF (February 2000). "Uprooting the tree of life" (PDF). Scientific American. 282 (2): 90–5. Bibcode:2000SciAm.282b..90D. doi:10.1038/scientificamerican0200-90. PMID 10710791. Archived from the original (PDF) on 7 September 2006. Retrieved 5 April 2015.
- ^ Peretó J (March 2005). "Controversies on the origin of life" (PDF). International Microbiology. 8 (1): 23–31. PMID 15906258. Archived from the original (PDF) on 24 August 2015.
- ^ Joyce GF (July 2002). "The antiquity of RNA-based evolution". Nature. 418 (6894): 214–21. Bibcode:2002Natur.418..214J. doi:10.1038/418214a. PMID 12110897. S2CID 4331004.
- ^ Trevors JT, Psenner R (December 2001). "From self-assembly of life to present-day bacteria: a possible role for nanocells". FEMS Microbiology Reviews. 25 (5): 573–82. doi:10.1111/j.1574-6976.2001.tb00592.x. PMID 11742692.
- ^ Wade N (25 July 2016). "Meet Luca, the Ancestor of All Living Things". New York Times. Retrieved 25 July 2016.
- ^ Bapteste E, Walsh DA (June 2005). "Does the 'Ring of Life' ring true?". Trends in Microbiology. 13 (6): 256–61. doi:10.1016/j.tim.2005.03.012. PMID 15936656.
- ^ Darwin C (1859). "On The Origin of the Species". London: John Murray.
- ^ Doolittle WF, Bapteste E (February 2007). "Pattern pluralism and the Tree of Life hypothesis". Proceedings of the National Academy of Sciences of the United States of America. 104 (7): 2043–9. Bibcode:2007PNAS..104.2043D. doi:10.1073/pnas.0610699104. PMC 1892968. PMID 17261804.
- ^ Kunin V, Goldovsky L, Darzentas N, Ouzounis CA (July 2005). "The net of life: reconstructing the microbial phylogenetic network". Genome Research. 15 (7): 954–9. doi:10.1101/gr.3666505. PMC 1172039. PMID 15965028.
- ^ Jablonski D (June 1999). "The future of the fossil record". Science. 284 (5423): 2114–6. doi:10.1126/science.284.5423.2114. PMID 10381868. S2CID 43388925.
- ^ Ciccarelli FD, Doerks T, von Mering C, Creevey CJ, Snel B, Bork P (March 2006). "Toward automatic reconstruction of a highly resolved tree of life". Science. 311 (5765): 1283–7. Bibcode:2006Sci...311.1283C. CiteSeerX 10.1.1.381.9514. doi:10.1126/science.1123061. PMID 16513982. S2CID 1615592.
- ^ Mason SF (6 September 1984). "Origins of biomolecular handedness". Nature. 311 (5981): 19–23. Bibcode:1984Natur.311...19M. doi:10.1038/311019a0. PMID 6472461. S2CID 103653.
- ^ Wolf YI, Rogozin IB, Grishin NV, Koonin EV (September 2002). "Genome trees and the tree of life". Trends in Genetics. 18 (9): 472–9. doi:10.1016/S0168-9525(02)02744-0. PMID 12175808.
- ^ Varki A, Altheide TK (December 2005). "Comparing the human and chimpanzee genomes: searching for needles in a haystack". Genome Research. 15 (12): 1746–58. doi:10.1101/gr.3737405. PMID 16339373.
- ^ Jump up to: a b Cavalier-Smith T (June 2006). "Cell evolution and Earth history: stasis and revolution". Philosophical Transactions of the Royal Society of London. Series B, Biological Sciences. 361 (1470): 969–1006. doi:10.1098/rstb.2006.1842. PMC 1578732. PMID 16754610.
- ^ Schopf JW (June 2006). "Fossil evidence of Archaean life". Philosophical Transactions of the Royal Society of London. Series B, Biological Sciences. 361 (1470): 869–85. doi:10.1098/rstb.2006.1834. PMC 1578735. PMID 16754604.
- Altermann W, Kazmierczak J (November 2003). "Archean microfossils: a reappraisal of early life on Earth". Research in Microbiology. 154 (9): 611–7. doi:10.1016/j.resmic.2003.08.006. PMID 14596897.
- ^ Jump up to: a b Schopf JW (July 1994). "Disparate rates, differing fates: tempo and mode of evolution changed from the Precambrian to the Phanerozoic". Proceedings of the National Academy of Sciences of the United States of America. 91 (15): 6735–42. Bibcode:1994PNAS...91.6735S. doi:10.1073/pnas.91.15.6735. PMC 44277. PMID 8041691.
- ^ Jump up to: a b Poole AM, Penny D (January 2007). "Evaluating hypotheses for the origin of eukaryotes". BioEssays. 29 (1): 74–84. doi:10.1002/bies.20516. PMID 17187354.
- ^ Jump up to: a b Dyall SD, Brown MT, Johnson PJ (April 2004). "Ancient invasions: from endosymbionts to organelles". Science. 304 (5668): 253–7. Bibcode:2004Sci...304..253D. doi:10.1126/science.1094884. PMID 15073369. S2CID 19424594.
- ^ Martin W (October 2005). "The missing link between hydrogenosomes and mitochondria". Trends in Microbiology. 13 (10): 457–9. doi:10.1016/j.tim.2005.08.005. PMID 16109488.
- ^ Lang BF, Gray MW, Burger G (December 1999). "Mitochondrial genome evolution and the origin of eukaryotes". Annual Review of Genetics. 33: 351–97. doi:10.1146/annurev.genet.33.1.351. PMID 10690412.
- McFadden GI (December 1999). "Endosymbiosis and evolution of the plant cell". Current Opinion in Plant Biology. 2 (6): 513–9. doi:10.1016/S1369-5266(99)00025-4. PMID 10607659.
- ^ DeLong EF, Pace NR (August 2001). "Environmental diversity of bacteria and archaea". Systematic Biology. 50 (4): 470–8. CiteSeerX 10.1.1.321.8828. doi:10.1080/106351501750435040. PMID 12116647.
- ^ Kaiser D (December 2001). "Building a multicellular organism". Annual Review of Genetics. 35: 103–23. doi:10.1146/annurev.genet.35.102401.090145. PMID 11700279. S2CID 18276422.
- ^ Zimmer C (7 January 2016). "Genetic Flip Helped Organisms Go From One Cell to Many". The New York Times. Retrieved 7 January 2016.
- ^ Valentine JW, Jablonski D, Erwin DH (February 1999). "Fossils, molecules and embryos: new perspectives on the Cambrian explosion". Development. 126 (5): 851–9. doi:10.1242/dev.126.5.851. PMID 9927587.
- ^ Ohno S (January 1997). "The reason for as well as the consequence of the Cambrian explosion in animal evolution". Journal of Molecular Evolution. 44 (Suppl. 1): S23-7. Bibcode:1997JMolE..44S..23O. doi:10.1007/PL00000055. PMID 9071008. S2CID 21879320.
- Valentine JW, Jablonski D (2003). "Morphological and developmental macroevolution: a paleontological perspective". The International Journal of Developmental Biology. 47 (7–8): 517–22. PMID 14756327. Retrieved 30 December 2014.
- ^ Wellman CH, Osterloff PL, Mohiuddin U (September 2003). "Fragments of the earliest land plants" (PDF). Nature. 425 (6955): 282–5. Bibcode:2003Natur.425..282W. doi:10.1038/nature01884. PMID 13679913. S2CID 4383813.
- ^ Barton N (2007). Evolution. CSHL Press. pp. 273–274. ISBN 9780199226320. Retrieved 30 September 2012.
- ^ Waters ER (December 2003). "Molecular adaptation and the origin of land plants". Molecular Phylogenetics and Evolution. 29 (3): 456–63. doi:10.1016/j.ympev.2003.07.018. PMID 14615186.
- ^ Mayhew PJ (August 2007). "Why are there so many insect species? Perspectives from fossils and phylogenies". Biological Reviews of the Cambridge Philosophical Society. 82 (3): 425–54. doi:10.1111/j.1469-185X.2007.00018.x. PMID 17624962. S2CID 9356614.
- ^ Carroll RL (May 2007). "The Palaeozoic Ancestry of Salamanders, Frogs and Caecilians". Zoological Journal of the Linnean Society. 150 (Supplement s1): 1–140. doi:10.1111/j.1096-3642.2007.00246.x. ISSN 1096-3642.
- ^ Wible JR, Rougier GW, Novacek MJ, Asher RJ (June 2007). "Cretaceous eutherians and Laurasian origin for placental mammals near the K/T boundary". Nature. 447 (7147): 1003–6. Bibcode:2007Natur.447.1003W. doi:10.1038/nature05854. PMID 17581585. S2CID 4334424.
- ^ Witmer LM (July 2011). "Palaeontology: An icon knocked from its perch". Nature. 475 (7357): 458–9. doi:10.1038/475458a. PMID 21796198. S2CID 205066360.
- ^ Schloss PD, Handelsman J (December 2004). "Status of the microbial census". Microbiology and Molecular Biology Reviews. 68 (4): 686–91. doi:10.1128/MMBR.68.4.686-691.2004. PMC 539005. PMID 15590780.
- ^ Миллер Г.Т., Спулман С. (январь 2012 г.). «Глава 4.1: Что такое биоразнообразие и почему оно важно?» . Экологическая наука . Cengage Обучение. п. 62. ИСБН 978-1-133-70787-5 .
- ^ Мора С, Титтенсор ДП, Адл С, Симпсон А.Г., Червь Б (август 2011 г.). «Сколько видов существует на Земле и в океане?» . ПЛОС Биология . 9 (8): e1001127. дои : 10.1371/journal.pbio.1001127 . ПМК 3160336 . ПМИД 21886479 .
- ^ Мэдиган М., Мартинко Дж., ред. (2006). Брок Биология микроорганизмов (13-е изд.). Пирсон Образование. п. 1096. ИСБН 978-0-321-73551-5 .
- ^ Рыбицкий Е.П. (1990). «Классификация организмов на пороге жизни, или Проблемы вирусной систематики». Южноафриканский научный журнал . 86 : 182–6. ISSN 0038-2353 .
- ^ Львофф А. (октябрь 1957 г.). «Понятие вируса» . Журнал общей микробиологии . 17 (2): 239–53. дои : 10.1099/00221287-17-2-239 . ПМИД 13481308 .
- ^ «Данные ВОЗ о смертности за 2002 год» . Архивировано из оригинала 19 августа 2006 года . Проверено 20 января 2007 г.
- ^ «Функции микробиома глобального океана являются ключом к пониманию изменений окружающей среды» . www.sciencedaily.com . Университет Джорджии. 10 декабря 2015 года . Проверено 11 декабря 2015 г.
- ^ Саттл, Калифорния (сентябрь 2005 г.). «Вирусы в море». Природа . 437 (7057): 356–61. Бибкод : 2005Natur.437..356S . дои : 10.1038/nature04160 . ПМИД 16163346 . S2CID 4370363 .
- ^ Перейти обратно: а б Шорс Т (2017). Понимание вирусов (3-е изд.). Издательство Джонс и Бартлетт. ISBN 978-1-284-02592-7 .
- ^ Перейти обратно: а б с Саттл, Калифорния (октябрь 2007 г.). «Морские вирусы — основные игроки глобальной экосистемы». Обзоры природы. Микробиология . 5 (10): 801–12. дои : 10.1038/nrmicro1750 . ПМИД 17853907 . S2CID 4658457 .
- ^ Моррисон Дж. (11 января 2016 г.). «Живые бактерии движутся в воздушных потоках Земли» . Смитсоновский журнал .
- ^ Роббинс Дж. (13 апреля 2018 г.). «Триллионы и триллионы вирусов падают с неба каждый день» . Нью-Йорк Таймс . Проверено 14 апреля 2018 г.
- ^ Рече И., Д'Орта Г., Младенов Н., Вингет Д.М., Саттл, Калифорния (апрель 2018 г.). «Скорость осаждения вирусов и бактерий над пограничным слоем атмосферы» . Журнал ISME . 12 (4): 1154–1162. дои : 10.1038/s41396-017-0042-4 . ПМК 5864199 . ПМИД 29379178 .
- ^ Персонал (2014). «Биосфера» . Аспенский институт глобальных изменений . Архивировано из оригинала 2 сентября 2010 года . Проверено 10 ноября 2014 г.
- ^ Перейти обратно: а б с Чой CQ (17 марта 2013 г.). «Микробы процветают в самом глубоком месте на Земле» . ЖиваяНаука . Проверено 17 марта 2013 г.
- ^ Глуд Р.Н., Венцхёфер Ф., Мидделбо М., Огури К., Турневич Р., Кэнфилд Д.Э., Китазато Х (17 марта 2013 г.). «Высокие темпы микробного круговорота углерода в отложениях самой глубокой океанической впадины на Земле». Природа Геонауки . 6 (4): 284–288. Бибкод : 2013NatGe...6..284G . дои : 10.1038/ngeo1773 .
- ^ Оськин Б. (14 марта 2013 г.). «Инопланетяне: жизнь процветает на дне океана» . ЖиваяНаука . Проверено 17 марта 2013 г.
- ^ Морель Р. (15 декабря 2014 г.). «Проанализированы микробы, обнаруженные с помощью самой глубоководной морской буровой установки» . Новости Би-би-си . Проверено 15 декабря 2014 г.
- ^ Такай К., Накамура К., Токи Т., Цуногай У., Миядзаки М., Миядзаки Дж. и др. (август 2008 г.). «Пролиферация клеток при 122 градусах Цельсия и производство изотопно-тяжелого CH4 гипертермофильным метаногеном при культивировании под высоким давлением» . Труды Национальной академии наук Соединенных Штатов Америки . 105 (31): 10949–54. Бибкод : 2008PNAS..10510949T . дои : 10.1073/pnas.0712334105 . ПМК 2490668 . ПМИД 18664583 .
- ^ Фокс Д. (август 2014 г.). «Озера подо льдом: тайный сад Антарктиды» . Природа . 512 (7514): 244–6. Бибкод : 2014Natur.512..244F . дои : 10.1038/512244a . ПМИД 25143097 .
- ^ Мак Э. (20 августа 2014 г.). «Жизнь подтверждена подо льдом Антарктики; следующий космос?» . Форбс . Проверено 21 августа 2014 г.
- ^ Виммер Э., Мюллер С., Тумпей Т.М., Таубенбергер Дж.К. (декабрь 2009 г.). «Синтетические вирусы: новая возможность понять и предотвратить вирусные заболевания» . Природная биотехнология . 27 (12): 1163–72. дои : 10.1038/nbt.1593 . ПМК 2819212 . ПМИД 20010599 .
- ^ Кунин Е.В., Сенкевич Т.Г., Доля В.В. (сентябрь 2006 г.). «Древний мир вирусов и эволюция клеток» . Биология Директ . 1:29 . дои : 10.1186/1745-6150-1-29 . ПМЦ 1594570 . ПМИД 16984643 .
- ^ Топли В.В., Уилсон Г.С., Коллиер Л.Х., Балоуз А., Сассман М. (1998). Махи Б.В., Кольер Л. (ред.). Микробиология и микробные инфекции Топли и Уилсона . Том. 1 (9-е изд.). Лондон: Арнольд. стр. 33–37. ISBN 978-0-340-66316-5 .
- ^ Айер Л.М., Баладжи С., Кунин Е.В., Аравинд Л. (апрель 2006 г.). «Эволюционная геномика ядерно-цитоплазматических крупных ДНК-вирусов» . Вирусные исследования . 117 (1): 156–84. doi : 10.1016/j.virusres.2006.01.009 . ПМИД 16494962 .
- ^ Санхуан Р., Небот М.Р., Кирико Н., Мански Л.М., Белшоу Р. (октябрь 2010 г.). «Темпы вирусных мутаций» . Журнал вирусологии . 84 (19): 9733–48. дои : 10.1128/JVI.00694-10 . ПМЦ 2937809 . ПМИД 20660197 .
- ^ Махи У.Дж., Ван Регенмортель М.Х., ред. (2009). Настольная энциклопедия общей вирусологии . Оксфорд: Академическая пресса. п. 28. ISBN 978-0-12-375146-1 .
- ^ Перейти обратно: а б Канчая С., Фурнус Г., Чибани-Шеннуфи С., Диллманн М.Л., Брюссов Х. (август 2003 г.). «Фаг как агент латерального переноса генов». Современное мнение в микробиологии . 6 (4): 417–24. дои : 10.1016/S1369-5274(03)00086-9 . ПМИД 12941415 .
- ^ Перейти обратно: а б Кунин Е.В., Старокадомский П. (октябрь 2016 г.). «Живы ли вирусы? Парадигма репликатора проливает решающий свет на старый, но ошибочный вопрос» . Исследования по истории и философии биологических и биомедицинских наук . 59 : 125–34. дои : 10.1016/j.shpsc.2016.02.016 . ПМК 5406846 . ПМИД 26965225 .
- ^ Рыбицкий Е.П. (1990). «Классификация организмов на пороге жизни, или Проблемы вирусной систематики». Южноафриканский научный журнал . 86 : 182–186.
- ^ Перейти обратно: а б Манн, Нью-Хэмпшир (май 2005 г.). «Третья эра фага» . ПЛОС Биология . 3 (5): е182. doi : 10.1371/journal.pbio.0030182 . ПМК 1110918 . ПМИД 15884981 .
- ^ Уоммак К.Е., Колвелл Р.Р. (март 2000 г.). «Вириопланктон: вирусы в водных экосистемах» . Обзоры микробиологии и молекулярной биологии . 64 (1): 69–114. дои : 10.1128/ММБР.64.1.69-114.2000 . ПМК 98987 . ПМИД 10704475 .
- ^ Перейти обратно: а б Саттл, Калифорния (сентябрь 2005 г.). «Вирусы в море». Природа . 437 (7057): 356–61. Бибкод : 2005Natur.437..356S . дои : 10.1038/nature04160 . ПМИД 16163346 . S2CID 4370363 .
- ^ Берг О., Борсхайм К.Ю., Братбак Г., Хелдал М. (август 1989 г.). «Высокое количество вирусов обнаружено в водной среде». Природа . 340 (6233): 467–8. Бибкод : 1989Natur.340..467B . дои : 10.1038/340467a0 . ПМИД 2755508 . S2CID 4271861 .
- ^ Вигингтон Ч., Зондереггер Д., Бруссаард К.П., Бьюкен А., Финке Дж.Ф., Фурман Дж.А. и др. (январь 2016 г.). «Повторное исследование взаимосвязи между численностью морских вирусов и микробных клеток» (PDF) . Природная микробиология . 1 (3): 15024. doi : 10.1038/nmicrobiol.2015.24 . ПМИД 27572161 . S2CID 52829633 .
- ^ Крупович М., Бэмфорд Д.Х. (июль 2007 г.). «Предположительные профаги, родственные литическому бесхвостому морскому фагу дцДНК PM2, широко распространены в геномах водных бактерий» . БМК Геномика . 8 : 236. дои : 10.1186/1471-2164-8-236 . ЧВК 1950889 . ПМИД 17634101 .
- ^ Сюэ Х, Сюй Ю, Буше Ю, Полц МФ (январь 2012 г.). «Высокая частота появления нового нитчатого фага VCY φ в окружающей популяции холерного вибриона» . Прикладная и экологическая микробиология . 78 (1): 28–33. Бибкод : 2012ApEnM..78...28X . дои : 10.1128/АЕМ.06297-11 . ПМЦ 3255608 . ПМИД 22020507 .
- ^ Ру С., Крупович М., Пуле А., Деброа Д., Эно Ф. (2012). «Эволюция и разнообразие вирусного семейства Microviridae посредством коллекции из 81 нового полного генома, собранного из считываний вирома» . ПЛОС ОДИН . 7 (7): е40418. Бибкод : 2012PLoSO...740418R . дои : 10.1371/journal.pone.0040418 . ПМЦ 3394797 . ПМИД 22808158 .
- ^ «Вредное цветение водорослей: Красный прилив: Домой |CDC HSB» . www.cdc.gov . Проверено 19 декабря 2014 г.
- ^ Лоуренс С.М., Менон С., Эйлерс Б.Дж., Ботнер Б., Хаят Р., Дуглас Т., Янг М.Дж. (май 2009 г.). «Структурно-функциональные исследования архейных вирусов» . Журнал биологической химии . 284 (19): 12599–603. дои : 10.1074/jbc.R800078200 . ПМК 2675988 . ПМИД 19158076 .
- ^ Прангишвили Д., Фортер П., Гаррет Р.А. (ноябрь 2006 г.). «Вирусы архей: объединяющий взгляд». Обзоры природы. Микробиология . 4 (11): 837–48. дои : 10.1038/nrmicro1527 . ПМИД 17041631 . S2CID 9915859 .
- ^ Прангишвили Д., Гаррет Р.А. (апрель 2004 г.). «Исключительно разнообразные морфотипы и геномы кренархейных гипертермофильных вирусов» (PDF) . Труды Биохимического общества . 32 (Часть 2): 204–8. дои : 10.1042/BST0320204 . ПМИД 15046572 . S2CID 20018642 .
- ^ Фортер П., Филипп Х (июнь 1999 г.). «Последний универсальный общий предок (LUCA), простой или сложный?». Биологический вестник . 196 (3): 373–5, обсуждение 375–7. дои : 10.2307/1542973 . JSTOR 1542973 . ПМИД 11536914 .
- ^ Фредриксон Дж.К., Захара Дж.М., Балквилл Д.Л., Кеннеди Д., Ли С.М., Костандаритес Х.М. и др. (июль 2004 г.). «Геомикробиология вадозных отложений, сильно загрязненных ядерными отходами, на полигоне в Хэнфорде, штат Вашингтон» . Прикладная и экологическая микробиология . 70 (7): 4230–41. Бибкод : 2004ApEnM..70.4230F . дои : 10.1128/АЕМ.70.7.4230-4241.2004 . ПМК 444790 . ПМИД 15240306 .
- ^ Вёзе Ч.Р., Кандлер О., Уилис М.Л. (июнь 1990 г.). «На пути к естественной системе организмов: предложения по доменам архей, бактерий и эукариев» . Труды Национальной академии наук Соединенных Штатов Америки . 87 (12): 4576–9. Бибкод : 1990PNAS...87.4576W . дои : 10.1073/pnas.87.12.4576 . ПМК 54159 . ПМИД 2112744 .
- ^ Делонг Э.Ф., Пейс Н.Р. (август 2001 г.). «Экологическое разнообразие бактерий и архей». Систематическая биология . 50 (4): 470–8. CiteSeerX 10.1.1.321.8828 . дои : 10.1080/106351501750435040 . ПМИД 12116647 .
- ^ Браун-младший, Дулиттл В.Ф. (декабрь 1997 г.). «Археи и переход от прокариот к эукариотам» . Обзоры микробиологии и молекулярной биологии . 61 (4): 456–502. doi : 10.1128/mmbr.61.4.456-502.1997 . ПМК 232621 . ПМИД 9409149 .
- ^ Ланг Б.Ф., Грей М.В., Бургер Дж. (1999). «Эволюция митохондриального генома и происхождение эукариот». Ежегодный обзор генетики . 33 : 351–97. дои : 10.1146/annurev.genet.33.1.351 . ПМИД 10690412 .
- ^ Макфадден Дж.И. (декабрь 1999 г.). «Эндосимбиоз и эволюция растительной клетки». Современное мнение в области биологии растений . 2 (6): 513–9. дои : 10.1016/S1369-5266(99)00025-4 . ПМИД 10607659 .
- ^ Килинг П.Дж. (2004). «Разнообразие и история эволюции пластид и их хозяев». Американский журнал ботаники . 91 (10): 1481–1493. дои : 10.3732/ajb.91.10.1481 . ПМИД 21652304 . S2CID 17522125 .
- ^ «Самая большая бактерия: ученый обнаруживает новую бактериальную форму жизни у африканского побережья» , Институт морской микробиологии Макса Планка , 8 апреля 1999 г., заархивировано из оригинала 20 января 2010 г.
- ^ Список названий прокариот, имеющих место в номенклатуре - Род Thiomargarita
- ^ Банг С., Шмитц Р.А. (сентябрь 2015 г.). «Археи, связанные с человеческими поверхностями: не следует недооценивать» . Обзоры микробиологии FEMS . 39 (5): 631–48. дои : 10.1093/femsre/fuv010 . ПМИД 25907112 .
- ^ «Архея» . Интернет-словарь этимологии . Проверено 17 августа 2016 г.
- ^ Пейс НР (май 2006 г.). «Время перемен» . Природа . 441 (7091): 289. Бибкод : 2006Natur.441..289P . дои : 10.1038/441289a . ПМИД 16710401 . S2CID 4431143 .
- ^ Стоекениус В. (октябрь 1981 г.). «Квадратная бактерия Уолсби: тонкая структура ортогонального прокариота» . Журнал бактериологии . 148 (1): 352–60. дои : 10.1128/JB.148.1.352-360.1981 . ПМК 216199 . ПМИД 7287626 .
- ^ Уиттакер Р.Х., Маргулис Л. (апрель 1978 г.). «Классификация протистов и царства организмов». Биосистемы . 10 (1–2): 3–18. дои : 10.1016/0303-2647(78)90023-0 . ПМИД 418827 .
- ^ Фор Э, Нот Ф, Бенуастон А.С., Лабади К., Биттнер Л., Аята С.Д. (апрель 2019 г.). «Миксотрофные протисты демонстрируют контрастную биогеографию в мировом океане» . Журнал ISME . 13 (4): 1072–1083. дои : 10.1038/s41396-018-0340-5 . ПМК 6461780 . ПМИД 30643201 .
- ^ Перейти обратно: а б Лелес С.Г., Митра А., Флинн К.Дж., Стокер Д.К., Хансен П.Дж., Калбет А. и др. (август 2017 г.). «Океанические протисты с различными формами приобретенной фототрофии демонстрируют контрастную биогеографию и численность» . Слушания. Биологические науки . 284 (1860): 20170664. doi : 10.1098/rspb.2017.0664 . ПМЦ 5563798 . ПМИД 28768886 .
- ^ Бадд Дж.Э., Дженсен С. (февраль 2017 г.). «Происхождение животных и гипотеза «саванны» ранней двусторонней эволюции» . Биологические обзоры Кембриджского философского общества . 92 (1): 446–473. дои : 10.1111/brv.12239 . ПМИД 26588818 .
- ^ Кавалер-Смит Т. (декабрь 1993 г.). «Царство простейших и его 18 типов» . Микробиологические обзоры . 57 (4): 953–94. дои : 10.1128/MMBR.57.4.953-994.1993 . ПМК 372943 . ПМИД 8302218 .
- ^ Корлисс Дж.О. (1992). «Должен ли быть отдельный кодекс номенклатуры для протистов?». Биосистемы . 28 (1–3): 1–14. дои : 10.1016/0303-2647(92)90003-H . ПМИД 1292654 .
- ^ Слапета Х., Морейра Д., Лопес-Гарсия П. (октябрь 2005 г.). «Степень разнообразия протистов: выводы из молекулярной экологии пресноводных эукариот» . Слушания. Биологические науки . 272 (1576): 2073–81. дои : 10.1098/rspb.2005.3195 . ПМЦ 1559898 . ПМИД 16191619 .
- ^ Морейра Д., Лопес-Гарсия П. (январь 2002 г.). «Молекулярная экология микробных эукариот открывает скрытый мир». Тенденции в микробиологии . 10 (1): 31–8. дои : 10.1016/S0966-842X(01)02257-0 . ПМИД 11755083 .
- ^ Перейти обратно: а б Алверсон А. (11 июня 2014 г.). «Воздух, которым вы дышите? Это сделала диатомовая водоросль» . Живая наука .
- ^ «Подробнее о диатомовых водорослях» . Музей палеонтологии Калифорнийского университета . Архивировано из оригинала 4 октября 2012 года . Проверено 27 июня 2019 г.
- ^ Девреотес П. (сентябрь 1989 г.). «Dictyostelium discoideum: модельная система межклеточных взаимодействий в развитии». Наука . 245 (4922): 1054–8. Бибкод : 1989Sci...245.1054D . дои : 10.1126/science.2672337 . ПМИД 2672337 .
- ^ Мац М.В., Фрэнк Т.М., Маршалл Н.Дж., Виддер Э.А., Джонсен С. (декабрь 2008 г.). «Гигантский глубоководный протист оставляет следы, похожие на двусторонние». Современная биология . 18 (23). Elsevier Ltd: 1849–54. дои : 10.1016/j.cub.2008.10.028 . ПМИД 19026540 . S2CID 8819675 .
- ^ Гудэй А.Дж., Да Силва А.А., Павловски Дж. (1 декабря 2011 г.). «Ксенофиофоры (Rhizaria, Foraminifera) из каньона Назаре (португальская окраина, северо-восток Атлантики)». Глубоководные исследования. Часть II: Актуальные исследования в океанографии . Геология, геохимия и биология подводных каньонов к западу от Португалии. 58 (23–24): 2401–2419. Бибкод : 2011DSRII..58.2401G . дои : 10.1016/j.dsr2.2011.04.005 .
- ^ Нил А.С., Рис Дж.Б., Саймон Э.Дж. (2004). Основная биология с физиологией . Пирсон/Бенджамин Каммингс. п. 291. ИСБН 978-0-8053-7503-9 .
- ^ О'Мэлли М.А., Симпсон А.Г., Роджер А.Дж. (2012). «Другие эукариоты в свете эволюционной протистологии». Биология и философия . 28 (2): 299–330. дои : 10.1007/s10539-012-9354-y . S2CID 85406712 .
- ^ Адл С.М., Симпсон А.Г., Фармер М.А., Андерсен Р.А., Андерсон О.Р., Барта Дж.Р. и др. (2005). «Новая классификация эукариот более высокого уровня с упором на таксономию простейших» . Журнал эукариотической микробиологии . 52 (5): 399–451. дои : 10.1111/j.1550-7408.2005.00053.x . ПМИД 16248873 . S2CID 8060916 .
- ^ Маргулис Л., Чепмен М.Дж. (19 марта 2009 г.). Королевства и владения: Иллюстрированный путеводитель по типам жизни на Земле . Академическая пресса. ISBN 9780080920146 .
- ^ Фанг Дж (апрель 2010 г.). «Животные процветают без кислорода на морском дне» . Природа . 464 (7290): 825. Бибкод : 2010Natur.464..825F . дои : 10.1038/464825b . ПМИД 20376121 .
- ^ «Глубокий соленый бассейн может быть домом для животных, живущих без кислорода» . Новости науки . 23 сентября 2013 г.
- ^ Йонасдоттир, Сигрун Хульд; Виссер, Андре В.; Ричардсон, Кэтрин; Хит, Майкл Р. (29 сентября 2015 г.). «Сезонный липидный насос веслоногих ракообразных способствует секвестрации углерода в глубинах Северной Атлантики» . Труды Национальной академии наук . 112 (39): 12122–12126. дои : 10.1073/pnas.1512110112 . ISSN 0027-8424 . ПМЦ 4593097 . ПМИД 26338976 .
- ^ Пинти, Жером; Йонасдоттир, Сигрун Х.; Запись, Николас Р.; Виссер, Андре В. (7 марта 2023 г.). «Глобальный вклад сезонно мигрирующих копепод в биологический углеродный насос» . Лимнология и океанография . 68 (5): 1147–1160. Бибкод : 2023LimOc..68.1147P . дои : 10.1002/lno.12335 . ISSN 0024-3590 . S2CID 257422956 .
- ^ Пепато, Альмир Р.; Видигал, Теофания HDA; Климов, Павел Б. (2018). «Молекулярная филогения морских клещей (Acariformes: Halacaridae), древнейшей разновидности современных вторично морских животных» . Молекулярная филогенетика и эволюция . 129 : 182–188. дои : 10.1016/j.ympev.2018.08.012 . ПМИД 30172010 . S2CID 52145427 .
- ^ Хайд К.Д., Джонс Э.Г., Леаньо Э., Пойнтинг С.Б., Пунит А.Д., Вреймоед Л.Л. (1998). «Роль грибов в морских экосистемах». Биоразнообразие и сохранение . 7 (9): 1147–1161. дои : 10.1023/A:1008823515157 . S2CID 22264931 .
- ^ Кирк П.М., Кэннон П.Ф., Минтер Д.В., Сталперс Дж. (2008). Словарь грибов (10-е изд.). КАБИ.
- ^ Хайд К.Д., Гринвуд Р., Джонс Э.Г. (1989). «Прикрепление спор у морских грибов». Ботаника Марина . 32 (3): 205–218. дои : 10.1515/ботм.1989.32.3.205 . S2CID 84879817 .
- ^ Ле Кальвес Т., Бурго Г., Маэ С., Барбье Г., Ванденкокорнхейс П. (октябрь 2009 г.). «Грибное разнообразие в глубоководных гидротермальных экосистемах» . Прикладная и экологическая микробиология . 75 (20): 6415–21. Бибкод : 2009ApEnM..75.6415L . дои : 10.1128/АЕМ.00653-09 . ПМЦ 2765129 . ПМИД 19633124 .
- ^ Сан-Мартин А., Оредхарена С., Галлардо С., Сильва М., Бесерра Дж., Рейносо Р.О., Чами М.К., Вергара К., Ровироса Дж. (2008). «Стероиды морского гриба Geotrichum sp» . Журнал Чилийского химического общества . 53 (1): 1377–1378. дои : 10.4067/S0717-97072008000100011 .
- ^ Джонс Э.Б., Хайд К.Д., Панг К.Л., ред. (2014). Пресноводные грибы: и грибоподобные организмы . Берлин/Бостон: Де Грюйтер. ISBN 9783110333480 .
- ^ Джонс Э.Б., Панг К.Л., ред. (2012). Морские грибы и грибоподобные организмы . Морская и пресноводная ботаника. Берлин, Бостон: Де Грюйтер (опубликовано в августе 2012 г.). дои : 10.1515/9783110264067 . ISBN 978-3-11-026406-7 . Проверено 3 сентября 2015 г.
- ^ Ван X, Сингх П., Гао Z, Чжан X, Джонсон ZI, Ван G (2014). «Распространение и разнообразие планктонных грибов в теплом бассейне западной части Тихого океана» . ПЛОС ОДИН . 9 (7): e101523. Бибкод : 2014PLoSO...9j1523W . дои : 10.1371/journal.pone.0101523.s001 . ПМЦ 4081592 . ПМИД 24992154 .
- ^ Ван Г, Ван Х, Лю Х, Ли Ц (2012). «Разнообразие и биогеохимическая функция планктонных грибов океана» . В Рагукумаре С. (ред.). Биология морских грибов . Прогресс молекулярной и субклеточной биологии. Том. 53. Берлин, Гейдельберг: Springer-Verlag. стр. 71–88. дои : 10.1007/978-3-642-23342-5 . ISBN 978-3-642-23341-8 . S2CID 39378040 . Проверено 3 сентября 2015 г.
- ^ Дамаре С., Рагукумар С. (июль 2008 г.). «Грибы и макроагрегация в глубоководных отложениях». Микробная экология . 56 (1): 168–77. дои : 10.1007/s00248-007-9334-y . ПМИД 17994287 . S2CID 21288251 .
- ^ Кубанек Дж., Дженсен П.Р., Кейфер П.А., Саллардс М.К., Коллинз Д.О., Феникал В. (июнь 2003 г.). «Устойчивость морских водорослей к микробному воздействию: целенаправленная химическая защита от морских грибов» . Труды Национальной академии наук Соединенных Штатов Америки . 100 (12): 6916–21. Бибкод : 2003PNAS..100.6916K . дои : 10.1073/pnas.1131855100 . ПМК 165804 . ПМИД 12756301 .
- ^ Перейти обратно: а б Гао З., Джонсон З.И., Ван Дж. (январь 2010 г.). «Молекулярная характеристика пространственного разнообразия и новых линий микопланктона в прибрежных водах Гавайских островов» . Журнал ISME . 4 (1): 111–20. дои : 10.1038/ismej.2009.87 . ПМИД 19641535 .
- ^ Панцер К., Йилмаз П., Вайс М., Райх Л., Рихтер М., Визе Дж. и др. (30 июля 2015 г.). «Идентификация биомов водных грибковых сообществ, специфичных для среды обитания, с использованием комплексного почти полноразмерного набора данных 18S рРНК, обогащенного контекстными данными» . ПЛОС ОДИН . 10 (7): e0134377. Бибкод : 2015PLoSO..1034377P . дои : 10.1371/journal.pone.0134377 . ПМК 4520555 . ПМИД 26226014 .
- ^ Гутьеррес М.Х., Пантоха С., Хинонес Р.А., Гонсалес Р.Р. (2010). «Первая запись нитчатых грибов в прибрежной экосистеме апвеллинга у центрального Чили». Гаяна (на испанском языке). 74 (1): 66–73.
- ^ Перейти обратно: а б Шридхар КР (2009). «10. Водные грибы – они планктонные?». Планктонная динамика вод Индии . Джайпур, Индия: Публикации Пратикши. стр. 133–148.
- ^ «Виды высших морских грибов» . Университет Миссисипи. Архивировано из оригинала 22 апреля 2013 года . Проверено 5 февраля 2012 г.
- ^ Хоксворт Д.Л. (2000). «Пресноводные и морские лишайниковообразующие грибы» (PDF) . Грибное разнообразие . 5 : 1–7.
- ^ «Лишайники» . Служба национальных парков, Министерство внутренних дел США, Правительство США. 22 мая 2016 года . Проверено 4 апреля 2018 г.
- ^ Перейти обратно: а б «Сеть земной жизни, рост и развитие лишайников» . Earthlife.net. 14 февраля 2020 г.
- ^ Силлиман Б.Р., Ньюэлл С.Ю. (декабрь 2003 г.). «Грибное хозяйство улитки» . Труды Национальной академии наук Соединенных Штатов Америки . 100 (26): 15643–8. Бибкод : 2003PNAS..10015643S . дои : 10.1073/pnas.2535227100 . ПМК 307621 . ПМИД 14657360 .
- ^ Юань X, Сяо С., Тейлор Т.Н. (май 2005 г.). «Лишайноподобный симбиоз 600 миллионов лет назад». Наука . 308 (5724): 1017–20. Бибкод : 2005Sci...308.1017Y . дои : 10.1126/science.1111347 . ПМИД 15890881 . S2CID 27083645 .
- ^ Джонс Э.Б., Панг К.Л. (31 августа 2012 г.). Морские грибы: и грибоподобные организмы . Вальтер де Грюйтер. ISBN 9783110264067 .
- ^ Дэвидсон М.В. (26 мая 2005 г.). «Структура животных клеток» . Молекулярные выражения . Таллахасси, Флорида: Университет штата Флорида . Проверено 3 сентября 2008 г.
- ^ Фогель Г. (20 сентября 2018 г.). «Это ископаемое является одним из самых ранних животных в мире, если судить по молекулам жира, сохранившимся в течение полумиллиарда лет» . Наука . АААС . Проверено 21 сентября 2018 г.
- ^ Бобровский И., Хоуп Дж.М., Иванцов А., Неттерсхайм Б.Дж., Холлманн С., Брокс Дж.Дж. (сентябрь 2018 г.). «Древние стероиды делают эдиакарскую ископаемую дикинсонию одним из самых ранних животных» . Наука . 361 (6408): 1246–1249. Бибкод : 2018Sci...361.1246B . дои : 10.1126/science.aat7228 . hdl : 1885/230014 . ПМИД 30237355 .
- ^ Реталлак Г.Дж. (2007). «Рост, распад и уплотнение захоронений дикинсонии, культовой окаменелости Эдиакарского региона» (PDF) . Алчеринга: Австралазийский журнал палеонтологии . 31 (3): 215–240. Бибкод : 2007Алч...31..215Р . дои : 10.1080/03115510701484705 . S2CID 17181699 .
- ^ Сперлинг Э.А., Винтер Дж (2010). «Сродство плакозой к дикинсонии и эволюция способов питания многоклеточных животных позднего протерозоя». Эволюция и развитие . 12 (2): 201–9. дои : 10.1111/j.1525-142X.2010.00404.x . ПМИД 20433459 . S2CID 38559058 .
- ^ Голд Д.А., Раннегар Б., Гелинг Дж.Г., Джейкобс Д.К. (2015). «Реконструкция онтогенеза наследственного состояния подтверждает двустороннюю близость к дикинсонии». Эволюция и развитие . 17 (6): 315–24. дои : 10.1111/ede.12168 . ПМИД 26492825 . S2CID 26099557 .
- ^ Чен Дж.Ю., Оливери П., Гао Ф., Дорнбос С.К., Ли К.В., Боттьер DJ, Дэвидсон Э.Х. (август 2002 г.). «Животный мир докембрия: вероятные формы развития и взрослые книдарии из Юго-Западного Китая» (PDF) . Биология развития . 248 (1): 182–96. дои : 10.1006/dbio.2002.0714 . ПМИД 12142030 . Архивировано из оригинала (PDF) 26 мая 2013 года . Проверено 4 февраля 2015 г.
- ^ Гражданкин Д (июнь 2004 г.). «Схемы распространения в эдиакарской биоте: фации против биогеографии и эволюции». Палеобиология . 30 (2): 203–221. doi : 10.1666/0094-8373(2004)030<0203:PODITE>2.0.CO;2 . ISSN 0094-8373 . S2CID 129376371 .
- ^ Сейлахер А (август 1992 г.). «Вендобионта и Псаммокораллия: утраченные конструкции докембрийской эволюции» . Журнал Геологического общества . 149 (4): 607–613. Бибкод : 1992JGSoc.149..607S . дои : 10.1144/gsjgs.149.4.0607 . ISSN 0016-7649 . S2CID 128681462 . Проверено 4 февраля 2015 г.
- ^ Мартин М.В., Гражданкин Д.В., Боуринг С.А., Эванс Д.А., Федонкин М.А., Киршвинк Дж.Л. (май 2000 г.). «Возраст неопротерозойского тела двулатарий и следы окаменелостей, Белое море, Россия: значение для эволюции многоклеточных животных». Наука . 288 (5467): 841–5. Бибкод : 2000Sci...288..841M . дои : 10.1126/science.288.5467.841 . ПМИД 10797002 . S2CID 1019572 .
- ^ Федонкин М.А., Вагонер Б.М. (28 августа 1997 г.). позднего докембрия «Ископаемая кимберелла представляет собой двусторонний организм, похожий на моллюска» . Природа . 388 (6645): 868–871. Бибкод : 1997Natur.388..868F . дои : 10.1038/42242 . ISSN 0028-0836 . S2CID 4395089 .
- ^ Муи Р., Дэвид Б. (декабрь 1998 г.). «Эволюция внутри причудливого типа: гомологии первых иглокожих» . Американский зоолог . 38 (6): 965–974. дои : 10.1093/icb/38.6.965 . ISSN 1540-7063 .
- ^ Макменамин М.А. (сентябрь 2003 г.). Сприггина — трилобитоидное экдизозойное животное . Геонаучные горизонты, Сиэтл, 2003 . Рефераты с программами. Том. 35. Боулдер, Колорадо: Геологическое общество Америки. п. 105. OCLC 249088612 . Архивировано из оригинала 12 апреля 2016 года . Проверено 24 ноября 2007 г. Документ № 40-2, представленный на ежегодном собрании Геологического общества Америки в Сиэтле 2003 г. (2–5 ноября 2003 г.) 2 ноября 2003 г. в Конференц-центре штата Вашингтон .
- ^ Линь Дж.П., Гон III С.М., Гелинг Дж.Г., Бэбкок Л.Е., Чжао Ю.Л., Чжан С.Л. и др. (2006). « Парванкориноподобное членистоногое из кембрия Южного Китая». Историческая биология: Международный журнал палеобиологии . 18 (1): 33–45. дои : 10.1080/08912960500508689 . ISSN 1029-2381 . S2CID 85821717 .
- ^ Баттерфилд, штат Нью-Джерси (декабрь 2006 г.). «Зацепка некоторых «червей» стволовой группы: ископаемые лофотрохозойи в сланцах Берджесс». Биоэссе . 28 (12): 1161–6. doi : 10.1002/bies.20507 . ПМИД 17120226 . S2CID 29130876 .
- ^ Перейти обратно: а б Бенгтсон С. (ноябрь 2004 г.). «Ранние скелетные окаменелости». Документы Палеонтологического общества . 10 : 67–78. дои : 10.1017/S1089332600002345 .
- ^ Валентин Дж.В. (2004). О происхождении Фила . Чикаго: Издательство Чикагского университета. п. 7. ISBN 978-0-226-84548-7 .
Классификации организмов в иерархических системах использовались в семнадцатом и восемнадцатом веках. Обычно организмы группировались по их морфологическому сходству, как это воспринималось теми ранними исследователями, а затем эти группы группировались по их сходству и так далее, чтобы сформировать иерархию.
- ^ Перейти обратно: а б Валентин JW (18 июня 2004 г.). О происхождении Фила . Издательство Чикагского университета. ISBN 9780226845487 .
- ^ «WoRMS — Всемирный реестр морских видов» . www.marinespecies.org .
- ^ Новак Б.Дж., Фрейзер Д., Мэлони Т.Х. (февраль 2020 г.). «Преобразование охраны океана: применение набора инструментов генетического спасения» . Гены . 11 (2): 209. doi : 10.3390/genes11020209 . ПМК 7074136 . ПМИД 32085502 .
- ^ Гулд С.Дж. (1990). Прекрасная жизнь: сланцы Бёрджесс и природа истории . WW Нортон. ISBN 978-0-393-30700-9 .
- ^ Эрвин Д., Валентайн Дж., Яблонски Д. (1997). «Недавние находки окаменелостей и новое понимание развития животных открывают новый взгляд на загадку массового расцвета животных в раннем кембрии» . Американский ученый (март – апрель).
- ^ Перейти обратно: а б Бадд Дж.Э., Дженсен С. (май 2000 г.). «Критическая переоценка летописи окаменелостей двусторонних типов». Биологические обзоры Кембриджского философского общества . 75 (2): 253–95. дои : 10.1111/j.1469-185X.1999.tb00046.x . ПМИД 10881389 . S2CID 39772232 .
- ^ Гулд С.Дж. (1989). Прекрасная жизнь: сланцы Берджесс и природа истории (Первое изд.). Нью-Йорк. ISBN 978-0-393-02705-1 .
{{cite book}}
: CS1 maint: отсутствует местоположение издателя ( ссылка ) - ^ Бадд Дж. Э. (февраль 2003 г.). «Кембрийская летопись окаменелостей и происхождение типов» . Интегративная и сравнительная биология . 43 (1): 157–65. дои : 10.1093/icb/43.1.157 . ПМИД 21680420 .
- ^ Бадд Дж. Э. (март 1996 г.). «Морфология Opabinia regalis и реконструкция стеблевой группы членистоногих». Летайя . 29 (1): 1–14. дои : 10.1111/j.1502-3931.1996.tb01831.x . ISSN 0024-1164 .
- ^ Маршалл CR (май 2006 г.). «Объяснение кембрийского «взрыва» животных». Ежегодный обзор наук о Земле и планетах . 34 : 355–384. Бибкод : 2006AREPS..34..355M . doi : 10.1146/annurev.earth.33.031504.103001 . ISSN 1545-4495 . S2CID 85623607 .
- ^ Кинг Н., Рокас А. (октябрь 2017 г.). «Принятие неопределенности в реконструкции ранней эволюции животных» . Современная биология . 27 (19): Р1081–Р1088. дои : 10.1016/j.cub.2017.08.054 . ПМК 5679448 . ПМИД 29017048 .
- ^ Феуда Р., Дорманн М., Петт В., Филипп Х., Рота-Стабелли О., Лартильо Н. и др. (декабрь 2017 г.). «Улучшенное моделирование композиционной неоднородности подтверждает, что губки являются сестрами всех других животных» . Современная биология . 27 (24): 3864–3870.e4. дои : 10.1016/j.cub.2017.11.008 . hdl : 11572/302898 . ПМИД 29199080 .
- ^ Нильсен С (июль 2019 г.). «Ранняя эволюция животных: взгляд морфолога» . Королевское общество открытой науки . 6 (7): 190638. Бибкод : 2019RSOS....690638N . дои : 10.1098/rsos.190638 . ПМК 6689584 . ПМИД 31417759 .
- ^ «Порифера (н.)» . Интернет-словарь этимологии . Проверено 18 августа 2016 г.
- ^ Перейти обратно: а б Петралия Р.С., Мэттсон, член парламента, Яо П.Дж. (июль 2014 г.). «Старение и долголетие у простейших животных и поиски бессмертия» . Обзоры исследований старения . 16 :66–82. дои : 10.1016/J.arr.2014.05.003 . ПМЦ 4133289 . ПМИД 24910306 .
- ^ Йохум КП, Ван Х, Веннеманн Т.В., Синха Б., Мюллер В.Е. (2012). «Кремневая глубоководная губка Monorhaphis chuni : потенциальный архив палеоклимата древних животных». Химическая геология . 300 : 143–151. Бибкод : 2012ЧГео.300..143J . doi : 10.1016/j.chemgeo.2012.01.009 .
- ^ Васелет Дж., Дюпор Э. (ноябрь 2004 г.). «Захват и переваривание добычи у плотоядной губки Asbestopluma hypogea (Porifera: Demospongiae)». Зооморфология . 123 (4): 179–90. дои : 10.1007/s00435-004-0100-0 . S2CID 24484610 .
- ^ « Губка Линней , 1759 год» . Всемирный регистр морских видов . Проверено 18 июля 2012 г.
- ^ Роуленд С.М., Стивенс Т. (2001). «Археоциаты: история филогенетической интерпретации». Журнал палеонтологии . 75 (6): 1065–1078. doi : 10.1666/0022-3360(2001)075<1065:AAHOPI>2.0.CO;2 . JSTOR 1307076 . S2CID 86211946 .
- ^ Сперлинг Э.А., Пизани Д., Петерсон К.Дж. (1 января 2007 г.). «Пориферановая парафилия и ее значение для палеобиологии докембрия» (PDF) . Геологическое общество, Лондон, специальные публикации . 286 (1): 355–368. Бибкод : 2007GSLSP.286..355S . дои : 10.1144/SP286.25 . S2CID 34175521 . Архивировано из оригинала (PDF) 9 мая 2009 года . Проверено 22 августа 2012 г.
- ^ Руперт Э.Э., Фокс Р.С., Барнс Р.Д. (2004). Зоология беспозвоночных (7-е изд.). Брукс / Коул. стр. 182–195 . ISBN 978-0-03-025982-1 .
- ^ Миллс CE. «Гребневики – некоторые заметки эксперта» . Проверено 5 февраля 2009 г.
- ^ Перейти обратно: а б Бруска Р.К., Бруска Г.Дж. (2003). Беспозвоночные (Второе изд.). Синауэр Ассошиэйтс. ISBN 978-0-87893-097-5 .
- ^ Ле Пейдж М (март 2019 г.). «Животное с анусом, который приходит и уходит, может показать, как развивался наш анус» . Новый учёный .
- ^ Мартиндейл MQ, Финнерти-младший, Генри JQ (сентябрь 2002 г.). «Ладиаты и эволюционное происхождение двустороннего строения тела». Молекулярная филогенетика и эволюция . 24 (3): 358–65. дои : 10.1016/s1055-7903(02)00208-7 . ПМИД 12220977 .
- ^ Placozoa в Национальной медицинской библиотеке США по медицинским предметным рубрикам (MeSH)
- ^ Венер Р., Геринг В. (июнь 2007 г.). Зоология (на немецком языке) (24-е изд.). Штутгарт: Тиме. п. 696.
- ^ Шульце Ф.Э. (1883). « Трихоплакс прилипший , нс». Зоологический вестник . Том 6. Амстердам и Йена: Эльзевир. п. 92.
- ^ Эйтель М., Фрэнсис В.Р., Осигус Х.Дж., Кребс С., Варгас С., Блюм Х. и др. (13 октября 2017 г.). «Таксогеномный подход открывает новый род в типе Placozoa» . bioRxiv : 202119. doi : 10.1101/202119 . S2CID 89829846 .
- ^ Осигус Х.Дж., Рольфес С., Херцог Р., Камм К., Шируотер Б. (март 2019 г.). «Polyplacotoma mediterranea — новый разветвленный вид плакозойных» . Современная биология . 29 (5): Р148–Р149. дои : 10.1016/j.cub.2019.01.068 . ПМИД 30836080 .
- ^ «Прилипание трихоплакса» . ВОРМС . 2009 год
- ^ Смит К.Л., Варокко Ф., Киттельманн М., Аззам Р.Н., Купер Б., Уинтерс К.А. и др. (июль 2014 г.). «Новые типы клеток, нейросекреторные клетки и строение тела ранних дивергентных многоклеточных животных Trichoplax adhaerens» . Современная биология . 24 (14): 1565–1572. дои : 10.1016/j.cub.2014.05.046 . ПМЦ 4128346 . ПМИД 24954051 .
- ^ Барнс Р.Д. (1982). Зоология беспозвоночных . Филадельфия: Холт-Сондерс Интернэшнл. стр. 84–85. ISBN 978-0-03-056747-6 .
- ^ Чжан ZQ (2011). «Биоразнообразие животных: введение в классификацию более высокого уровня и таксономическое богатство» (PDF) . Зоотакса . 3148 : 7–12. дои : 10.11646/zootaxa.3148.1.3 .
- ^ « Нематостелла vectensis v1.0» . Геномный портал . Калифорнийский университет . Проверено 19 января 2014 г.
- ^ « Нематостелла » . Nematostella.org. Архивировано из оригинала 8 мая 2006 года . Проверено 18 января 2014 г.
- ^ Перейти обратно: а б Генихович Г, Технау У (сентябрь 2009 г.). «Старлетка актиния Nematostella vectensis: модельный организм антозоев для исследований в области сравнительной геномики и функциональной эволюционной биологии развития». Протоколы Колд-Спринг-Харбора . 2009 (9): pdb.emo129. дои : 10.1101/pdb.emo129 . ПМИД 20147257 .
- ^ «Откуда берется наша голова? Безмозглая актиния проливает новый свет на эволюционное происхождение головы» . Наука Дейли . 12 февраля 2013 года . Проверено 18 января 2014 г.
- ^ Синигалья К., Бусенгдал Х., Леклер Л., Технау У., Ренцш Ф. (2013). «Ген формирования билатерального паттерна головы шесть3/6 контролирует развитие аборального домена у книдарий» . ПЛОС Биология . 11 (2): e1001488. дои : 10.1371/journal.pbio.1001488 . ПМЦ 3586664 . ПМИД 23483856 .
- ^ «Медуза-красный бумажный фонарь» . Настоящие чудовища . Проверено 25 октября 2015 г.
- ^ «Голубые кнопки во Флориде» . BeachHunter.net .
- ^ Карлескинт Дж., Тернер Р., Смолл Дж. (2012). Введение в морскую биологию (4-е изд.). Cengage Обучение. п. 445. ИСБН 978-1-133-36446-7 .
- ^ Бавестрелло Дж., Соммер С., Сара М. (1992). «Двунаправленное преобразование у Turritopsis nutricula (Hydrozoa)». Морская наука . 56 (2–3): 137–140.
- ^ Пираино С., Боэро Ф., Эшбах Б., Шмид В. (1996). «Обращение жизненного цикла: медузы превращаются в полипы и трансдифференцировка клеток у Turritopsis nutricula (Cnidaria, Hydrozoa)». Биологический бюллетень . 190 (3): 302–312. дои : 10.2307/1543022 . JSTOR 1543022 . ПМИД 29227703 . S2CID 3956265 .
- ^ Феннер П.Дж., Уильямсон Дж.А. (1996). «Смерть во всем мире и тяжелое отравление от укусов медуз». Медицинский журнал Австралии . 165 (11–12): 658–61. дои : 10.5694/j.1326-5377.1996.tb138679.x . ПМИД 8985452 . S2CID 45032896 .
- ^ Перейти обратно: а б с Кэннон Дж.Т., Веллутини Б.С., Смит Дж., Ронквист Ф., Джонделиус Ю., Хейнол А. (февраль 2016 г.). «Xenacoelomorpha — сестринская группа Nephrozoa» . Природа . 530 (7588): 89–93. Бибкод : 2016Natur.530...89C . дои : 10.1038/nature16520 . ПМИД 26842059 . S2CID 205247296 .
- ^ Перейти обратно: а б Минелли А (2009). Перспективы филогении и эволюции животных . Издательство Оксфордского университета. п. 53. ИСБН 978-0-19-856620-5 .
- ^ Перейти обратно: а б с Бруска RC (2016). Введение в Bilateria и тип Xenacoelomorpha | Триплобластия и двусторонняя симметрия открывают новые возможности для облучения животных (PDF) . Синауэр Ассошиэйтс. стр. 345–372. ISBN 978-1605353753 .
{{cite book}}
:|work=
игнорируется ( помогите ) - ^ Финнерти-младший (ноябрь 2005 г.). «Способствовал ли внутренний транспорт, а не направленное передвижение, развитию двусторонней симметрии у животных?» (PDF) . Биоэссе . 27 (11): 1174–80. дои : 10.1002/bies.20299 . ПМИД 16237677 . Архивировано из оригинала (PDF) 10 августа 2014 года . Проверено 27 августа 2019 г.
- ^ Куиллин К.Дж. (май 1998 г.). «Онтогенетическое масштабирование гидростатических скелетов: масштабирование геометрического, статического и динамического стресса дождевого червя lumbricus terrestris» . Журнал экспериментальной биологии . 201 (12): 1871–83. дои : 10.1242/jeb.201.12.1871 . ПМИД 9600869 .
- ^ Спектор Б (26 марта 2020 г.). «Этот первобытный червь может быть предком всех животных]» . Живая наука» .
- ^ Перейти обратно: а б Уэйд Н. (30 января 2017 г.). «Этот доисторический предок человека был весь рот» . Нью-Йорк Таймс . Проверено 31 января 2017 г.
- ^ Перейти обратно: а б Хан Дж., Моррис СК, Оу Ц, Шу Д., Хуан Х. (февраль 2017 г.). «Мейофауна вторичноротых из базального кембрия Шэньси (Китай)». Природа . 542 (7640): 228–231. Бибкод : 2017Natur.542..228H . дои : 10.1038/nature21072 . ПМИД 28135722 . S2CID 353780 .
- ^ «Корнуолл – Природа – Червь-суперзвезда» . Би-би-си .
- ^ Марк Карвардин (1995) Книга рекордов животных Гиннеса . Издательство Гиннесс. п. 232.
- ^ «Постоянные паразиты» . Время . 8 апреля 1957 года. Архивировано из оригинала 27 июня 2008 года.
- ^ Харгис В., изд. (1985). Паразитология и патология морских организмов Мирового океана (Технический отчет). Национальное управление океанических и атмосферных исследований .
- ^ «Классификация паразитов животных» . Архивировано из оригинала 14 сентября 2006 года.
- ^ Гарсия Л.С. (октябрь 1999 г.). «Классификация паразитов, переносчиков и подобных организмов человека» . Клинические инфекционные болезни . 29 (4): 734–6. дои : 10.1086/520425 . ПМИД 10589879 .
- ^ Ходда М (2011). «Тип Nematoda Cobb, 1932. В: Чжан, З.-К. Биоразнообразие животных: обзор классификации более высокого уровня и обзор таксономического богатства». Зоотакса . 3148 : 63–95. дои : 10.11646/zootaxa.3148.1.11 .
- ^ Чжан Цзы (2013). «Биоразнообразие животных: обновление классификации и разнообразия в 2013 году. В: Чжан, З.-К. (ред.) Биоразнообразие животных: план классификации более высокого уровня и исследование таксономического богатства (Дополнения, 2013 г.)» . Зоотакса . 3703 (1): 5–11. дои : 10.11646/zootaxa.3703.1.3 . S2CID 85252974 .
- ^ Ламбсхед П.Дж. (1993). «Последние достижения в исследованиях биоразнообразия морского бентоса». Океанис . 19 (6): 5–24.
- ^ Боргони Г., Гарсиа-Мояно А., Литтауэр Д., Берт В., Бестер А., ван Херден Е. и др. (июнь 2011 г.). «Нематода из земных глубоких недр Южной Африки » Природа 474 (7349): 79–82. Бибкод : 2011Природа.474...79Б . дои : 10.1038/nature09974 . hdl : 1854/LU-1269676 . ПМИД 21637257 . S2CID 4399763 .
- ^ Дановаро Р., Гамби С., Делл'Анно А., Коринальдези С., Фраскетти С., Ванреузель А. и др. (январь 2008 г.). «Экспоненциальное снижение функционирования глубоководных экосистем, связанное с утратой донного биоразнообразия» . Современная биология . 18 (1): 1–8. дои : 10.1016/j.cub.2007.11.056 . ПМИД 18164201 . S2CID 15272791 .
- «Исчезновение глубоководных видов может привести к коллапсу океанов, предполагает исследование» . ЭврекАлерт! (Пресс-релиз). 27 декабря 2007 г.
- ^ Платт Х.М. (1994). «предисловие». В Лоренцен С., Лоренцен С.А. (ред.). Филогенетическая систематика свободноживущих нематод . Лондон: Общество Рэев. ISBN 978-0-903874-22-9 .
- ^ Барнс Р.С., Калоу П., Олив П.Дж. (2001). Беспозвоночные, синтез (3-е изд.). Великобритания: Blackwell Science.
- ^ «Тирийский пурпур» . Зеленый Лев . 28 февраля 2014 г. Архивировано из оригинала 28 февраля 2014 г.
- ^ Чепмен А.Д. (2009). Численность живых видов в Австралии и мире (2-е изд.). Канберра: Исследование биологических ресурсов Австралии. ISBN 978-0-642-56860-1 .
- ^ Хэнкок Р. (2008). «Признание исследований моллюсков» . Австралийский музей. Архивировано из оригинала 30 мая 2009 года . Проверено 9 марта 2009 г.
- ^ Подумайте В.Ф., Линдберг Д.Р., ред. (2008). Филогения и эволюция моллюсков . Беркли: Издательство Калифорнийского университета. п. 481. ИСБН 978-0-520-25092-5 .
- ^ Манро Д., Блиер П.У. (октябрь 2012 г.). «Чрезвычайное долголетие Arctica Islandica связано с повышенной устойчивостью митохондриальных мембран к перекисному окислению» . Стареющая клетка . 11 (5): 845–55. дои : 10.1111/j.1474-9726.2012.00847.x . ПМИД 22708840 . S2CID 205634828 .
- ^ «Добро пожаловать в CephBase» . CephBase . Архивировано из оригинала 12 января 2016 года . Проверено 29 января 2016 г.
- ^ Уилбур К.М., Кларк, М.Р., Труман, ER, ред. (1985), Моллюска , том. 12. Палеонтология и неонтология головоногих моллюсков, Нью-Йорк: Academic Press, ISBN. 0-12-728702-7
- ^ «Есть ли пресноводные головоногие моллюски?» . Австралийская радиовещательная корпорация . 16 января 2013 г.
- ^ Каллауэй Э (2 июня 2008 г.). «Простодушный Наутилус демонстрирует вспышку памяти» . Новый учёный . Проверено 7 марта 2012 г.
- ^ Филлипс К. (15 июня 2008 г.). «Живые ископаемые воспоминания». Журнал экспериментальной биологии . 211 (12): iii. дои : 10.1242/jeb.020370 . S2CID 84279320 .
- ^ Крук Р., Бэзил Дж (2008). «Двухфазная кривая памяти у наутилуса с камерами Nautilus pompilius L. (Cephalopoda: Nautiloidea)». Журнал экспериментальной биологии . 211 (12): 1992–1998. дои : 10.1242/jeb.018531 . ПМИД 18515730 . S2CID 6305526 .
- ^ Черный R (26 апреля 2008 г.). «Колоссальный кальмар из морозилки» . Новости Би-би-си .
- ^ Перейти обратно: а б Руперт Р.Э., Фокс Р.С., Барнс Р.Д. (2004). Зоология беспозвоночных (7-е изд.). Cengage Обучение. ISBN 978-81-315-0104-7 .
- ^ Хейворд П.Дж. (1996). Справочник морской фауны Северо-Западной Европы . Издательство Оксфордского университета. ISBN 978-0-19-854055-7 .
- ^ Уилсон Х.М., Андерсон Л.И. (январь 2004 г.). «Морфология и систематика палеозойских многоножек (Diplopoda: Chilognatha: Archipolypoda) из Шотландии» . Журнал палеонтологии . 78 (1): 169–184. doi : 10.1666/0022-3360(2004)078<0169:MATOPM>2.0.CO;2 . S2CID 131201588 .
- ^ Суарес С.Е., Брукфилд М.Э., Катлос Э.Дж., Штёкли Д.Ф. (2017). «U-Pb возраст циркона самого старого зарегистрированного наземного животного, дышащего воздухом» . ПЛОС ОДИН . 12 (6): e0179262. Бибкод : 2017PLoSO..1279262S . дои : 10.1371/journal.pone.0179262 . ПМЦ 5489152 . ПМИД 28658320 .
- ^ Кэмпбелл Л.И., Рота-Стабелли О., Эджкомб Г.Д., Маркиоро Т., Лонгхорн С.Дж., Телфорд М.Дж. и др. (сентябрь 2011 г.). «МикроРНК и филогеномика раскрывают взаимоотношения тихоходок и позволяют предположить, что бархатные черви являются сестринской группой членистоногих» . Труды Национальной академии наук Соединенных Штатов Америки . 108 (38): 15920–4. Бибкод : 2011PNAS..10815920C . дои : 10.1073/pnas.1105499108 . ПМК 3179045 . ПМИД 21896763 .
- ^ Смит Ф.В., Гольдштейн Б. (май 2017 г.). «Сегментация тихоходок и разнообразие сегментных моделей панартропод». Строение и развитие членистоногих . 46 (3): 328–340. дои : 10.1016/j.asd.2016.10.005 . ПМИД 27725256 .
- ^ Бадд Дж.Э. (2001). «Почему членистоногие сегментированы?». Эволюция и развитие . 3 (5): 332–42. дои : 10.1046/j.1525-142X.2001.01041.x . ПМИД 11710765 . S2CID 37935884 .
- ^ «Первая жизнь Дэвида Аттенборо» . Архивировано из оригинала 26 января 2011 года . Проверено 10 марта 2011 г.
- ^ Брэдди С.Дж., Пошманн М., Тетли О.Э. (февраль 2008 г.). «Гигантский коготь обнаружил самое большое членистоногое в истории» . Письма по биологии . 4 (1): 106–9. дои : 10.1098/rsbl.2007.0491 . ПМК 2412931 . ПМИД 18029297 .
- ^ Дэниел С. (21 ноября 2007 г.). «Обнаружен гигантский морской скорпион» . Природа . дои : 10.1038/news.2007.272 . Проверено 10 июня 2013 г.
- ^ Бикнелл, Рассел, округ Колумбия; Пейтс, Стивен (2020). «Иллюстрированный атлас ископаемых и современных мечехвостов с акцентом на Xiphosurida» . Границы в науках о Земле . 8 : 98. Бибкод : 2020FrEaS...8...98B . дои : 10.3389/feart.2020.00098 . ISSN 2296-6463 .
- ^ «Уродливый гигантский краб Японии» . Популярная наука . 96 (6): 42. 1920.
- ^ Карри Д.Р., Уорд ТМ (2009). Южно-Австралийский гигантский краб ( Pseudocarcinus gigas ) Промысел (PDF) . Южно-Австралийский институт исследований и разработок . Отчет об оценке рыболовства для PIRSA. Архивировано из оригинала (PDF) 28 марта 2012 года . Проверено 9 декабря 2013 г.
- ^ Килдей П. (28 сентября 2005 г.). «Креветка-богомол может похвастаться самыми развитыми глазами» . Ежедневный калифорнийец . Архивировано из оригинала 29 сентября 2012 года . Проверено 23 сентября 2016 г.
- ^ Патек С.Н., Колдуэлл Р.Л. (2005). «Чрезвычайные силы удара и кавитации биологического молота: ударные силы креветки-богомола-павлина» . Журнал экспериментальной биологии . 208 (19): 3655–3664. дои : 10.1242/jeb.01831 . ПМИД 16169943 . S2CID 312009 .
- ^ Хан, Цзянь; Моррис, Саймон Конвей; Оу, Цян; Шу, Деган; Хуан, Хай (2017). «Мейофауна вторичноротых из базального кембрия Шэньси (Китай)» . Природа . 542 (7640): 228–231. Бибкод : 2017Natur.542..228H . дои : 10.1038/nature21072 . ISSN 1476-4687 . ПМИД 28135722 . S2CID 353780 .
- ^ Лю, Юньхуань; Карлайл, Эмили; Чжан, Хуацяо; Ян, Бен; Штайнер, Майкл; Шао, Тецюань; Дуань, Байчуань; Мароне, Федерика; Сяо, Шухай; Донохью, Филип CJ (17 августа 2022 г.). «Saccorhytus — ранний экдисозой, а не самый ранний вторичноротый» . Природа . 609 (7927): 541–546. Бибкод : 2022Natur.609..541L . дои : 10.1038/s41586-022-05107-z . hdl : 1983/454e7bec-4cd4-4121-933e-abeab69e96c1 . ISSN 1476-4687 . ПМИД 35978194 . S2CID 251646316 .
- ^ «Сеть разнообразия животных - Иглокожие» . Зоологический музей Мичиганского университета . Проверено 26 августа 2012 г.
- ^ «Иглокожие | Определение, характеристики, виды и факты | Британника» . www.britanica.com . 8 июня 2023 г. Проверено 24 июня 2023 г.
- ^ Фокс Р. « Asterias forbesi » . Анатомия беспозвоночных онлайн . Ландерский университет . Проверено 14 июня 2014 г.
- ^ Холсингер, К. (2005). Краеугольные виды. Получено 10 мая 2010 г. с сайта Холсингер К. (11 октября 2005 г.). «Ключевые виды» . Университет Коннектикута . Архивировано из оригинала 30 июня 2010 года . Проверено 12 мая 2010 г.
- ^ Симаков О, Кавасима Т, Марлетаз Ф, Дженкинс Дж, Коянаги Р, Митрос Т и др. (ноябрь 2015 г.). «Гемихордовые геномы и происхождение вторичноротых» . Природа 527 (7579): 459–65. Бибкод : 2015Nature.527..459S . дои : 10.1038/nature16150 . ПМК 4729200 . ПМИД 26580012 .
- ^ «Как люди получили глотку от этого «уродливого зверя» » . Будущее . 23 ноября 2015 г.
- ^ Перейти обратно: а б с д Кларк М.А., Чой Дж., Дуглас М. (2018). «Хордовые» . Биология (2-е изд.). ОпенСтакс. ISBN 978-1-947172-95-1 . .
Измененный текст был скопирован из этого источника, который доступен по международной лицензии Creative Commons Attribution 4.0.
- ^ «Секрет жизни в Оезии: доисторические черви строили трубчатые «дома» на морском дне» . физ.орг .
- ^ Барнс Р.Д. (1982). Зоология беспозвоночных . Филадельфия, Пенсильвания: Холт-Сондерс Интернэшнл. стр. 1018–1026. ISBN 978-0-03-056747-6 .
- ^ «Вторичные организаторы раннего мозга и расположение мезодиэнцефальных дофаминергических клеток-предшественников» . Карта жизни . Архивировано из оригинала 10 марта 2014 года . Проверено 10 марта 2014 г.
- ^ Пани А.М., Малларки Э.Э., Аронович Дж., Асимакопулос С., Гроув Э.А., Лоу С.Дж. (март 2012 г.). «Древнее вторичноротое происхождение сигнальных центров мозга позвоночных» . Природа . 483 (7389). НаукаЖизнь: 289–94. Бибкод : 2012Natur.483..289P . дои : 10.1038/nature10838 . ПМЦ 3719855 . ПМИД 22422262 .
- ^ «Хордовые» . ОпенСтакс . 9 мая 2019 года.
- ^ Гевин В. (2005). «Функциональная геномика расширяет биологический сюжет» . ПЛОС Биология . 3 (6): е219. дои : 10.1371/journal.pbio.0030219 . ПМЦ 1149496 . ПМИД 15941356 .
- ^ Тиммер Дж (19 июня 2008 г.). «Геном ланцетника (амфиоксуса) и происхождение позвоночных» . Арс Техника .
- ^ Лемэр П. (2011). «Эволюционный перекресток биологии развития: оболочники». Разработка . 138 (11): 2143–2152. дои : 10.1242/dev.048975 . ПМИД 21558365 . S2CID 40452112 .
- ^ «FishBase: Глобальная информационная система по рыбам» . ФишБаза . Проверено 17 января 2017 г.
- ^ «Сколько рыбы в море? Перепись морской жизни представляет первый отчет» . Наука Дейли . Проверено 17 января 2017 г.
- ^ Docker MF (ноябрь 2006 г.). «Вклад Билла Бимиша в исследования миноги и последние достижения в этой области» . Обзоры гвельфской ихтиологии . 7 .
- ^ Хардисти М.В., Поттер И.С. (1971). Хардисти М.В., Поттер И.С. (ред.). Биология миног (1-е изд.). Академическая пресса. ISBN 978-0-123-24801-5 .
- ^ Гилл Х.С., Рено С.Б., Шапло Ф., Мэйден Р.Л., Поттер И.С. (2003). «Филогения живых паразитических миног (Petromyzontiformes) на основе морфологических данных». Копейя . 2003 (4): 687–703. дои : 10.1643/IA02-085.1 . S2CID 85969032 .
- ^ «Миксини» . Музей палеонтологии Калифорнийского университета. Архивировано из оригинала 15 декабря 2017 года . Проверено 17 января 2017 г.
- ^ Грин С.А., Броннер МЭ (2014). «Минога: модельная система бесчелюстных позвоночных для изучения происхождения нервного гребня и других черт позвоночных» . Дифференциация; Исследования биологического разнообразия . 87 (1–2): 44–51. дои : 10.1016/j.diff.2014.02.001 . ПМЦ 3995830 . ПМИД 24560767 .
- ^ Stock DW, Whitt GS (август 1992 г.). «Свидетельства на основе последовательностей рибосомальной РНК 18S о том, что миноги и миксины образуют естественную группу». Наука . 257 (5071): 787–9. Бибкод : 1992Sci...257..787S . дои : 10.1126/science.1496398 . ПМИД 1496398 .
- ^ Николлс Х. (сентябрь 2009 г.). «Эволюция: Из уст в уста» . Природа . 461 (7261): 164–6. дои : 10.1038/461164a . ПМИД 19741680 .
- ^ Маккой В.Е., Саупе Э.Э., Ламсделл Дж.К., Тархан Л.Г., МакМахон С., Лидгард С. и др. (апрель 2016 г.). «Монстр Талли» — позвоночное животное» . Природа . 532 (7600): 496–9. Бибкод : 2016Natur.532..496M . дои : 10.1038/nature16992 . ПМИД 26982721 . S2CID 205247805 .
- ^ Саллан Л., Джайлз С., Сэнсом Р.С., Кларк Дж.Т., Йохансон З., Сэнсом И.Дж. и др. (20 февраля 2017 г.). «Монстр Талли» не является позвоночным: характеры, конвергенция и тафономия у проблемных животных палеозоя» (PDF) . Палеонтология . 60 (2): 149–157. Бибкод : 2017Palgy..60..149S . дои : 10.1111/пала.12282 .
- ^ Геггель Л. (4 мая 2020 г.). «Древний «монстр Талли» был позвоночным, а не бесхарактерной каплей, утверждает исследование» . Живая наука .
- ^ Маккой В.Е., Виманн Дж., Ламсделл Дж.К., Уэлен К.Д., Лидгард С., Майер П. и др. (сентябрь 2020 г.). «Химические признаки мягких тканей различают позвоночных и беспозвоночных из каменноугольного лагеря Мазон-Крик в штате Иллинойс». Геобиология . 18 (5): 560–565. Бибкод : 2020Gbio...18..560M . дои : 10.1111/gbi.12397 . ПМИД 32347003 . S2CID 216646333 .
- ^ Миками, Томоюки; Икеда, Такафуми; Мурамия, Юсуке; Хирасава, Тацуя; Ивасаки, Ватару (2023). Чернс, Лесли (ред.). «Трехмерная анатомия монстра Талли ставит под сомнение его предполагаемое родство с позвоночными» . Палеонтология . 66 (2). дои : 10.1111/пала.12646 . ISSN 0031-0239 . S2CID 258198566 .
- ^ Киммел CB, Миллер CT, Кейнс Р.Дж. (2001). «Формирование нервного гребня и эволюция челюсти» . Журнал анатомии . 199 (Части 1–2): 105–20. дои : 10.1017/S0021878201008068 . ПМК 1594948 . ПМИД 11523812 .
- ^ Гай З, Чжу М (2012). «Происхождение челюсти позвоночных: пересечение модели, основанной на биологии развития, и ископаемых свидетельств» . Китайский научный бюллетень . 57 (30): 3819–3828. Бибкод : 2012ЧСБу..57.3819Г . дои : 10.1007/s11434-012-5372-z .
- ^ Мэйси Дж.Г. (2000). Обнаружение ископаемых рыб . Вествью Пресс. стр. 1–223. ISBN 978-0-8133-3807-1 .
- ^ Перейти обратно: а б Роу С., Хубер Д.Р., Лоури М., МакГенри С., Морено К., Клаузен П. и др. (2008). «Трёхмерный компьютерный анализ механики челюстей белой акулы: насколько сильно может укусить большая белая акула?» (PDF) . Журнал зоологии . 276 (4): 336–342. дои : 10.1111/j.1469-7998.2008.00494.x .
- ^ Пимьенто С., Эрет DJ, Макфадден Б.Дж., Хаббелл Дж. (май 2010 г.). Степанова А (ред.). «Древний питомник вымершей гигантской акулы мегалодона из миоцена Панамы» . ПЛОС ОДИН . 5 (5): е10552. Бибкод : 2010PLoSO...510552P . дои : 10.1371/journal.pone.0010552 . ПМК 2866656 . ПМИД 20479893 .
- ^ Ламберт О, Бьянуччи Дж, Пост К, де Мюзон К, Салас-Жисмонди Р, Урбина М, Роймер Дж (июль 2010 г.). «Гигантский укус нового хищного кашалота из миоценовой эпохи Перу». Природа . 466 (7302): 105–8. Бибкод : 2010Natur.466..105L . дои : 10.1038/nature09067 . ПМИД 20596020 . S2CID 4369352 .
- ^ Нильсен Дж., Хедехольм Р.Б., Хайнемайер Дж., Бушнелл П.Г., Кристиансен Дж.С., Олсен Дж. и др. (август 2016 г.). «Радиоуглеродный анализ хрусталика глаза показывает многовековое долголетие гренландской акулы (Somniosus microcephalus)». Наука . 353 (6300): 702–4. Бибкод : 2016Sci...353..702N . doi : 10.1126/science.aaf1703 . hdl : 2022/26597 . ПМИД 27516602 . S2CID 206647043 .
- Энрико де Лазаро (12 августа 2016 г.). «Гренландские акулы — самые долгоживущие позвоночные на Земле, говорят морские биологи» . Новости науки .
- ^ Маршалл А., Беннетт М.Б., Коджа Г., Инохоса-Альварес С., Гальван-Магана Ф., Хардинг М. и др. (2011). " Одеяло Бирострис Красный список исчезающих видов МСОП . 2011 : e.T198921A9108067. doi : 10.2305/IUCN.UK.2011-2.RLTS.T198921A9108067.en .
- ^ Черный R (11 июня 2007 г.). «Защита рыбы-пилы обретает зубы» . Новости Би-би-си .
- ^ Рядом с TJ, Эйтан Р.И., Дорнбург А., Кун К.Л., Мур Дж.А., Дэвис М.П. и др. (август 2012 г.). «Разрешение филогении лучепёрых рыб и сроки диверсификации» . Труды Национальной академии наук Соединенных Штатов Америки . 109 (34): 13698–703. Бибкод : 2012PNAS..10913698N . дои : 10.1073/pnas.1206625109 . ПМК 3427055 . ПМИД 22869754 .
- ^ Перейти обратно: а б Чжу М., Чжао В., Цзя Л., Лу Дж., Цяо Т., Цюй Цюй (март 2009 г.). «Самый старый сочлененный остихтиан демонстрирует мозаичные челюстно-костные признаки». Природа 458 (7237): 469–74. Бибкод : 2009Nature.458..469Z . дои : 10.1038/nature07855 . ПМИД 19325627 . S2CID 669711 .
- ^ Клак, JA (2002) Набирая силу . Университет Индианы
- ^ «Хондростеи: родственники осетровых» . палеос.com. Архивировано из оригинала 25 декабря 2010 года.
- ^ Лопес-Арбарелло А (2012). «Филогенетические взаимоотношения гинглимодовых рыб (Actinopterygii: Neopterygii)» . ПЛОС ОДИН . 7 (7): e39370. Бибкод : 2012PLoSO...739370L . дои : 10.1371/journal.pone.0039370 . ПМЦ 3394768 . ПМИД 22808031 .
- ^ Берра ТМ (2008). Распространение пресноводных рыб . Издательство Чикагского университета . п. 55. ИСБН 978-0-226-04443-9 .
- ^ Лакманн А.Р., Эндрюс А.Х., Батлер М.Г., Белак-Лакманн Э.С., Кларк М.Е. (23 мая 2019 г.). «Большеротый буйвол Ictiobus cyprinellus устанавливает рекорд пресноводной костистости, поскольку улучшенный возрастной анализ показывает столетнее долголетие» . Коммуникационная биология . 2 (1): 197. дои : 10.1038/s42003-019-0452-0 . ПМК 6533251 . ПМИД 31149641 .
- ^ Перейти обратно: а б Бентон М. (2005). «Эволюция рыб после девона» . Палеонтология позвоночных (3-е изд.). Джон Уайли и сыновья . стр. 175–84. ISBN 978-1-4051-4449-0 .
- ^ Боун Кью, Мур Р. (2008). Биология рыб . Гирляндная наука . п. 29. ISBN 978-0-415-37562-7 .
- ^ Дорит Р., Уокер В.Ф., Барнс Р.Д. (1991). Зоология . Издательство Колледжа Сондерса. стр. 67–69 . ISBN 978-0-03-030504-7 .
- ^ «Ученые описывают самую маленькую и легкую рыбу в мире» . Океанографический институт Скриппса . 20 июля 2004 г. Архивировано из оригинала 5 марта 2016 г. . Проверено 9 апреля 2016 г.
- ^ Роуч Дж. (13 мая 2003 г.). «Обнаружена самая тяжелая костистая рыба в мире?» . Национальные географические новости . Архивировано из оригинала 17 мая 2003 года . Проверено 9 января 2016 г.
- ^ «Сводная статистика видов, находящихся под угрозой исчезновения во всем мире» (PDF) . Красный список исчезающих видов МСОП . Всемирный союз охраны природы. Осень 2014 г.
Таблица 1: Численность находящихся под угрозой исчезновения видов по основным группам организмов (1996–2014 гг.)
- ^ Наркевич К., Наркевич М. (январь 2015 г.). «Возраст старейших следов четвероногих из Зачелме, Польша». Летайя . 48 (1): 10–12. дои : 10.1111/лет.12083 . ISSN 0024-1164 .
- ^ Лонг Дж.А., Гордон М.С. (сентябрь – октябрь 2004 г.). «Величайший шаг в истории позвоночных: палеобиологический обзор перехода от рыб к четвероногим» (PDF) . Физиологическая и биохимическая зоология . 77 (5): 700–19. дои : 10.1086/425183 . ПМИД 15547790 . S2CID 1260442 .
- ^ Шубин Н. (2008). Ваша внутренняя рыба: путешествие в 3,5-миллиардную историю человеческого тела . Нью-Йорк: Книги Пантеона. ISBN 978-0-375-42447-2 .
- ^ Лаурин М (2010). Как позвоночные покинули воду . Беркли, Калифорния, США: Издательство Калифорнийского университета. ISBN 978-0-520-26647-6 .
- ^ Кановиль А., Лорин М. (2010). «Эволюция микроанатомии плечевой кости и образа жизни амниот и некоторые комментарии к палеобиологическим выводам» . Биологический журнал Линнеевского общества . 100 (2): 384–406. дои : 10.1111/j.1095-8312.2010.01431.x .
- ^ Лорин М. , Кановиль А., Кильяк А. (август 2009 г.). «Использование палеонтологических и молекулярных данных супердеревьев для сравнительных исследований: на примере микроанатомии бедренной кости лисамфибий» . Журнал анатомии . 215 (2): 110–23. дои : 10.1111/j.1469-7580.2009.01104.x . ПМК 2740958 . ПМИД 19508493 .
- ^ Хопкинс Г.Р., Эд Броди-младший (2015). «Распространение земноводных в солончаковых средах обитания: обзор и эволюционная перспектива». Герпетологические монографии . 29 (1): 1–27. doi : 10.1655/HERPMONOGRAPHHS-D-14-00006 . S2CID 83659304 .
- ^ Натчев Н., Цанков Н., Гем Р. (2011). «Инвазия зеленых лягушек в Черное море: экология местообитаний популяции Pelophylax esculentus complex (Anura, Amphibia) в районе лагуны Шабленска Тузла в Болгарии» (PDF) . Герпетологические заметки . 4 : 347–351. Архивировано из оригинала (PDF) 24 сентября 2015 года . Проверено 11 августа 2016 г.
- ^ Сандер П.М. (август 2012 г.). «Палеонтология. Размножение ранних амниот». Наука . 337 (6096): 806–8. Бибкод : 2012Sci...337..806S . дои : 10.1126/science.1224301 . ПМИД 22904001 . S2CID 7041966 .
- ^ Модесто С.П., Андерсон Дж.С. (октябрь 2004 г.). «Филогенетическое определение рептилий» . Систематическая биология . 53 (5): 815–21. дои : 10.1080/10635150490503026 . ПМИД 15545258 .
- ^ Готье Дж.А., Клюге А.Г., Роу Т. (1988). «Ранняя эволюция амниоты». В Бентоне М.Дж. (ред.). Филогения и классификация четвероногих . Том. 1. Оксфорд: Кларендон Пресс. стр. 103–155. ISBN 978-0-19-857705-8 .
- ^ Лаурин М., Рейш Р.Р. (1995). «Переоценка ранней филогении амниот» (PDF) . Зоологический журнал Линнеевского общества . 113 (2): 165–223. дои : 10.1111/j.1096-3642.1995.tb00932.x . Архивировано из оригинала (PDF) 8 июня 2019 года . Проверено 14 августа 2016 г.
- ^ Модесто СП (1999). «Наблюдения за строением раннепермской рептилии Stereosternum tumidum Cope». Палеонтология Африканская . 35 : 7–19.
- ^ Расмуссен А.Р., Мерфи Дж.К., Омпи М., Гиббонс Дж.В., Уец П. (8 ноября 2011 г.). «Морские рептилии» . ПЛОС ОДИН . 6 (11): e27373. Бибкод : 2011PLoSO...627373R . дои : 10.1371/journal.pone.0027373 . ПМК 3210815 . ПМИД 22087300 .
- ^ Стидворти Дж (1974). Змеи мира . Grosset & Dunlap Inc. с. 160. ИСБН 978-0-448-11856-7 .
- ^ «Морские змеи» (PDF) . Продовольственная и сельскохозяйственная организация Объединенных Наций . Проверено 22 августа 2020 г.
- ^ Расмуссен А.Р., Мерфи Дж.К., Омпи М., Гиббонс Дж.В., Уетц П. (2011). «Морские рептилии» . ПЛОС ОДИН . 6 (11): e27373. Бибкод : 2011PLoSO...627373R . дои : 10.1371/journal.pone.0027373 . ПМК 3210815 . ПМИД 22087300 .
- ^ Мартилл Д.М. (1993). «Суповые субстраты: среда для исключительного сохранения ихтиозавров из сланцев Посидония (нижняя юра) в Германии». Каупиа – Дармштадтский вклад в естествознание , 2 : 77–97.
- ^ Гулд С.Дж. (1993). «Изогнутый из формы» . Восемь поросят: размышления о естествознании . Нортон. стр. 179–94. ISBN 978-0-393-31139-6 .
- ^ «Феномен безумного кормления акул сардинами в Африке» . Архивировано из оригинала 2 декабря 2008 года.
- ^ «Список видов и подвидов Комитета по таксономии Общества морских млекопитающих» . Общество морской маммологии. Октябрь 2015. Архивировано из оригинала 6 января 2015 года . Проверено 23 ноября 2015 г.
- ^ Ромер А.С., Парсонс Т.С. (1986). Тело позвоночного . Издательство колледжа Сандерса. п. 96. ИСБН 978-0-03-058446-6 .
- ^ «Синий кит» . Всемирный фонд природы . Проверено 15 августа 2016 г.
- ^ Марино Л. (2004). «Эволюция мозга китообразных: умножение порождает сложность» (PDF) . Международное общество сравнительной психологии (17): 1–16. Архивировано из оригинала (PDF) 16 сентября 2018 года . Проверено 15 августа 2016 г.
- ^ Перейти обратно: а б Кэмпбелл Н.А., Рис Дж.Б., Урри Л.А., Кейн М.Л., Вассерман С.А., Минорский П.В., Джексон Р.Б. (2008). Биология (8-е изд.). Сан-Франциско: Пирсон – Бенджамин Каммингс. ISBN 978-0-321-54325-7 .
- ^ Барри Ф.Р., Бак В.Р., Демулен В., Гройтер В., Хоксворт Д.Л., Херендин П.С. и др., ред. (2012). Международный кодекс номенклатуры водорослей, грибов и растений (Мельбурнский кодекс), принятый Восемнадцатым Международным ботаническим конгрессом в Мельбурне, Австралия, июль 2011 г. (электронное издание). Международная ассоциация систематики растений . Проверено 14 мая 2017 г.
- ^ Уолш П.Дж., Смит С., Флеминг Л., Соло-Габриэль Х., Гервик У.Х., ред. (2 сентября 2011 г.). «Цианобактерии и цианобактериальные токсины» . Океаны и здоровье человека: морские риски и средства правовой защиты . Академическая пресса. стр. 271–296. ISBN 978-0-08-087782-2 .
- ^ «Повышение уровня кислорода — журнал астробиологии» . Журнал астробиологии . 30 июля 2003 г. Проверено 6 апреля 2016 г.
- ^ Фланнери Д.Т., Уолтер Р.М. (2012). «Архейские тафтинговые микробные маты и Великое событие окисления: новый взгляд на древнюю проблему». Австралийский журнал наук о Земле . 59 (1): 1–11. Бибкод : 2012AuJES..59....1F . дои : 10.1080/08120099.2011.607849 . S2CID 53618061 .
- ^ Ротшильд Л. (сентябрь 2003 г.). «Понять эволюционные механизмы и экологические пределы жизни» . НАСА. Архивировано из оригинала 29 марта 2012 года . Проверено 13 июля 2009 г.
- ^ Надис С. (декабрь 2003 г.). «Клетки, управляющие морями» (PDF) . Научный американец . 289 (6): 52–3. Бибкод : 2003SciAm.289f..52N . doi : 10.1038/scientificamerican1203-52 . ПМИД 14631732 . Архивировано из оригинала (PDF) 19 апреля 2014 года . Проверено 2 июня 2019 г.
- ^ «Самый важный микроб, о котором вы никогда не слышали» . npr.org .
- ^ Фломбаум П., Гальегос Дж.Л., Гордилло Р.А., Корнер Дж., Шафран Л.Л., Цзяо Н. и др. (июнь 2013 г.). «Настоящее и будущее глобальное распространение морских цианобактерий Prochromococcus и Synechococcus» . Труды Национальной академии наук Соединенных Штатов Америки . 110 (24): 9824–9. Бибкод : 2013PNAS..110.9824F . дои : 10.1073/pnas.1307701110 . ПМЦ 3683724 . ПМИД 23703908 .
- ^ Нэборс МВ (2004). Введение в ботанику . Сан-Франциско, Калифорния: ISBN Pearson Education, Inc. 978-0-8053-4416-5 .
- ^ Аллаби М., изд. (1992). «Водоросли». Краткий словарь ботаники . Оксфорд: Издательство Оксфордского университета.
- ^ Гири, доктор медицинских наук (октябрь 2012 г.). «Сколько существует видов водорослей?». Журнал психологии . 48 (5): 1057–63. дои : 10.1111/j.1529-8817.2012.01222.x . ПМИД 27011267 . S2CID 30911529 .
- ^ Перейти обратно: а б Гири, доктор медицинских наук, Гири, генеральный директор (2016). «Альгебаза» . www.algaebase.org . Проверено 20 ноября 2016 г. .
- ^ Томас Д. (2002). Морские водоросли . Серия «Жизнь». Музей естественной истории , Лондон. ISBN 978-0-565-09175-0 .
- ^ Хук С., Манн Д., Янс Х.М., Янс М. (1995). Водоросли: введение в психологию . Издательство Кембриджского университета. п. 166. ИСБН 9780521316873 . OCLC 443576944 .
- ^ Трегер П., Нельсон Д.М., Ван Беннеком А.Дж., Демастер DJ, Лейнаерт А., Кегинер Б. (апрель 1995 г.). «Баланс кремнезема в мировом океане: переоценка». Наука . 268 (5209): 375–9. Бибкод : 1995Sci...268..375T . дои : 10.1126/science.268.5209.375 . ПМИД 17746543 . S2CID 5672525 .
- ^ «Королевский колледж Лондона — озеро Мегачад» . www.kcl.ac.uk. Проверено 5 мая 2018 г.
- ^ Гомес Ф (2012). «Контрольный список и классификация живых динофлагеллят (Dinoflagellata, Alveolata)» . СИКИМАР Океаниды . 27 (1): 65–140. doi : 10.37543/oceanides.v27i1.111 .
- ^ Стокер Д.К. (1999). «Миксотрофия среди динофлагеллят». Журнал эукариотической микробиологии . 46 (4): 397–401. дои : 10.1111/j.1550-7408.1999.tb04619.x . S2CID 83885629 .
- ^ Старкс С. (31 октября 2012 г.). «Место под солнцем – водоросли – это урожай будущего, по мнению исследователей из Geel] Flanders Today» . Архивировано из оригинала 4 марта 2016 года . Проверено 8 декабря 2012 г.
- ^ Дюваль Б., Маргулис Л. (1995). «Микробное сообщество разносторонних колоний Ophrydium: эндосимбионты, резиденты и арендаторы». Симбиоз . 18 : 181–210. ПМИД 11539474 .
- ^ Вернберг Т., Крумхансл К., Филби-Декстер К. и Педерсен М.Ф. (2019) «Состояние и тенденции мировых ламинариевых лесов». В: Мировые моря: экологическая оценка , стр. 57–78). Академическая пресса. два : 10.1016/B978-0-12-805052-1.00003-6 .
- ^ Манн К.Х. (декабрь 1973 г.). «Морские водоросли: их продуктивность и стратегия роста: роль крупных морских водорослей в продуктивности прибрежных районов гораздо важнее, чем предполагалось». Наука . 182 (4116): 975–81. Бибкод : 1973Sci...182..975M . дои : 10.1126/science.182.4116.975 . ПМИД 17833778 . S2CID 26764207 .
- ^ Таннелл Дж.В., Чавес Э.А., Уизерс К. (2007). Коралловые рифы южной части Мексиканского залива . Издательство Техасского университета A&M. п. 91. ИСБН 978-1-58544-617-9 .
- ^ « Каулерпа таксифолия (водоросли-убийцы)» . Справочник инвазивных видов . Международный центр сельского хозяйства и биологических наук. 6 ноября 2018 г.
- ^ Мандоли Д.Ф. (июнь 1998 г.). «Разработка плана тела и фазовый переход во время развития вертлужной впадины: как строится сложная архитектура гигантской одноклеточной клетки?». Ежегодный обзор физиологии растений и молекулярной биологии растений . 49 : 173–198. дои : 10.1146/annurev.arplant.49.1.173 . ПМИД 15012232 . S2CID 6241264 .
- ^ Мадл П., Йип М. (2004). «Обзор литературы Caulerpa Taxifolia» . БУФУС-Инфо . 19 (31). Архивировано из оригинала 8 октября 2022 года . Проверено 18 июля 2017 г.
- ^ Орт Р.Дж., Каррутерс Т.Дж., Деннисон В.К., Дуарте К.М., Фуркурин Дж.В., Хек К.Л. и др. (2006). «Глобальный кризис экосистем морских водорослей» . Бионаука . 56 (12): 987–996. doi : 10.1641/0006-3568(2006)56[987:AGCFSE]2.0.CO;2 . hdl : 10261/88476 . S2CID 4936412 .
- ^ Фрёзе, Райнер; Поли, Дэниел (ред.) (2009). « Phycodurus eques » в FishBase . Версия за июль 2009 года.
- ^ Гири С., Очиенг Э., Тисзен Л.Л., Чжу З., Сингх А., Лавленд Т. и др. (2011). «Состояние и распространение мангровых лесов мира по данным спутникового наблюдения Земли». Глобальная экология и биогеография . 20 (1): 154–159. дои : 10.1111/j.1466-8238.2010.00584.x .
- ^ Томас Н., Лукас Р., Бантинг П., Харди А., Розенквист А., Симард М. (2017). «Распространение и движущие силы глобального изменения мангровых лесов, 1996–2010 годы» . ПЛОС ОДИН . 12 (6): e0179302. Бибкод : 2017PLoSO..1279302T . дои : 10.1371/journal.pone.0179302 . ПМЦ 5464653 . ПМИД 28594908 .
- ^ Короткое FT, Фредерик Т. (2003). Мировой атлас морских трав . Беркли, Калифорния: Издательство Калифорнийского университета. п. 24. ISBN 978-0-520-24047-6 . Архивировано из оригинала 10 июля 2019 года . Проверено 10 июля 2019 г.
- ^ Спалдинг М (2010). Мировой атлас мангровых зарослей . Рутледж. дои : 10.4324/9781849776608 . ISBN 978-1-84977-660-8 .
- ^ Лалли С., Парсонс Т. (1993). Биологическая океанография: Введение . Баттерворт-Хайнеманн. ISBN 0-7506-3384-0 .
- ^ Линдси Р., Скотт М., Симмон Р. (2010). «Что такое фитопланктон» . Земная обсерватория НАСА .
- ^ Филд CB, Беренфельд М.Дж., Рандерсон Дж.Т., Фальковски П. (июль 1998 г.). «Первичная продукция биосферы: интеграция наземных и океанических компонентов» . Наука . 281 (5374): 237–40. Бибкод : 1998Sci...281..237F . дои : 10.1126/science.281.5374.237 . ПМИД 9657713 .
- ^ Рост Б, Рибеселл Ю (2004). «Кокколитофоры и биологический насос: реакция на изменения окружающей среды». Кокколитофоры: от молекулярных процессов к глобальному воздействию . Спрингер. стр. 99–125. ISBN 978-3-662-06278-4 .
- ^ Арсеньев Л., Симон Н., Риго-Жалабер Ф., Ле Галль Ф., Шаффрон С., Корр Е., Ком Е., Бигерд Э., Боду АС (2018). «Первые вирусы, заражающие морскую диатомовую водоросль Guinardia delicatula» . Границы микробиологии . 9 : 3235. дои : 10.3389/fmicb.2018.03235 . ПМК 6334475 . ПМИД 30687251 .
- ^ Вареа С., Арагон Х.Л., Баррио Р.А. (октябрь 1999 г.). «Узоры Тьюринга на сфере». Физический обзор E . 60 (4 части Б): 4588–92. Бибкод : 1999PhRvE..60.4588V . дои : 10.1103/PhysRevE.60.4588 . ПМИД 11970318 .
- ^ Харви Э.Н. (1952). Биолюминесценция . Академическая пресса.
- ^ «Предлагаемое объяснение светящихся морей, включая светящиеся в настоящее время калифорнийские моря» . Национальный научный фонд . 18 октября 2011 г.
- ^ Кастро П., Хубер М.Е. (2010). Морская биология (8-е изд.). МакГроу Хилл. стр. 95 . ISBN 978-0071113021 .
- ^ Гастингс Дж.В. (1996). «Химия и цвета биолюминесцентных реакций: обзор». Джин . 173 (1 номер спецификации): 5–11. дои : 10.1016/0378-1119(95)00676-1 . ПМИД 8707056 .
- ^ Хэддок С.Х., Молин М.А., Кейс Дж.Ф. (2009). «Биолюминесценция в море». Ежегодный обзор морской науки . 2 : 443–93. Бибкод : 2010ARMS....2..443H . doi : 10.1146/annurev-marine-120308-081028 . ПМИД 21141672 . S2CID 3872860 .
- ^ «Круговорот углерода и биосеквестрация» . Министерство энергетики США, Управление науки Министерства энергетики. 2008. с. 81.
Отчет семинара DOE/SC-108.
- ^ Кэмпбелл М. (22 июня 2011 г.). «Роль морского планктона в секвестрации углерода» . ЗемляТаймс . Проверено 22 августа 2014 г.
- ^ Роман Дж., Маккарти Джей-Джей (октябрь 2010 г.). «Китовый насос: морские млекопитающие повышают первичную продуктивность прибрежного бассейна» . ПЛОС ОДИН . 5 (10): е13255. Бибкод : 2010PLoSO...513255R . дои : 10.1371/journal.pone.0013255 . ПМЦ 2952594 . ПМИД 20949007 . е13255.
- ^ Браун Дж. Э. (12 октября 2010 г.). «Китовый экскремент улучшает здоровье океана» . Наука Дейли . Проверено 18 августа 2014 г.
- ^ «Вода – универсальный растворитель» . Геологическая служба США . Архивировано из оригинала 9 июля 2017 года . Проверено 27 июня 2017 г.
- ^ Брам-младший, Моррис Джей-Джей, Десима М., Стукель М.Р. (2014). Глава 2: Смертность в океанах: причины и последствия . Материалы симпозиума Eco-DAS IX. Ассоциация наук лимнологии и океанографии. стр. 16–48. ISBN 978-0-9845591-3-8 . .
- ^ Рис Дж. Б. (2013). Кэмпбелл Биология (10-е изд.). Пирсон . ISBN 978-0-321-77565-8 .
- ^ Прентис IC (2001). «Круговорот углерода и углекислый газ в атмосфере» . Изменение климата 2001: научная основа: вклад Рабочей группы I в Третий оценочный доклад Межправительственной группы экспертов по изменению климата / Хоутон, Дж. Т. [ред.] Проверено 31 мая 2012 г. .
- ^ Томсон CW (2014). Путешествие «Челленджера: Атлантика» . Издательство Кембриджского университета. п. 235. ИСБН 978-1-108-07475-9 .
- ^ Хасле Г.Р., Сиверцен Э.Э., Стейдингер, Танген К. (25 января 1996 г.). «Морские диатомеи» . В Томасе ЧР (ред.). Определение морских диатомей и динофлагеллят . Академическая пресса. стр. 5–385. ISBN 978-0-08-053441-1 . Проверено 13 ноября 2013 г.
- ^ Адл С.М., Леандер Б.С., Симпсон А.Г., Арчибальд Дж.М., Андерсон О.Р., Басс Д. и др. (август 2007 г.). «Разнообразие, номенклатура и систематика протистов» . Систематическая биология . 56 (4): 684–9. дои : 10.1080/10635150701494127 . ПМИД 17661235 .
- ^ Мохеймани Н.Р., Уэбб Дж.П., Боровицка М.А. (октябрь 2012 г.). «Биоремедиация и другие потенциальные применения кокколитофоридных водорослей: обзор». Водорослевые исследования . 1 (2): 120–33. дои : 10.1016/j.algal.2012.06.002 .
- ^ Тейлор А.Р., Кракри А., Уиллер Г., Годдард Х., Браунли С. (июнь 2011 г.). «Потенциал-зависимый канал H+, лежащий в основе гомеостаза pH в кальцинирующих кокколитофорах» . ПЛОС Биология . 9 (6): e1001085. дои : 10.1371/journal.pbio.1001085 . ПМК 3119654 . ПМИД 21713028 .
- ^ Халперн Б.С., Фрейзер М., Аффлербах Дж., Лаундс Дж.С., Мишели Ф., О'Хара С. и др. (август 2019 г.). «Недавние темпы изменения воздействия человека на мировой океан» . Научные отчеты . 9 (1): 11609. Бибкод : 2019NatSR...911609H . дои : 10.1038/s41598-019-47201-9 . ПМК 6691109 . ПМИД 31406130 .
- ^ «Воздействие человека на морские экосистемы» . ГЕОМАР Центр океанических исследований имени Гельмгольца . Архивировано из оригинала 31 октября 2020 года . Проверено 22 октября 2019 г.
- ^ Розинг М.Т., Бёрд Д.К., Sleep NH, Бьеррум С.Дж. (апрель 2010 г.). «Никакого климатического парадокса под слабым ранним Солнцем». Природа . 464 (7289): 744–7. Бибкод : 2010Natur.464..744R . дои : 10.1038/nature08955 . ПМИД 20360739 . S2CID 205220182 .
- ^ Перейти обратно: а б Сахни С., Бентон М.Дж., Ферри, Пенсильвания (август 2010 г.). «Связи между глобальным таксономическим разнообразием, экологическим разнообразием и распространением позвоночных на суше» . Письма по биологии . 6 (4): 544–7. дои : 10.1098/rsbl.2009.1024 . ПМК 2936204 . ПМИД 20106856 .
- ^ МакКинни М.Л. (1997). «Как редким видам удается избежать вымирания? Палеонтологический взгляд» . Биология редкости . стр. 110–29. дои : 10.1007/978-94-011-5874-9_7 . ISBN 978-94-010-6483-5 .
- ^ Стернс БП, Стернс СК (1999). Смотрю с края вымирания . Нью-Хейвен, Коннектикут: Издательство Йельского университета. п. х. ISBN 978-0-300-08469-6 .
- ^ Новачек М.Ю. (8 ноября 2014 г.). «Блестящее будущее предыстории» . Нью-Йорк Таймс . Нью-Йорк. ISSN 0362-4331 . Проверено 25 декабря 2014 г.
- ^ Ни С. (август 2004 г.). «Вымирание, слизь и низы» . ПЛОС Биология . 2 (8): Е272. дои : 10.1371/journal.pbio.0020272 . ПМК 509315 . ПМИД 15314670 .
- ^ Уорд ПД (октябрь 2006 г.). «Удар из глубины». Научный американец . 295 (4): 64–71. Бибкод : 2006SciAm.295d..64W . doi : 10.1038/scientificamerican1006-64 (неактивен 26 апреля 2024 г.). ПМИД 16989482 .
{{cite journal}}
: CS1 maint: DOI неактивен по состоянию на апрель 2024 г. ( ссылка ) - ^ Морские вымирания: закономерности и процессы - обзор. 2013. Монография CIESM 45 [1]
- ^ Перейти обратно: а б с «Исследование морской жизни | Перепись морской жизни» . www.coml.org . Проверено 27 декабря 2023 г.
- ^ «Инструменты исследования: АНПА: Управление по исследованию и исследованию океана НОАА» . Oceanexplorer.noaa.gov . Проверено 27 декабря 2023 г.
- ^ «Буксируемые глубоководные аппараты (DTV) | Перепись морской жизни» . www.coml.org . Проверено 27 декабря 2023 г.
- ^ «Революция дронов под водой» . Расширенная навигация . Проверено 27 декабря 2023 г.
Дальнейшее чтение
[ редактировать ]
- Халперн Б.С., Уолбридж С., Селкое К.А., Каппель К.В., Микели Ф., Д'Агроса С. и др. (февраль 2008 г.). «Глобальная карта воздействия человека на морские экосистемы». Наука . 319 (5865): 948–52. Бибкод : 2008Sci...319..948H . дои : 10.1126/science.1149345 . ПМИД 18276889 . S2CID 26206024 .
- Палечный М., Хэммилл Э., Карпузи В., Поли Д. (2015). «Тенденция численности наблюдаемых морских птиц в мире, 1950-2010 гг.» . ПЛОС ОДИН . 10 (6): e0129342. Бибкод : 2015PLoSO..1029342P . дои : 10.1371/journal.pone.0129342 . ПМЦ 4461279 . ПМИД 26058068 .
- Руперт Э.Э., Фокс Р.С., Барнс Р.Д. (2004). Зоология беспозвоночных (7-е изд.). Брукс / Коул. ISBN 978-0-03-025982-1 .
- «После 60 миллионов лет экстремального образа жизни морские птицы терпят крушение» . Хранитель . 22 сентября 2015 г.